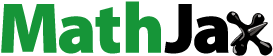
Abstract
The molecular phylogeny of Kazakhstan’s Fritillaria karelinii (Fisch. ex D. Don) Baker was reconstructed and analyzed to confirm its genetic identification and phylogenetic position. The BLAST results were matched at a high rate of 98%, and (nrITS and rbcL) formed a single clade in the phylogenetic analysis. The studied plant belongs to the branch of the subgenus Fritillaria Tourn. ex L., which is consistent with the literature. This confirmed a clear genetic identification and taxonomic position of the studied plant. Despite some sequence differences between the Kazakhstani and Chinese species (C1, C3, C5) found in the nrITS region, no specific sequence existed for the other markers (rbcL and trnH-psbA). The outcomes of the antioxidant activity of F. karelinii fractions were prepared by extraction with 70% ethanol and separation into several fractions using the partition procedure with chloroform, ethyl acetate, n-butanol, and water. Among these, the ethyl acetate fraction most significantly affected the activity in the FC, DPPH, and FRAP assays.
Introduction
Fritillaria Tourn. ex L. is a genus of plant species from Liliaceae Juss. family. Around 140 bulbous perennial species are distributed in the Northern Hemisphere temperate zones, which are currently divided into eight subgenera (Rix Citation2001; Day et al. Citation2014; Hill Citation2022). Most of these species are used as ornamental plants (Ivashchenko Citation2008; Day et al. Citation2014), medicinal plants, and some as food plants (Rashid and Yaqoob Citation2021). The bulbs of the Fritillaria species are utilized as a decoction from dried bulbs to cure dry cough, lung infections, asthma, and bronchitis (Shaheen et al. Citation2014; Abbaszadeh et al. Citation2019).
Baitenov (Citation2001) identified five species of Fritillaria L. in the Kazakhstan flora, including the rare species F. pallidiflora Schrenk, as well as two species from the Rhinopetalum F. E. Fischer ex Alexander genera (Rh. karelenii Fisch. ex D. Don and Rh. stenantherum Regel). However, only F. pallidiflora is listed in the Kazakhstan Red Book (Citation2014). The latest nomenclature changes in Plants of the World Online show that Rhinopetalum karelinii is a synonym of Fritillaria karelinii (Fisch. ex D. Don) Baker which grows in Kazakhstan, Kyrgyzstan, Tajikistan, Turkmenistan, Uzbekistan, and Xinjiang (Rønsted et al. Citation2005, powo.science.kew.org/results?q = Fritillaria%20karelinii).
Studies on the introduction of F. karelinii in the Main botanical garden in Almaty have been carried out since 1958, and in the Zhezkazgan botanical garden since 1978. However, all of these studies have been sporadic (Ivashchenko et al. Citation2023).
Fritillaria karelinii Fisch. ex D. Don is a perennial herb grows up to 10–20 cm tall. It has a white spherical bulb covered by yellowish scales. The stem is straight, and the short villous pubescent leaves are close together, nearly opposite, glabrous, smooth, lanceolate-oblong 0.5-1.5 cm wide, or lanceolate in shape. The bract leaves are linear-lanceolate, wavy along the edge, and longer than the flowers. The drooping pedicels, within bracts densely pubescent with short villous hairs, equal to or shorter than flowers. The perianth is pink-violet at the base with a dark spot, deeply divided into oblong-oval lobes with a clear checkerboard pattern, of which the upper one has a larger spur-shaped outgrowth. The stamens have short thin filaments that are several times shorter than the perianth and spherical villous anthers. The style is longer than stamens with a small stigma. The capsule is almost rounded in shape and has narrowed base that leads into a stem with six smoothed ribs, without wings, with numerous flat greyish – brown seeds. Blooms in March – April. Plants occurs along clayey and sandy desert steppes and dry foothills (Flora of Kazakhstan Citation1958).
According to the information in Kazakhstan’s flora (1958), this particular species is found in 16 floristic regions within the country’s territory. Additionally, the herbarium AA (Institute of Botany and Phytointroduciton) has collected over 40 specimens from 10 different floristic districts in Kazakhstan (Figure , Table ). These indicates that the examined species has a wide distribution throughout the country.
Figure 1. Floristic regiones and distribution of Fritillaria karelinii on the territory of Kazakhstan.
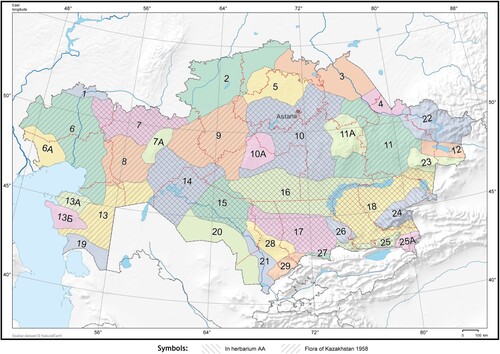
Table 1. Distribution of F. karelinii on the territories of Kazakhstan floristic regions.
The initial account of F. karelinii was collected from West Kazakhstan in 1830 by F.B. Fisher (1782–1854), a Russian botanist and the founder of the St. Petersburg Botanical Garden. The species was named after G.S. Karelin (1801–1872), who conducted a study the flora in that region. The species specimens are currently stored in St. Petersburg (Ivaschenko, Citation2005).
Despite being widespread and used for its honey, perganous, and decorative properties, F. karelinii is already protected in Ustyurt and Barsa-Kelmes the reserves territories (Tursynbay et al. Citation2020).
Fritillaria genera are divided into several subgroups and corresponding a certain species to a specific subgroup requires a complete and more thorough genome analysis. This has been confirmed in numerous studies (Ronsted et al. Citation2005; Yun et al. Citation2008; Xiang et al. Citation2016; Zhang et al. Citation2021). For instance, Chen et al. (Citation2022) classified 23 Fritillaria species that grow in China have been grouped into four clades based on the geographical distribution. The analyzed species showed a high percentage of coincidences with this classification.
Recently, a growing interest has been in exploring the potential of natural drugs derived from plant species. However, the uncontrolled harvesting of wild-growing medicinal plants poses a significant threat to their survival. It is crucial to implement sustainable and well-regulated harvesting practices to ensure the conservation of the biological diversity of these plants, especially those that are indigenous and have valuable genetic diversity. Therefore, conducting studies on the protection of wild medicinal plants is one of the most important issue.
Fritillaria species have been widely used in traditional Chinese medicine for centuries due to their various pharmaceutical active ingredients. The research on their chemotaxis, molecular phylogeny, and pharmacology have also been conducted, and leads to the discovery and isolation of new steroid alkaloids, saponins, terpenoids, glycosides, and many other compounds in various Fritillaria species. Molecular phylogenetic methods based on nuclear and chloroplast DNA sequences have related within and between the Chinese species Bei Mu and species growing outside of China. Therefore, it is essential to continue studying more species for sustainable use (Hao et al. Citation2013).
F. karelinii is not listed in the official medicine and has been poorly studied in Kazakhstan. However, the bulbs, along with the Siberian fritillary (F. pallidiflora Schrenk ex Fisch & C.A.Mey.) have become a target of spontaneous and predatory harvesting in the southeast region of Kazakhstan for export into China. Where the bulbs are used as antitussive and exhilarating drugs known as ‘Bei-mu and/or Pei-mu' (Chinese Pharmacopoeia Commission Citation2015; Nile et al. Citation2021). This has increased concerns about the sustainability of this species and the need for further research to understand its potential medicinal benefits.
In this study, we utilized DNA barcoding to verify the identity of F. karelinii and conducted a qualitative comparative analysis of fractions to determine their antioxidant activities. F. karelinii is currently endangered in Kazakhstan due to reckless overharvesting.
Material and methods
Three accessions of Fritillaria karelinii (Fisch. ex D. Don) Baker were collected from different locations in the Almaty region in 2022 (Figure , Table ). Associated locational details, including the longitude, latitude, and altitude were recorded in the field during the collection period. Herbarium sheets were prepared by the standard taxonomic procedure (Skvorcov Citation1977). The identified herbarium specimens were submitted to the IBPh herbarium (AA), Almaty, Kazakhstan, and the KRIBB herbarium (KRIB), Daejeon, South Korea.
Figure 2. Collection sites of F. karelinnii in Almaty region.
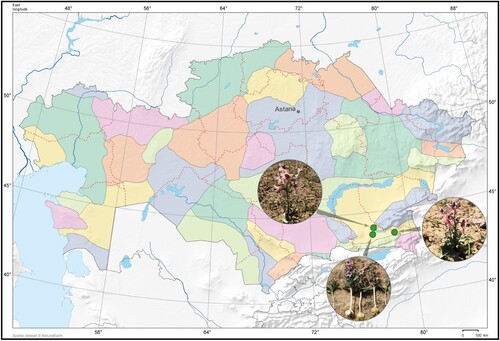
Table 2. Information on nucleotide sequence of Fritillaria karelinii collected from different populations in the Almaty region.
Materials and instruments
DNA extraction, PCR amplification, and sequencing.
Total genomic DNAs was extracted from silica-dried leaves of the three F. karelinii samples using the DNeasy Plant Mini Kit (Qiagen). The quality of the gDNAs was checked using a Nanodrop (Thermo Fisher Scientific) and 1.0% agarose gel electrophoresis.
For species identification, we used three DNA barcoding markers (rbcL, trnH-psbA, and nrITS). The universal DNA barcoding primer information is summarized in Tables and . The PCR conditions were as follows: denaturation for 3 min at 95 °C; 30 cycles of 30 at 95 °C;, 1.5 min at 55 °C, and 2 min at 72 °C and a final extension of 7 min at 72 °C. The PCR amplification results were confirmed by 1.0% agarose gel electrophoresis.
Table 3. DNA barcoding primer information in this study.
Purification and Sanger sequencing (ABI 3730xl) of the PCR products were performed by Macrogen. The generated nucleotide sequences were registered in the GenBank database of the National Center for Biotechnology Information (NCBI) (Table ).
DNA barcoding analysis. Sequences obtained by Sanger sequencing were subjected to de novo assembly using Geneious Prime version 2023.1.1. (licensee KRIBB) Primer sequences were removed from the contigs. The final sequences were checked using the Nucleotide BLAST program of NCBI GenBank (https://blast.ncbi.nlm.nih.gov/Blast.cgi).
To construct the phylogenetic tree, the rbcL, trnH-psbA, and nrITS sequences of plants belonging to the genus Fritillaria were downloaded from GenBank (Supplement 1). Two Lilium species (L. pensylvanicum and L. regale) were used as outgroups. Sequence alignments of three DNA barcoding regions (nrITS, 63 accessions; rbcL, 60 accessions) were performed using MAFFT v.7.490 (Katoh & Standley Citation2013) in Geneious Prime v.2023.1.1. The aligned sequence lengths for each dataset were 721 bp (ITS) and 602 bp (rbcL) (Supplement 2).
Both datasets (nrITS and cpDNA rbcL markers) were analyzed separately for position identification in the genus Fritilaria and to find the closest relatives of F. karelinii through Fitch parsimony using the heuristic search option in PAUP version 4.0b10 (Swofford Citation2004) with MULTREES, TBR branch swapping and 100 replicates of random addition sequences. These gaps were treated as missing data. The consistency index (CI) (Kluge & Farris Citation1969) was calculated to estimate the amount of homoplasy in the character set. The phylogenetic analysis was similar to the one that was carried out by Khan (Citation2022). The most parsimonious trees returned by the analysis were summarized into one consensus tree using the strict consensus method. Bootstrap analyses (BS) using 1,000 pseudoreplicates were performed to assess clade support of the clades (Felsenstein Citation1985). Bayesian phylogenetic analyses were performed using MrBayes 3.1.23 (Ronquist & Huelsenbeck Citation2003). The sequence evolution model was chosen according to the Akaike Information Criterion (AIC) obtained from jModelTest2 (Dariba et al. Citation2012). Two independent analyses with four Markov chains were run for 10 million generations, with trees sampled every 100 generations. The first 25% of trees were discarded as burn-ins. The remaining 150,000 trees were combined into a single dataset, and a majority-rule consensus tree was obtained along with posterior probabilities (PP).
However, a 12 bp small inversion was identified in two F. karelinii samples (KX354691.1 and MZ970254.1) in the trnH-psbA dataset. In order to increase the accuracy of the phylogenetic tree, this region was inverted and used to construct the phylogenetic tree.
Biochemicals for antioxidant analysis, such as ferric chloride (FeCl3), Folin–Ciocalteu reagent, 2,2-diphenyl-1-picrylhydrazyl (DPPH) and 2,2’-azobis(2-methylpropionamidine)dihydrochloride were purchased from local market (in Almaty, RK). A Rider Multiscan FC 357 device was used to make colorimetric measurements.
The FT-IR spectra were recorded as KBr pellets in an infrared spectrometer (Spectrum 65, PerkinElmer) in the spectral range between 4000 and 400 cm−1.
Extraction. Dry powdered bulbs of F.karellii (136 g) were percolated with 70% EtOH (400 ml) for one day and then extracted three times. The combined filtrates were concentrated to a volume of 300 ml. The filtrate was shaken using ChCl3 (4 × 250 ml), EtOA (4 × 300 ml) and n-BuOH (4 × 200 ml). The dried weights of the ChCl3, EtOA, n-BuOH and water filtrates were 1.97, 5.79, 4.31, and 26.44 g. Accordingly, the yields of these fractions from the crude extract were ∼5.11, 15.03, 11.19 and 65.0%, respectively.
Total phenolic compounds (TPC) were analyzed by the Folin – Ciocalteu method, according to the procedure of Denisenko et al. Citation2015. In brief, aliquots (40 µl) of ethanol extracts were mixed with 210 µL of sodium carbonate solution (Na2CO3) at a ration 1:10. The mixture was then incubated for 10 min at 38 °C. Afterward, 50 µL Folin–Ciocalteu reagent (1:6 with ethanol) was added to the mixture and allowed to react for 15 min at room temperature. The absorbance was determined at 720 nm. Gallic acid was used as a standard and the results were expressed as gallic acid equivalents (GAE) by the equation 1:
(1)
(1) where As is the absorbance of the extract sample and a and b are the slope and intercept of the calibration curve, respectively. TPC was calculated from the GAE and the concentration of the extract sample solution, C extract (2).
(2)
(2)
DPPH radical scavenging assay. The effect of extracts on DPPH radicals was measured using Reis et al. (Citation2012) method with some modifications. Three wells of the microplate were loaded with 20 µL of extract sample and 300 µL of DPPH (2,2-Azobis(2-methylpropionamidine)dihydrochloride), and another three wells were filled with 20 µL of solvent and 300 µL of DPPH (control). The microplate was incubated at room temperature for 30 min and then absorbance was measured at 517 nm. The following equation (3) calculated radical scavenging activity (RSA):
(3)
(3) where As-absorbance of sample and Ab-absorbance of blank (solvent, in our case EtOH).
FRAP assay. The ferric-reducing antioxidant power of the studied extracts was evaluated as described by Benzie & Devaki (Citation2018) with slight modifications. In brief, 300 mM acetic acid buffer (pH 3.6), 20 mM FeCl3, and 10 mM 2,4,6-tri(2-pyridyl)-s-triazine (TPTZ) (10:1:1, v/v/v) in 40 mM HCl were mixed and warmed in water at 37 °C for 5 min to prepare the working reagent. FeSO4 – 0.8 mM (1:5, v/v) was used as the positive control.
The concentration of ascorbic acid standard solution 0.1875µM/L and with the following dilutions (1-0.0125) were used. The extract samples 35 µL were mixed with 265 µL of FRAP solution and the absorbance of the mixture was measured at 620 nm after incubation at 37 °C for 4 min. The FRAP values of the extracts were calculated by the following equation:
(4)
(4) where As-absorbance of sample, Ab-absorbance of blank.
Statistical analysis. All data analyzed in this investigation were averaged and conducted in triplicate. The statistical analyses were performed using Microsoft Excel. The quantification of activities was based on a standard curve generated with gallic and ascorbic acid and the results were expressed as gallic acid equivalents (GAE).
Results
Species identification by blast
Species identity was determined by analysing the sequences of F. karelinii from Kazakhstan with the Basic Local Alignment Search Tool (NCBI-BLAST). The analyses confirmed that all nrITS and rbcL sequences matched Fritillaria karelinii. The pairwise identities of rbcL and trnH-psbA among the Kazakhstan and NCBI three F. karelinii species were found to be 100% and 99.8%, respectively. Additionally, the nrITS results showed a 97.9% pairwise identity.
Phylogenetic analysis
In order to determine the precise phylogenetic position within the genus Fritillaria, Fritillaria sequences from NCBI were downloaded and phylogenetic trees were prepared. Its shown that F. karelinii formed one clade in the nrITS and rbcL phylogenetic trees. The nrITS sequences, along with the 5.8S gene, were aligned with 63 accessions of Fritilaria species, including a selection of representatives from each section and 9 accessions of F. karelinii. The alignment consisted of 721 characters, out of which 144 were variable characters that were parsimony-informative. An unweighted parsimony analysis of the 63 sequences resulted in approximately 1.2 million most parsimonious trees of 482 steps (CI = 0.6037). The substitution model GTR + G was selected through AIC in JModeltest-2.1.7 for the Bayesian analysis. Both the Parsimony and Bayesian analyses produced identical topologies (see Figure ). All F. karelinii accessions formed a strongly supported monophyletic clade (PP 1 and BS 100%) together with F. gibboosa and F. stenanthera.
Figure 3. Phylogenetic tree of nrITS sequences of genus Fritillaria.
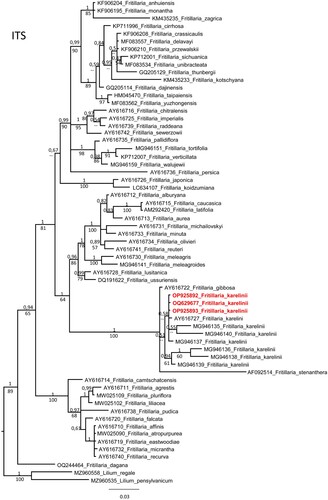
The rbcL alignments of sequences with 60 accessions of diverse Fritillaria species including six accessions of F. karelinii, had a total 602 characters. Out of these characters, only 12 were variable parsimony informative. An unweighted parsimony analysis of the 60 sequences resulted in approximately 52037 most parsimonious trees of 33 steps (CI = 0.6970). The substitution model GTR + I was chosen using AIC in JModeltest-2.1.7 for the Bayesian analysis. Both the Parsimony and Bayesian analyses produced identical topologies (Figure ).
Figure 4. Phylogenetic tree of rbcL sequences of genus Fritillaria.
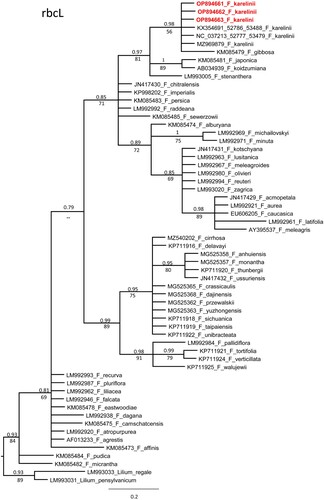
The topology of the plastid tree based only on rbcL is very different from the nrITS tree. However, all accessions of F. karelinii formed a monophyletic clade with F. gibbosa (PP 0.98, BS 56%) and further as a sister clade with F. japonica, F. koidzumiana and F. stenanther (PP 0.97, BS 81%). The bootstrap values (BV) of the F. karelinii clade were 74% and 55%, respectively.
Determination of total phenolic content
The total phenolic content (TPC) was estimated using the Folin–Ciocalteu (FC) reagent. A comparative examination of TPC was conducted for the aerial part and bulbs. Crude EtOH extracts were resuspended in 2 mL dichloromethane and the findings showed significant differences between the aerial part and bulbs. The TPC for the bulbs ranged from 210-260 µg, while the TPC for the aerial part was 400–480 µg GAE per gram of extracts.
The TPC of EtOA, n-BuOH and water extracts (after sequential extraction with organic solvents) was measured by extrapolation of the gallic acid standard calibration curve. The total phenol value was determined using the following regression equation:
where y is the absorbance at 720 nm and x is the concentration of GA expressed in µg/ml.
TPC was estimated as µg of GA equivalent per gram of extract. The absorbance at 720 nm was used to analyze the EtOA fractions (876, 637, 345, and 162). The n-BuOH and water extracts showed low concentrations of phenolic compounds. Currently, the TCP (%) was calculated only for the EtOA fraction, along with four concentrations in the range of 40.7-87%. These results revealed that the EtOA fraction from F.karelinii has a significant phenolic component.
In the study, the free radical scavenging ability of diverse phenolic compounds was estimated using FC, DPPH, and FRAP assays. To obtain a calibration curve for each assay, eight standards (gallic and ascorbic acid) concentrations were used. The calibration curve for each assay had a linear fit, and the 95% confidence band is shown in Figure . The corresponding slope, intercept, and R2 for each method are given in Table .
Table 4. Results from linear curve fitting of the FC DPPH, FRAP assays. For slope and intercept, mean ± SD are given.
In order to assess the antioxidant activity of a substance, it is crucial to understand the structural requirements of its phenolic constituents. Therefore, the correlation between TPC and antioxidant activity, including FC, DPPH, and FRAP assays being estimated. Few studies on the antioxidant activity of extracts from Fritillaria species were found. According to Sevim et al. (Citation2011), out of the 41 Fritillaria species that grow in Turkey, only less than 25% showed antioxidant activity in their water-alcohol extract. Li et al. (Citation2010) tested the crude non-alkaloid fraction for the invitro antioxidant test.
Data Table shows a linear relationship between the standards and EtOA fractions concentrations observed at concentrations ranging 0.4–1.0. for the neutralization of free radicals by DPPH and FRAP methods. There was a strong correlation between TPC and % inhibition from linear curve fitting of the FC, DPPH, and FRAP assays for the EtOA fractions (Figure ).
Figure 6. Correlation between TPC content and DPPH, FRAP.
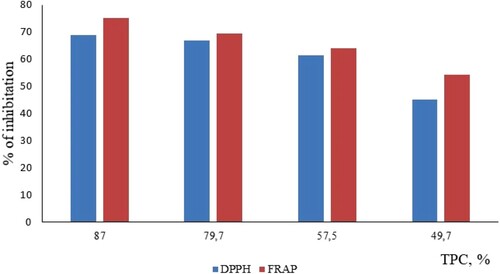
Table 5. Assay values of different EtOA fractions from F.karellinii bulbs on FC, DPPH and FRAP scavenging activity.
The IR spectra of the analyzed fractions (EtOA and n-BuOH) showed broad peaks at 3450–2850 cm−1 (phenolic O-H stretching) and 1750–1650cm−1 (double bond of C = O group). The presence of carbohydrates is evidenced by absorption bands of-CH2-groups at 2931 cm−1 and 1620–1470cm−1 (aromatic C–H). There were also characteristic peaks observed at 1750–1200 cm−1, where the stretching vibrations corresponding to C – O – C in aromatic and unsaturated esters were observed in the region 1270-1200 cm−1 and 1041 (stretching vibration – C – OH), 1516 (aromatic C = O) (Kazicyna et al. Citation1971; Heneczkowski et al. Citation2001).
Discussion (Comparison of cpDNA and nrITS matrices)
First, samples obtained from the nrITS, rbcL, and trnH-psbA regions were identified as Fritillaria karelinii using NCBI BLAST. In the phylogenetic trees (nrITS and plastid rbcL) F. karelinii formed a monophyletic clade with F. gibbosa and F. stenanthera. A comparison of trnH-psbA data requires more sequences. In each phylogenetic sister species F. karelinii was previously treated as belonging to the genus Rhinopetalum (Flora of Kazakhstan Citation1958). It is currently treated as a subgenus Rhinopetalum with F. karelinii (Rix Citation2001).
The DNA barcoding results in this study could not distinguish F. karelinii from those from Kazakhstan, Uzbekistan, and China. Despite some sequence differences found in the nrITS region between the Kazakhstani and Chinese species (C1, C3, C5), but no specific sequence existed (Figure ). For the other markers (rbcL and trnH-psbA), there were no significant differences between the Chinese species (Figures and ). The distribution area of F. karelinii appears to be widespread in Central Asia and Western China, including Xinjiang.
Several molecular studies of the genus Fritillaria, including F. karelinii have been conducted in the past and demonstrated the generic delimitation of Fritillaria and Lilium using DNA barcoding markers (matK, rpl16, and nrITS) F. karelinii formed a subgenus Rhinopetalum clade with F. gibbosa (Rønsted et al. Citation2005). Other studies have solved the limitations of phylogenetic studies using DNA barcoding markers, such as low resolution, with phylogenomic studies using complete Fritillaria chloroplast genomes. In addition, regions with high mutation rates between Fritillaria species were compared, suggesting the presence of Fritillaria-specific candidate marker regions (Bi et al. Citation2018; Li et al. Citation2018).
Conclusion
The value of this study is that, for the first time, the nucleotide sequences of Fritillaria karelinii distributed in Kazakhstan were analyzed to confirm its genetic identification and phylogenetic position. According to the results, the BLAST results matched at a high rate of 98%, and a single clade was formed in the phylogenetic analysis. This confirmed a clear genetic identification and taxonomic position.
F. karelinii has been extensively used in China and Kazakhstan as an important medicinal plant and is currently endangered due to reckless overharvesting. DNA barcoding was performed to establish the information necessary for the identification of the endangered species of F. karelinii which is native to Kazakhstan and for the conservation of the species. For the future conservation and utilization of F. karelinii in Kazakhstan, research on the development of markers that can be distinguished from F. karelinii populations in other locations is needed.
Primary data on the antioxidant activity of EtOH aerial and bulbs and EtOA fractions (at four different concentrations) have shown potential activity as a source of antioxidants.
Acknowledgments
The authors thank Professor Friesen N. (Osnabrück Botanical Garden, Germany) for reviewing and providing valuable comments in the DNA barcoding section.
Author contribution statement
Conceptualization and design of the study was made by N.G., and Z.K.; N.G. and A.T. carried out the collection field data, preparation plant samples, submitted specimens; S.J. and J-H.P. performed the experiments and analyzed the DNA barcoding data, registration in NCBI; A.S. and G.M. carried out the antioxidant experiments; Z.K. conceived of the presented data, care out the laboratory extraction and preparation fractions, IR spectrum and antioxidant activity test, data collection and its interpretation. N.G., S.J. and J-H.P. contributed to the interpretation of the results. Z.K. took the lead in writing the manuscript. All authors agree to be accountable for all stage of this study.
Supplemental Figures and Tables
Download Zip (26.7 KB)Disclosure statement
No potential conflict of interest was reported by the author(s).
Data availability statement
The nucleotide sequence data are available in the GenBank database of the National Center for Biotechnology Information (NCBI) at https://www.ncbi.nlm.nih.gov/ (see the codes in Table ) and the experimental data supporting the findings of this study are in the figshare repository and can be accessed using the following link: https://doi.org/10.17632/mv9xf2jk84.2.
Additional information
Funding
References
- Abbaszadeh S, Teimouri H, Farzan B. 2019. An ethnobotanical study of medicinal plants with antianxiety and antidepressant effects in Shahrekord. Egypt J Vet Sci. 50(1):81–87. doi:10.21608/ejvs.2019.12612.1077.
- Baitenov MS. 2001. Flora of Kazakhstan. generic complex of flora. Gylym: Almaty; p. 48–49.
- Baytulin IO. 2014. Kazakhstan Red book, 2nd ed. Astana: «ArtPrintXX1»; p. 349.
- Benzie IFF, Devaki M. 2018. The ferric reducing/antioxidant power (FRAP) assay for non-enzymatic antioxidant capacity: concepts, procedures, limitations and applications.
- Bi Y, Zhang MF, Xue Jing Dong Ran Du Yun-peng 2018. Chloroplast genomic resources for phylogeny and DNA barcoding: a case study on Fritillaria. Sci Rep. 8(1):1184. doi:10.1038/s41598-018-19591-9.
- Chen Q, Hu H, Zhang D. 2022. DNA barcoding and phylogenomic analysis of the genus fritillaria in China based on complete chloroplast genomes. Front Plant Sci. 13:764255. doi:10.3389/fpls.2022.764255.
- Chinese Pharmacopoeia Commission. 2015. Pharmacopoeia of the people’s republic of China version. Beijing: China Medical Science Press; p. 349.
- Dariba D, Taboada GL, Doallo R. 2012. Jmodeltest 2: more models, new heuristics and parallel computing. Nat Methods. 9:772. doi:10.1038/nmeth.2109.
- Day PD, Madeleine B, Laurence H, Berger M, Hill L, Fay MF, Leitch AR, Leitch IJ, Kelly LJ. 2014. Evolutionary relationships in the medicinally important genus Fritillaria L. (Liliaceae). Mol Phylogenet Evol. 80:11–19. doi:10.1016/j.ympev.2014.07.024.
- Denisenko TA, Vishnikin AB, Tsiganok LP. 2015. Spectrophotometric determination of phenolic compounds sum in plants using aluminum chloride, 18-molybdodiphosphate and folin-ciocalteu reagents. Аналитика и контроль. 19:373–380. doi:10.15826/analitika.2015.19.4.012.
- Fay MF, Swensen SM, Chase MW. 1997. Taxonomic affinities of Medusagyne oppositifolia (Medusagynaceae). Kew Bull. 52:111–120. doi:10.2307/4117844.
- Felsenstein J. 1985. Confidence limits on phylogenies: an approach using the bootstrap. Evolution. 39:783–791. doi:10.2307/2408678. accessed 11.12. 2023.
- Flora of Kazakhstan. 1958. ed. Pavlov N.V.; Science Academy of KazSSR; Alma-Ata, USSR. Т. 2; pp. 195.
- Fritillaria karelinii. URL:https://powo.science.kew.org/results?q=Fritillaria%20karelinii [accessed 2023 May 15].
- Hao D-C, Gu X-J, XIAO P-G, PENG Y. 2013. Phytochemical and biological research of fritillaria medicine resources. Chin J Nat Med. 11:330–344. doi:10.1016/S1875-5364(13)60050-3.
- Heneczkowski M, Kopacz M, Nowak D, Kuźniar A. 2001. Infrared spectrum analysis of some flavonoids. Acta Poloniae Pharmaceutica –Drug Research. 58(6):415–420.
- Hill L. T Fritillaria: A List of Published Names. http://www.fritillariaicones.com/info/names/frit.names.pdfV7.9.
- Ivaschenko A. 2005. Tulips and other bulbs plants of Kazakhstan. Publisher “Dve stolicy”: Almaty; pp. 192.
- Ivashchenko AA. 2008. Flowering plants of the south-east of Kazakhstan. Field specificity of the most common species. Almaty: Biodiversity Association of Kazakhstan; pp. 184.
- Ivashchenko AA, Grudzinskaya LM, Tursynbay A, Gemejiyeva NG. 2023. The experience of the primary introduction of Fritillaria karelinii in the main botanical garden of almaty. Problems of Botany of South Siberie and Mongolia. 22:166–172.
- Katoh K, Standley DM. 2013. Mafft multiple sequence alignment software version 7: improvements in performance and usability. Mol Biol Evol. 30(4):772–780. doi:10.1093/molbev/mst010.
- Kazicyna LA, Kupletskaya NB. 1971. UV-, IR- and NMR spectrometry application in organic chemistry. 2nd Edition. Moscow, USSR: Vysshaya shkola. 264 pp..
- Khan N, Friesen N, Sultan A, Fritsch RM, Ishaq K. 2022. Allium sulaimanicum: A new allium species and section from Pakistan. Front Plant Sci. 13:1020440. doi:10.3389/fpls.2022.1020440.
- Kluge AG, Farris JS. 1969. Quantitative phyletics and the evolution of anurans. Syst Zool. 18:l–1. doi:10.2307/2412407.
- Kress WH, Wurdack KJ, Zimmer EA, Weigt LA, Janzen DH. 2005. Use of DNA barcodes to identify flowering plants. Proc Natl Acad Sci USA. 102:8369–8374. doi:10.1073/pnas.0503123102.
- Li X, Gao W, et al. 2010. In vitro antioxidant and in vivo antiinflomatory potential of crude non-alkaloid fractions from Fritillaria usseriensis Maxim. Am. J. Pharm. 29:1328–1335.
- Li Y, Zhang Z, Yang J, Lv G. 2018. Complete chloroplast genome of seven fritillaria species, variable DNA markers identification and phylogenetic relationships within the genus. PLoS One. 13(3):e0194613. doi:10.1371/journal.pone.0194613.
- Nile SH, Su J, Wu D, Wang L, Hu J, Sieniawska E, Kai G. 2021. Fritillaria thunbergii Miq. (Zhe Beimu): A review on its traditional uses, phytochemical profile and pharmacological properties. Food Chem Toxicol. 112289. doi:10.1016/j.fct.2021.112289.
- Rashid I, Yaqoob U. 2021. Traditional uses, phytochemistry and pharmacology of genus Fritillaria—a review. Bullet Nat Res Centre. 45:124. doi:10.1186/s42269-021-00577-z.
- Reis SF, Rai DK, Abu-Ghannam Nissreen. 2012. Water at room temperature as a solvent for the extraction of apple pomace phenolic compounds. Food Chem. 135:1991. doi:10.1016/j.foodchem.2012.06.068.
- Rix M. 2001. Fritillaria: A revised classification: together with updated list species. Fritillaria Group of the Alpine Garden Society. 14.
- Ronquist R, Huelsenbeck JP. 2003. Mrbayes 3: Bayesian phylogenetic inference under mixed models. Bioinformatics. 19:1572–1574. doi:10.1093/bioinformatics/btg180.
- Rønsted N, Law S, Thornton H, Abu-Ghannam N. 2005. Molecular phylogenetic evidence for the monophyly of Fritillaria and Lilium (Liliaceae; Liliales) and the infrageneric classification of fritillaria. Mol Phylogenet Evol. 35(3):509–527. doi:10.1016/j.ympev.2004.12.023.
- Sevim D, Senol FS, Orhan I Şener B Kaya E Rastgeldi U Kesici A Aslay M 2011. Studies on anticholinesterase and DPPH radical scavenging effects of 41 species of Fritillaria L. genus of Turkish origin. Planta Med. 77:1229–1472. doi:10.1055/s-0031-1282922.
- Shaheen H, Qureshi R, Akram A Gulfraz M 2014. Inventory of medicinal flora from Thal desert, Punjab, Pakistan. Afric J Tradition Complement Alternat Med. 11:282–290. doi:10.4314/ajtcam.v11i3.39.
- Skvortsov AK. 1977. Herbarium. Methodology and technique manual. Moscow: Nauka; pp. 199.
- Swofford D–L. 2004. PAUP*: phylogenetic analysis using parsimony (* and other methods). Version 4. Sunderland, Massachusetts: Sinauer Associates.
- Tursynbay A, Gemejieva NG. 2020. Identification of distribution features of Fritillaria karelinii (Fisch. еx D. Don) Baker on the south east of Kazakhstan. Bulletin of Karagandy University. Series «Biology. Medicine. Geography». 4(100):85–93. doi 10.31489/2020BMG4/85-93.
- White TJ, Bruns TD. 1990. Amplification and direct sequencing of fungal ribosomal RNA Genes for phylogenetics. PCR Protocols: A Guide to Methods and Amplifications, pp. 315–322.
- Xiang L, Su Y, Li X, Xue G, Wang Q, Shi J, Wang L, Chen S. 2016. Identification of Fritillariae bulbus from adulterants using ITS2 regions. Plant Gene. 7:42–49. doi:10.1016/j.plgene.2016.05.001.
- Yun YG, Jeon BH, Lee J, Lee S, Lee H, Jung K, Jun-Lee J, Lee S, Kim E. 2008. Verticinone induces cell cycle arrest and apoptosis in immortalized and malignant human oral keratinocytes. Phytother Res. 22(3):416–423. doi:10.1002/ptr.2345.
- Zhang T, Huang S, Song S, Zou M, Yang T, Wang W, Zhou J, Liao H. 2021. Identification of evolutionary relationships and DNA markers in the medicinally important genusFritillariabased on chloroplast genomics. PeerJ. doi:10.7717/peerj.12612.