Abstract
Plant-derived natural compounds are desired for their anti-inflammatory properties. We investigated the chemical composition and the anti-inflammatory effects of the essential oil obtained from leaves of the Chinese medicinal plant Artemisia annua L. Artemisia annua L. essential oil (AO) was obtained by hydrodistillation; the extraction yield was approximately 1.2%. Gas chromatography–mass spectrometry (GC–MS) revealed the presence of 13 compounds in AO; the major compounds were caryophyllene (32.16%), eucalyptol (28.62%), α-pinene (19.08%), and β-pinene (14.72%). Cell counting kit-8 (CCK8) assay revealed that AO did not show in vitro cytotoxicity toward RAW264.7 cells and suppressed the expression of nitric oxide (NO), tumor necrosis factor-alpha (TNF-α), and interleukin-6 (IL-6) in lipopolysaccharide (LPS)-induced RAW264.7 cells. Pretreatment with AO significantly reduced xylene-induced inflammation in the ears of Kunming mice. AO inhibited inflammatory cell infiltration, decreased epidermis thickness in the ear, and suppressed expression of TNF-α in the serum. The in vivo anti-inflammatory activity of AO was similar to that of the positive control compound Di Song Zhang Bo Cream. Overall, AO was observed to be an effective anti-inflammatory agent. These results provide an experimental basis for commercializing AO-derived external preparations for pharmacological and cosmetic industries.
GRAPHICAL ABSTRACT
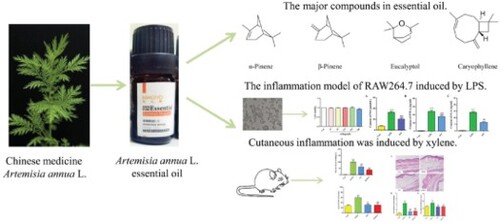
Introduction
Essential oils are complex mixtures of natural substances with aromatic odors, usually extracted from aromatic plants, which are present as secondary metabolites. Traditionally, essential oils have been widely used in food preparation and preservation, perfumery, and flavoring agents. In previous studies, the phytochemical composition and the biological activities of essential oils have been analyzed. Essential oils contain numerous bioactive compounds that exhibit a wide range of biological activities, including antibacterial, antioxidant, anti-inflammatory, antiviral, antitumor, analgesic, antiparasitic, antidiarrheal, and anxiolytic activities (Borges et al. Citation2019; Tarumi and Shinohara Citation2020; Muller et al. Citation2021; Van et al. Citation2021; Yatoo et al. Citation2021; Zi et al. Citation2021). Essential oils are hydrophobic and lipophilic and have a low molecular weight, which allows them to be easily absorbed through the skin. Chinese medicine essential oil widely exists in aromatic Chinese medicine, with various biological and pharmacological activities (Pan et al. Citation2013; Chu et al. Citation2022). Recently, Chinese medicinal essential oils have attracted considerable market attention, and the demand for these oils has increased.
Artemisia annua L. essential oil (AO) is a volatile, pleasant aroma extracted from Artemisia annua L. (A. annua). Artemisia annua L. is used in traditional Chinese medicine and was first mentioned in ‘Shen Nong Ben Cao Jing.’ It belongs to the family Compositae that is widely distributed throughout China. It has been used since ancient China to clear deficiency heat, treat malaria, and remove jaundice (Liu Citation2017). AO is a Chinese medicinal essential oil that shows various biological and pharmacological activities. Recent studies have shown that AO has antibacterial, anti-inflammatory, antiviral, mosquito-repellent, insecticidal, and antioxidant activities (Abad et al. Citation2012; Bilia et al. Citation2014; Kim et al. Citation2015; Zhao et al. Citation2016; Taleghani et al. Citation2020; Zhang et al. Citation2020; Fu et al. Citation2022). Previous studies have reported the application of AO via oral and nasal administration and intravenous injection, but little information is available regarding topical administration. AO has dual properties of an essential oil and a drug; however, it is not known whether AO would show the same effect upon administration through the epidermis. Research on the application of AO to the skin could significantly influence the development of external preparations by the pharmacological and cosmetic industries.
Inflammation is the primary component of a complex reaction triggered by tissue injury, stress, or infection and helps to restore tissue homeostasis. The inflammatory response is characterized by redness, edema, increased body temperature, pain, and decreased tissue function caused by various vascular and cellular events (Chen et al. Citation2018). Inflammatory responses induce activation of immune cells such as macrophages, which are the most important inflammatory cells and play a critical role in the inflammatory process by secreting a large number of pro-inflammatory mediators and cytokines, such as nitric oxide (NO), tumor necrosis factor-alpha (TNF-α), prostaglandin E2 (PGE2), interleukin-1 (IL-1), IL-6, IL-8 and so on (Oh et al. Citation2015). So inhibiting their release has become an essential means of treating inflammation.
Anti-inflammatory drugs, including non-steroidal anti-inflammatory drugs, are associated with adverse effects. Moreover, anti-inflammatory drug abuse leads to an increase in resistance to drugs (Gias et al. Citation2020). Plant-derived natural compounds have been examined in various studies for their anti-inflammatory properties.
Acute inflammation induced in a xylene-induced ear swelling model is frequently used to validate the evaluation of anti-inflammatory effect of drugs in vivo (Bitencourt et al. Citation2011; Oh et al. Citation2015; Zhang et al. Citation2018). LPS stimulated RAW264.7 is a well-established in vitro inflammation model (Chen et al. Citation2014; Feng et al. Citation2020). In this study, xylene-induced ear swelling model and LPS stimulated RAW264.7 model were used to verify the anti-inflammatory effects of AO by using a transdermal drug delivery system. We hope to provide experimental evidence for the potential application of AO as a raw material for synthesizing cosmetic products and external pharmacological preparations.
Materials and methods
Plant materials and chemicals
Fresh leaves of Artemisia annua L. were collected in May from Henan Province, China. Lipopolysaccharide (LPS; CAS no.: L2630) was purchased from Sigma-Aldrich (USA), Dulbecco’s Modified Eagle Medium (DMEM) and FBS were purchased from Gibco (USA), cell counting kit-8 (CCK8) was purchased from Beyotime (Shanghai, China), and Di Song Zhang Bo Cream (DSZBC, CAS no.: 21060501) was obtained from the Shanghai Skin Disease Hospital (Shanghai, China). Kits for analysis of NO, TNF-α, and IL-6 were purchased from Nanjing Jiancheng Bioengineering Institute (Nanjing, China). Xylene was purchased from Sinopharm Chemical Reagent Co., Ltd. (Shanghai, China).
AO extraction from Artemisia annua L
The freshly collected leaves of A. annua were washed, dried, sliced, and mechanically crushed. The powder was soaked in distilled water for 1.5 h. AO was extracted by performing hydrodistillation for approximately 75 min using a Clevenger-type apparatus. The distilled essential oil was dried over anhydrous sodium sulfate, filtered, and stored in an airtight bottle at 4 ˆC until use.
Analysis of AO
Gas chromatography–mass spectrometry (GC–MS)-based analysis of AO was performed using an Agilent 7000B GC–MS instrument (Agilent Technologies, USA). First, 1 μl of AO was dissolved in 1 ml of diethyl ether, filtered through a 0.22 μm-rated filter membrane, and then used to identify the components. The components were separated by fused and bonded HP-5 ms column (30 m × 0.25 mm; 0.25 μm film thickness; Agilent). The analytical conditions were: injection volume 1 μl with split ratio 1:10, helium (99.9995% purity) used as the carrier gas at a flow rate of 1 ml/min, and injector inlet temperature 250 ˆC. The initial temperature of the oven was 60 ˆC, which was ramped at 5 ˆC/min to a final temperature of 300 ˆC; the final temperature was maintained for 20 min. The MS scan parameters included an electron impact ionization voltage of 70 eV and a mass range of 30–400 m/e. The ion source and the transfer line temperatures were 250 and 230 ˆC, respectively. Component identification was performed by comparing the mass spectra to the mass spectrometry database (Wiley Library 2020 v 2.4) and the retention indices to the retention index database (NIST Chemistry WebBook).
RAW264.7 cell culture
RAW264.7 cell line was purchased from the Shanghai Institute of Cell Biology (Shanghai, China). The cells were cultured in DMEM supplemented with 10% FBS at 37 ˆC in a 5% CO2 incubator.
Cell viability
Cell viability was determined by CCK8 assay. The cells were seeded in 96-well plates at a density of 4 × 104 cells/ml, and 100 μl of fresh media containing different concentrations of AO (0, 25, 50, 125, 250, and 500 μg/ml) were added to each well. After 24 h of incubation, 10 μl of CCK8 reagent was added to each well, and subsequently, the cells were incubated at 37 ˆC for 2 h. Absorbance was measured at 450 nm using a microplate reader (Synergy 2, BioTek). Cell viability was calculated using the following formula: rate of cell viability (%) = (OD of the AO – OD of the blank) / (OD of the control- OD of the blank)× 100%.
Assay for detection of NO, TNF-α, and IL-6 in the cells
LPS was used to induce inflammatory damage in RAW264.7 cells. The cells were seeded in 96-well plates at a density of 4 × 104 cells/ml, and 200 μl of fresh medium containing AO (250 μg/ml) was added to each well. The cells were incubated with the essential oil for 1 h and then induced by treatment with 1 μg/ml LPS for 24 h. Subsequently, the cellular supernatant was collected. The concentrations of NO, TNF-α, and IL-6 were measured using detection kits following the protocols mentioned in the respective user manuals.
Experimental animals
All experimental protocols were approved by the Animal Care and Use Committee of the Shanghai University of Traditional Chinese Medicine (Shanghai, China; approval number SCXK (H) 2018-0004). Adult male Kunming mice (7 weeks old, weighing 18-22 g) were purchased from Shanghai Jiesijie Laboratory Animal Co., Ltd. (Shanghai, China). The mice were housed in cages with controlled temperature and humidity under 12 h light/12 h dark conditions; free access to drinking water and food was provided. Prior to the experiments, all mice were acclimatized for approximately 7 days.
Treatments and establishment of xylene-induced ear edema model
The care and use of animals in the experiment were in accordance with the regulations of animal ethics. The number of mice in each group were in accordance with the preliminary experimental results and regulations of animal ethics (Replacement, Reduction, Refinement). All 32 experimental mice were randomly divided into four groups by the random number table (n = 8 per group): control (saline), model (saline), positive (DSZBC), and AO (AO). The right ears of mice in the four groups were topically treated once a day for 5 days; AO and DSZBC were smeared on the right ears of AO and DSZBC mice, respectively; the saline solution was applied on the right ears of the mice in the other two groups. The topical application of xylene induced cutaneous inflammation. After 1 h of the last administration, 20 μl of xylene was applied to the inner surface of the right ear in all groups except the control group; the left ear was not subjected to this treatment. 30 min after the treatment, the mice were anesthetized by pentobarbital sodium, then the ear was cut and blood collected. After the blood collection, the mice were in light narcosis but still alive, so they were finally euthanized by cervical dislocation. The ears were cut using an 8-mm-diameter perforator and weighed. The degree of ear swelling was calculated using the following formula: degree of ear edema (%) = (weight of the right ear – weight of the left ear) / weight of the left ear × 100%.
Hematoxylin and eosin (H&E) staining
The right ear samples were rinsed in PBS, fixed in 4% paraformaldehyde, embedded in paraffin, and then serially sectioned at a thickness of 5 μm. The sections were stained with H&E to detect the inflammatory cells and the epidermal thickness. The images were captured using a digital microscope (Zeiss).
Determination of TNF-α level in the serum
Blood samples were obtained from the anesthetized mice via the aorta abdominalis. After being maintained at room temperature (25ˆC) for 2 h, the serum was centrifuged at 3500 rpm for 10 min at 4ˆC and collected. Samples with severe hemolysis were discarded. The TNF-α concentration in the serum was measured using an enzyme-linked immunosorbent assay (ELISA) kit. Briefly, the serum was diluted by sample analysis buffer, then 100μl of the diluted sample or standard were added to the pre-coated 96-well plate, the next steps were to follow the manufacturer’s instructions.
Statistical analysis
The data were presented as means ± SD. Statistical comparisons were performed by one-way analysis of variance (ANOVA) followed by Tukey’s post hoc analysis using the GraphPad Prism 8 software, and the level of statistical significance was set at p < 0.05.
Results
Chemical analysis of essential oil of A. annua (AO) by GC–MS
The constituents of AO were sorted based on their retention times and GC peak areas. The yield of the extracted AO was 1.2%. GC–MS analysis of AO revealed the presence of 13 compounds, representing 99.82% of the total constituents [Figure (A and B)]. The compounds whose concentrations were the highest were caryophyllene (32.16%), eucalyptol (28.62%), α-pinene (19.08%), and β-pinene (14.72%). The chemical compositions and the structures of the major compounds are presented in Table and Figure (C), respectively.
Figure 1. Chemical identification of AO by GC-MS. A. Total ion chromatograms of the AO. B. The mass spectrogram of every component. C. Chemical structures of major compounds identified in AO. AO: Artemisia annua L. essential oil; (1): α-Pinene; (2): Camphene; (3): β-Pinene; (4): β- Myrcene; (5): β-Cymene; (6): Eucalyptol; (7): γ-Terpinene; (8): (-)-trans-Pinocarveol; (9): Camphor; (10): Terpine-4-ol; (11): Caryophyllene; (12): Germacrene D; (13): Caryophyllene oxide.
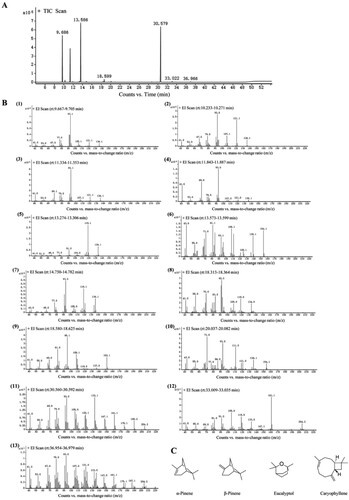
Table 1. Chemical composition of AO identified by GC-MS.
Effect of AO on cell viability
RAW264.7 cells were exposed to several concentrations of AO (ranging from 25 to 500 μg/ml) to determine a non-toxic concentration for use in the anti-inflammatory assay. As shown in Figure , compared with that observed in the control group (not treated with AO), the viability of cells treated with different AO concentrations was unaffected. These data suggest that AO is not toxic to RAW264.7 cells.
Effect of AO on the levels of NO, TNF-α, and IL-6 in LPS-induced RAW264.7 cells
Pro-inflammatory mediators such as NO, TNF-α, and IL-6 aggravate the symptoms of chronic inflammation. As shown in Figure , compared to that observed in the control (Con) group, overproduction of these mediators was observed in LPS-stimulated cells (p < 0.001). Pre-treatment with AO reduced the levels of these LPS-induced mediators (p < 0.01 and p < 0.001). The inhibition rates of NO, TNF-α, and IL-6 were 47.84, 26.57, and 55.55%, respectively.
Effect of AO on xylene-induced ear swelling
We investigated the effect of AO on xylene-induced mouse ear swelling. After the application of xylene, the weight of the ear increased due to the activation of the inflammatory reaction. Five samples were selected randomly from each group for statistics. As shown in Figure , compared to the model group, AO significantly inhibited ear edema, with an inhibition rate of 38%. DSZBC is a drug with anti-inflammatory, anti-allergic, and anti-pruritic effects and serves as a positive control. Ear edema was inhibited to a greater extent after treatment with DSZBC than that with AO; the inhibition rate observed after DSZBC treatment was 62%.
Effects of AO observed by analysis of pathological sections of the ear
The effects of AO on epidermal thickness and inflammatory cell infiltration were assessed using histopathological analysis. Five samples were selected randomly from each group for statistics. As shown in Figure (A), an increase in the infiltration of inflammatory cells was accompanied by an increase in vascular permeability in the model group. Three microscopic fields per pathological section were selected to count the number of inflammatory cells. The results showed that the number of macrophages increased in the model group compared to that in the control group (p < 0.001). AO and DSZBC inhibited the increase in the number of inflammatory cells induced by xylene (p < 0.001). No significant difference was observed between the results obtained from AO and DSZBC groups (p > 0.05).
Figure 5. Effect of AO on histopathological morphology. A. Representative HE staining; B and C. Statistical quantification of number of macrophages and epidermal thickness. Data represent the mean ± SD (n = 5). ***p < 0.001 vs Con, ### p < 0.001 vs xylene. Con: Control; AO: Artemisia annua L. essential oil; positive: DSZBC.
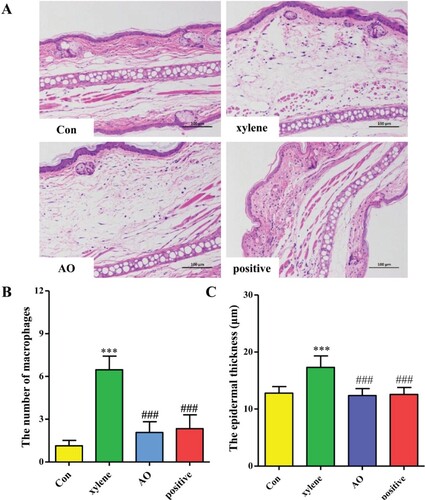
We also measured the epidermal thickness using ImageJ. We found that the epidermal thickness in the model group was significantly greater than that in the control group (p < 0.001). Compared to that in the model group, the epidermal thickness in the AO and DSZBC groups decreased (p < 0.001), and no differences were obtained among the findings for the control, AO, and DSZBC groups (p > 0.05).
Effect of AO on the level of TNF-α in serum
While collecting the serum, some samples had severe hemolysis, and some samples were insufficient to get serum after being centrifuged. If the sample was sufficient, the same number of samples were selected randomly for statistics. So the exact value of n is 5 in each group in the analysis of TNF-α. As shown in Figure , the level of TNF-α was elevated in the model group compared to that in the control group (p < 0.001), whereas the level of TNF-α in the mice pretreated with AO or DSZBC was significantly decreased compared to that in the model group (p < 0.001). No significant differences were obtained among the findings for the AO and the DSZBC groups (p > 0.05).
Discussion
Essential oils are hydrophobic and lipophilic concentrates containing volatile aromatic compounds extracted from flowers, leaves, roots, stems, bark, fruits, buds, and seeds of various aromatic plants (Zhang et al. Citation2021). Essential oils are rarely toxic to mammals and are considered environmentally safe because of their low persistence rates (Lazarvic et al. Citation2020). The yield and the chemical composition of essential oils are closely related to the extraction techniques used (Chinese Pharmacopoeia Commission Citation2020). Several methods have been used to extract essential oils, including hydrodistillation, steam distillation, supercritical carbon dioxide extraction, ultrasound-assisted extraction, organic solvent extraction, cold pressing, molecular distillation, supercritical water extraction, enzyme-assisted extraction, and microwave extraction. Among these, hydrodistillation and steam distillation are standardized methods for obtaining essential oils.
The essential oil of A. annua (AO) is used in Chinese medicine. With the discovery of artemisinin and its antimalarial activity, A. annua has received attention worldwide. AO is also raising interest as it is an important ingredient in A. annua. In our lab, AO was extracted by hydrodistillation from fresh leaves of A. annua, which were collected in May in the Henan province, China. Many papers have been published on the chemical components of the essential oil obtained from A. annua. Yang et al. (Citation2012) found that the main components of essential oils obtained from A. annua at the full flowering stage were oxygenated monoterpenes and sesquiterpene hydrocarbons. The essential oils of A. annua extracted from plants grown in Italy (Donato et al. Citation2015), Serbia (Acimovic et al. Citation2022), and Iran (Habibi et al. Citation2013) contain the artemisia ketone as the dominant compound. In our study, GC–MS analysis revealed the presence of 13 compounds in AO, among which the major compounds were caryophyllene (32.16%), eucalyptol (28.62%), α-pinene (19.08%), and β-pinene (14.72%). The major component of AO in this study differed from that reported in the literature. Such differences may be due to variations in the plant genotype, growth stage, region, altitude, climate, and other factors at the time of harvest, as well as in the extraction parameters and drying methods (Rana et al. Citation2013).
AO has been demonstrated to have good antiviral, antitumor, antibacterial, anti-parasitic, antioxidant, anti-inflammatory, and immune-boosting properties and shows low toxicity (Abad et al. Citation2012; Bilia et al. Citation2014; Kim et al. Citation2015; Zhao et al. Citation2016; Taleghani et al. Citation2020; Fu et al. Citation2022). This study focused on the anti-inflammatory effects of AO administered through the skin. Inflammation is the primary component of a complex reaction triggered by tissue injury, stress, or infection and helps to restore tissue homeostasis. The inflammatory response is characterized by redness, edema, temperature increase, pain, and decrease in tissue function resulting from vascular and cellular events (Chen et al. Citation2018). As part of the inflammatory response, monocytes and macrophages are activated, and secrete a large number of pro-inflammatory cytokines (IL-6 and TNF-α) that play crucial roles in the development of inflammatory diseases. Inflammatory mediators such as NO, TNF-α, and IL-6 are some examples of cell signaling molecules that promote prolonged inflammation; if the production of these molecules is not controlled, it can result in chronic inflammation (Qu et al. Citation2019). However, self-damage caused by inflammation is unavoidable, and non-resolution of this condition leads to pathological conditions, necessitating the development of safe anti-inflammatory agents. In vitro, AO had no toxic effects on cells in the range of the measured concentration (25μg/ml – 500μg/ml), which fully demonstrated the safety of AO. Meanwhile, according to many essential oil related references (Egbuta et al. Citation2022; Liu et al. Citation2022), we selected one of the concentrations as the treatment concentration in the further assay. We observed that 250μg/ml AO markedly reduced the levels of NO, IL-6, and TNF-α, whose expression was induced by LPS-treatment of RAW264.7 cells. The results suggested that the AO extracted in our lab had anti-inflammatory effects and was non-toxic.
An in vivo xylene-stimulated ear swelling model was used in this study. In preliminary experiments, in which AO was applied to conduct skin irritation tests, we used 10%, 25%, 50% AO without stimulation to topical skin. In order to work on the ear edema model, we selected the treatment with 50% AO in this animal examination. The right ears of mice were pretreated with AO or DSZBC for 5 days. DSZBC, containing 0.04% dexamethasone acetate, 2% camphor, 1% menthol, was a hospital preparation obtained from the Shanghai Skin Disease Hospital. It is widely used to treat local pruritus, neurodermatitis, contact dermatitis, seborrheic dermatitis, eczema, and other skin diseases. In our study, DSZBC, which possesses anti-inflammatory, anti-allergic, and anti-pruritic effects, was used as a positive control. AO and DSZBC were applied to the skin. Finally, xylene was applied to the inner surface of the right ear. The model with ear edema induced by xylene is a classic model of acute inflammation. In the literature, after the application of xylene, the ear edema rate was decreased with the retention time prolonged, and the optimal mouse model of ear edema is induced by applying 20 μl xylene with 30 min retention. Xylene caused remarkable inflammatory changes in the model group after 30 min, such as thickening of the epidermis, infiltration of macrophage cells, and high-expression of TNF-α compared with the control group. AO and DSZBC inhibited xylene-induced inflammatory changes. No significant differences were observed between the findings for the AO and the DSZBC groups.
Transdermal drug delivery systems have a huge market potential. However, transdermal delivery is limited due to the inability of most ingredients to cross the skin barrier. The hydrophobic and lipophilic properties, coupled with the low molecular weights of essential oils, lead to easy absorption of essential oils through the skin. In a previous study (Zhu et al. Citation2022), we found that AO increased the percutaneous absorption of celastol to the highest level, and the penetration-promoting mechanism was related to the effect of AO on the skin structure and the changes in thermodynamic properties of the skin. The skin is the largest organ, stimulated easily by ultraviolet radiation and dust; therefore, it is prone to inflammation. An inappropriate or excessive cutaneous inflammation promotes the infiltration of inflammatory cells and stimulates the release of various inflammatory mediators, which cause skin problems, such as skin allergy, pigmentation, skin aging, acne, eczema, and atopic dermatitis. Anti-inflammatory drugs are widely used in the field of dermatology. However, lots of drugs are associated with adverse effects and drug resistance. The results of this study provide pharmacological evidence for the anti-inflammatory effects of AO. AO is a natural penetration enhancer with excellent therapeutic efficiency. AO is a potential raw material for the synthesis of cosmetic products and external preparations to alleviate skin inflammation.
Research on the anti-inflammatory effects of AO has provided an experimental basis for its application on the skin. Compared to chemically synthesized agents, AO is derived from plants and provides an option for sustainable and environment-friendly management of raw materials. Our study had several limitations that should be addressed in future research. The biological activities of essential oils are linked to the differences in their chemical composition. In future research, the major components of AO should be separated, and their biological activities should be determined. In addition, a combination of essential oils should be investigated to determine whether they have synergistic or additive effects.
Conclusions
AO significantly inhibited LPS-induced RAW264.7 cells and xylene-induced ear swelling, proving that it is an effective natural anti-inflammatory agent. These findings provide a basis for future studies on the efficacy of AO and pave the way for the potential application of AO in the synthesis of external preparations in pharmacological and cosmetic industries.
Author contributions
The researchers conducting the experiment were different to the researchers analysing the outcomes, so as to minimize the influence of human factors. Each author’s contribution is as follows: Congcong Zhu and Manqi Hu conducted the experiments; Zongguang Tai, Ge Yan and Bin Fan analyzed and interpreted the data; Zongguang Tai and Pei Zhao were responsible for the statistical analysis; Xiao Miao and Quangang Zhu designed the research; Xiao Miao drafted the manuscript; Ge Yan was responsible for the correspondence and revision of the manuscript in the process of submission. Congcong Zhu and Ge Yan contributed equally to the article.
Disclosure statement
No potential conflict of interest was reported by the author(s).
Data availability statement
The data that support the findings of this study are openly available in Mendeley Data at http://doi.org/10.17632/k6j6c2gnkh.1.
Additional information
Funding
References
- Abad MJ, Bedoya LM, Apaza L, Bermejo P. 2012. The artemisia L. genus: a review of bioactive essential oils. Molecules. 17:2542–2566. doi:10.3390/molecules17032542.
- Acimovic M, Jeremic JS, Todosijevic M, Kiprovski B, Vidovic S, Vladic J, Pezo L. 2022. Comparative study of the essential oil and hydrosol composition of Sweet Wormwood (Artemisia annua L.) from Serbia. Chem Biodivers. 19:e202100954. doi:10.1002/cbdv.202100954.
- Bilia AR, Santomauro F, Sacco C, Bergonzi MC, Donato R. 2014. Essential oil of Artemisia annua L.: an extraordinary component with numerous antimicrobial properties. Evid Based Complement Alternal Med. Article ID159819, 7. doi:10.1155/2014/159819.
- Bitencourt MAO, Dantas GR, Lira DP, Barbosa-Filho JM, Miranda GEC, Santos BVO, Souto JT. 2011. Caulerpa Mexicana aqueous and methanolic extracts of Caulerpa mexicana suppress Cell Migration and Ear Edema Induced by Inflammatory Agents. Mar Drugs. 9(8):1332–1345. 103390/md9081332.
- Borges RS, Ortiz BLS, Pereira ACM, Keita H, Carvalho JCT. 2019. Rosmarinus officinalis essential oil: a review of its phytochemistry, anti-inflammatory activity, and mechanisms of action involved. J Ethnopharmacol. doi:10.1016/1j.jep.2018.09.038.
- Chen L, Deng H, Cui H, Fang J, Zuo Z, Deng J. 2018. Inflammatory responses and inflammation associated diseases in organs. Oncotarget. 9:7204–7218. doi:10.18632/oncotarget.23208.
- Chen LC, Lin YY, Jean YH, Lu Y, Chen WF, Yang SN, Wang HM, Jang IY, Chen IM, Su JH, et al. 2014. Anti-inflammatory and analgesic effects of the Marine-Derived Compound Comaparvin Isolated from the Crinoid Comanthus bennetti. Molecules. 19(9):14667–14686. doi:10.3390/molecules190914667.
- Chinese Pharmacopoeia Commission. 2020. Chinese pharmacopoeia (2020). Beijing: The medicine science and technology press of China.
- Chu KK, Chen DD, Li XM, Miao MS. 2022. Research and application of Chinese medicinal essential oil: based on data mining and Chinese pharmacopoeia(2020). Pharmacol Clinics Chin Materia Medica. doi:10.13412/j.cnki.zyyl.
- Donato R, Santomauro F, Bilia AR, Flamini G, Sacco C. 2015. Antibacterial activity of Tuscan Artemisia annua essential oil and its major components against some foodborne pathogens. Food Sci Technol. 64:1251–1254. doi:10.1016/j.lwt.2015.07.014.
- Egbuta MA, McIntosh S, Waters DLE, Vancov T, Liu L. 2022. In vitro anti-inflammatory activity of essential oil and β-Bisabolol derived from Cotton Gin Trash. Molecules. 27:526. doi:10.3390/molecules27020526.
- Feng J, Li H, Li J, Meng P, Wang L, Liu C, Zhao S, Sun W, Zhang Y. 2020. hnRNPK knockdown alleviates NLRP3 inflammasome priming by repressing FLIP expression in Raw264.7 macrophages. Redox Rep. 25(1):104–111. doi:10.1080/13510002.2020.1857157.
- Fu C, Zhang K, Wang M, Qiu F. 2022. Casticin and chrysosplenol D from Artemisia annua L. induce apoptosis by inhibiting topoisomerase IIα in human non-small-cell lung cancer cells. Phytomedicine. 100:154095–154108. doi:10.1016/j.phymed.2022.154095.
- Gias ZT, Afsana F, Debnath P, Alam MS, Ena TN, Hossain MH, Jain P, Reza HM. 2020. A mechanistic approach to HPLC analysis, antinociceptive, anti-inflammatory and postoperative analgesic activities of panch phoron in mice. BMC Complement Med Ther. 20:102–110. doi:10.1186/s12906-020-02891-x.
- Habibi Z, Ghaniam S, Ghasemi S, Yousefi M. 2013. Chemical composition and antibacterial activity of the volatile oil from seeds of Artemisia annua L. from Iran. Nat Prod Res. 27:198–200. doi:10.1080/14786419.2012.662652.
- Kim WS, Choi WJ, Lee S, Kim WJ, Lee DC, Sohn UD, Shin HS, Kim W. 2015. Anti-inflammatory, antioxidant and antimicrobial effects of Artemisinin extracts from Artemisia annua L. Korean J Physiol Pharmacol. 19:21–27. doi:10.4196/kjpp.2015.19.1.21.
- Lazarvic J, Jevremovic S, Kostic I, Kostic M, Vuleta A, Manitasevic JS, Seslija JD. 2020. Toxic, oviposition deterrent and oxidative stress effects of essential oil against Acanthoscelides obtectus. Insects. 11:563–581. doi:10.3390/insects11090563.
- Liu CX. 2017. Discovery and development of artemisinin and related compounds. CH M. 9:101–114. doi:10.1016/S1674-6384(17)60084-4.
- Liu XJ, Li Y, Su SL, Wei DD, Yan H, Guo S, Shang EX, Sun XD, Duan JA. 2022. Comparative analysis of chemical composition and antibacterial and anti-inflammatory activities of the essential oils from Chrysanthemum morifolium of different flowering stages and different parts. Evid Based Compl Alt. Article ID 5954963, 10. doi:10.1155/2022/5954963.
- Muller WE, Sillani G, Schuwald A, Friedland K. 2021. Pharmacological basis of the anxiolytic and antidepressant properties of Silexan®, an essential oil from the flowers of lavender. Neurochem Int. 143:104899. doi:10.1016/j.neuint.2020.104899.
- Oh YC, Jeong YH, Cho WK, Ha JH, Gu MJ, Ma JY. 2015. Anti-inflammatory and analgesic effects of Pyeongwisan on LPS-stimulated murine macrophages and mouse models of acetic acid-induced writhing response and Xylene-induced ear edema.2015. Int J Mol Sci. 16(1):1232–1251. doi:10.3390/ijms16011232.
- Pan SY, Zhou SF, Gao SH, Yu ZL, Zhang SF, Tang MK, Sun JN, Ma DL, Han YF, Fong WF, Ko KM. 2013. New perspectives on how to discover drugs from herbal medicines: CAM’s outstanding contribution to modern therapeutics. Evid Based Compl Alt. Article ID 627375, 25. doi:10.1155/2013/627375.
- Qu Z, Zhao J, Zhu L, Huang L, Ma Y, Ma C, Luo C, Zhu Z, Yuan Z, Wu J, et al. 2019. Anti-inflammatory effect and potential mechanism of betulinic acid on λ-carrageenan-induced paw edema in mice. Biomed Pharmacother. 118:109347–110945. doi:10.1016/j.biopha.2019.109347.
- Rana VS, Blazquez MA, Maiti S. 2013. Essential oil composition of Artemisia annua L. at different growth stages. J Spices Arom Crops. 22:181–187. https://www.researchgate.net/publication/262167418.
- Taleghani A, Emami SA, Tayarani-Najaran Z. 2020. Artemisia: a promising plant for the treatment of cancer. Bioorg Med Chem. 28:115180. doi:10.1016/j.bmc.2019.115180.
- Tarumi W, Shinohara K. 2020. The effects of essential oil on salivary oxytocin concentration in postmenopausal women. J Altern Compliment Med. 26:226–230. doi:10.1089/acm.2019.0361.
- Van HT, Thang TD, Luu TN, Doan VD. 2021. An overview of the chemical composition and biological activities of essential oils from genus (Zingiberaceae). RSC Adv. 11:37767–37783. doi:10.1039/D1RA07370B.
- Yang ZN, Zhu SQ, Yu ZW. 2012. Comparison of terpene components from flowers of Artemisia annua. Bangladesh J Pharmacol. 7:114–119. doi:10.3329/bjp.v7i2.10815.
- Yatoo GN, Wani H, Shah SA, Zargar MI, Rather MA, Banday JA. 2021. Gas chromatographic-mass spectrometric analysis, antibacterial, antioxidant and antiproliative activities of the needle essential oil of Abies pindrow growing wild in Kashmir, India. Microb Pathog. 158:105013. doi:10.1016/j.micpath.2021.105013.
- Zhang SQ, Xu HB, Zhang SJ, Li XY. 2020. Identification of the active compounds and significant pathways of Artemisia annua in the treatment of non-small cell lung carcinoma based on network pharmacology. Med Sci Monit. 26:e923624. doi:10.12659/MSM.923624.
- Zhang Y, Long Y, Yu S, Li D, Yang M, Guan Y, Zhang D, Wan J, Liu S, Shi A, Li N. 2021. Natural volatile oils derived from herbal medicines: a promising therapy way for treating depressive disorder. Pharm Res. 164:105376. doi:10.1016/j.phrs.2020.105376.
- Zhang Z, Luo D, Xie J, Xian Y, Lai Z, Liu Y, Liu W, Chen J, Lai X, Lin Z, Su Z. 2018. Curcumin’s Metabolites, Tetrahydrocurcumin and Octahydrocurcumin, possess superior anti-inflammatory effects in vivo through suppression of TAK1-NF-κB pathway. Front Pharmacol. 9:1181–1192. doi:10.3389/fphar.2018.01181.
- Zhao YP, Wang H, Yang G, Qiu ZD, Qu XB, Feng WH. 2016. Anti-tumor mechanism of Artemisia annua based on bioinformatics. China J Chin Mater Med. 41:2695–2700. doi:10.4268/cjcmm20161421.
- Zhu CC, Yu Q, Pan HJ, Chen Y, Miao X, Zhu QG. 2022. Effect of artemisia oil in the nanoemulsion as an oil excipient and enhancer on percutaneous absorption of Celastrol. Chin J Mod Appl Pharm. 39:1054–1058. doi:10.13748/j.cnki.issn1007-7693.2022.08.008.
- Zi Y, Yao M, Lu Z, Lu F, Bie X, Zhang C, Zhao H. 2021. Glycoglycerolipids from the leaves of Perilla frutescens (L.) Britton (Labiatae) and their anti-inflammatory activities in lipopolysaccharide-stimulated RAW264.7 cells. Phytochemistry. 184:112679–112679. doi:10.1016/j.phytochem.2021.112679.