Abstract
Ultraviolet (UV) has a significant impact on the health and development of plants. Depending on the wavelength, UV is divided into three regions according to their wavelengths: UVC (less than 280 nm), UVB (280-315 nm), and UVA (315-400 nm). Although high UV levels have been shown to harm plant growth, low doses of UV stimulate the synthesis and buildup of healthy compounds that absorb UV, and it has positive effects on the nutritional value and productivity of various fruits, ornamental crops, and vegetables. However, there is little recent data on the use of UV radiation to improve the quality of agricultural production. This review discusses various UVA, UVB, and UVC radiation applications for pre- and post-harvest agricultural production improvement. Both UVA and UVB boost plant resilience to environmental pressures by increasing their antioxidant power through the manufacturing of secondary metabolites like flavonoids and phenolics. UVC radiation has strong germicidal qualities that improve the quality of fruits and the shelf life of fresh foods, which is something that consumers who prefer healthy, organic food urgently need right now. This review offers essential knowledge and methods for using UV radiation as a safe tool to increase global food security.
Introduction
UV (ultraviolet) is a region of the light spectrum that is divided into three regions according to their wavelengths: UVC (less than 280 nm), UVB (280-315 nm), and UVA (315-400 nm). Long-UVA (350-400 nm) and short-UVA (315-350 nm) are additional categories for the UVA regions (Rai et al. Citation2021). UVB radiation is known to have a significant impact on plants and animals despite having the lowest percentage in sunlight (less than 0.5% of the total energy reaching the surface). Higher UVB exposures cause physiological stress that includes DNA deterioration and damage, cellular proliferation inhibition, and the production of reactive oxygen species (ROS) (Heijde and Ulm Citation2012; Mmbando et al. Citation2020). UVC can also harm DNA, impair the function of mitochondria and chloroplasts, and trigger stress responses in plants (Saxena et al. Citation2011; Otake et al. Citation2021). However, low doses of UVB radiation cause photomorphogenic changes, such as the biosynthesis of anthocyanins and flavonoids, inhibition of hypocotyl growth and stomatal opening, and cotyledon expansion (Jenkins Citation2017; Clayton et al. Citation2018; Stockenhuber et al. Citation2024). At low doses, it activates the UVR8 photoreceptor and alters the expression of genes involved in UVB stress adaptation (Podolec et al. Citation2021; Fang et al. Citation2022).
Photoreceptors, such as UV Resistance Locus 8 (UVR8), which enables acclimation to UVB stress (Loconsole Citation2021), and Zeitlupe, cryptochromes, and phototropin proteins, which affect UVA (Heijde and Ulm Citation2012), regulate the relationship between plants and UV light. Studies have shown the effects of 1.2 W m −2 of UVB radiation on reducing growth inhibition of economically significant crops like rice using growth chamber experiments (Hidema and Kumagai Citation2006; Mmbando et al. Citation2020, Citation2021). On the other hand, Mmbando et al. recently showed the advantages of UVB radiation in improving the resistance mechanism to the rice blast fungus Magnaporthe oryzae at a lower dose of UVB radiation (0.4 W m−2) (Mmbando et al. Citation2023). Therefore, at the right dosage, UV radiation could be used as a non-toxic method to enhance the flavor, flavonoid content, and biotic and abiotic resistance of many agricultural products. Recent research has suggested that low, ecologically-relevant UVB levels have regulatory properties that activate specific changes in the plant's secondary metabolism, leading to the accumulation of secondary metabolites in the plant (Schreiner et al. Citation2016; Escobar-Bravo et al. Citation2017; Vàsquez et al. Citation2020; Volkova et al. Citation2022). UV radiation influences the quality of postharvest fruits by preventing fruit senescence (Duarte-Sierra et al. Citation2019; Darré et al. Citation2022; Ortiz-Araque et al. Citation2022). By serving as antioxidants and UV shielding agents, flavonoids and related phenolic compounds are widely recognized as being essential for protecting plants from UVB stress (Del Valle et al. Citation2020; Martínez-Silvestre et al. Citation2022; Cun et al. Citation2024). Flavonoid biosynthesis, which can be influenced by visible radiation (400-700 nm), UVA, and UVB (Siipola et al. Citation2015), is at least partially mediated by the UVR8 photoreceptor (Clayton et al. Citation2018; Podolec et al. Citation2021). Furthermore, the impact of UVC on plants may result from the expression of transcription factors that control gene expression (Martins et al. Citation2022), the accumulation of secondary metabolites like anthocyanins and flavonoids (Apelt et al. Citation2019; Abbasi et al. Citation2021), activation of antioxidant enzymes (Kamel et al. Citation2022), and changes in the hormone profile of plants (Kondo et al. Citation2011).
The production of high-quality, risk-free food products has continued to be the biggest challenge for the food industry (Fung et al. Citation2018). Fresh fruits will experience significant losses due to shelf life, mechanical damage, and disease even though they are essential parts of the human diet due to their abundance in minerals, vitamins, sugar, acid, and pigments (Peng et al. Citation2022). According to study by Stevens et al., these losses account for 30% of the harvested yield (Stevens et al. Citation1997). Due to their propensity to sustain damage to fruit tissue during transportation and storage, fruits have low disease resistance and are highly susceptible to microbial infection (Peng et al. Citation2022). The majority of convection methods investigated and used in the food industry to protect agricultural products use ultrasound, pulsed electric fields, high-pressure processing, cold plasma processing, thermal processing, and pasteurization (Mújica-Paz et al. Citation2011; Soni et al. Citation2016; Daher et al. Citation2017; Ekezie et al. Citation2017). However, some of these methods harm nutrition (oxidation of lipids, loss of vitamins, denaturation of lipids, etc.) and negatively alter the physical characteristics of foods (texture, odor, quality, etc.), which harms their quality. Some may rely on the use of chemicals that are harmful to our health or the environment (Singh et al. Citation2020). Finding non-chemical methods such as UV radiation as an alternative to chemical protection for fruits and other agricultural products is crucial because the majority of preharvest factors, such as disease, will result in many postharvest quality losses. The recent use of UV radiation to improve the quality of agricultural products before and after harvest, however, is little known.
The primary focus of this review is on the most recent research on the relationship between UV radiation and the improvement of agricultural product quality. This article discusses the processes by which UV radiation enhances the quality of pre- and post-harvested agricultural products, such as fruits, by altering factors like leaf morphology, sugar content, and ripening (Figure ). Given that UV is free energy derived from sunlight, the information provided here will help identify strategies for creating secure and economical ways to enhance the quality of agricultural products.
Figure 1. The possible beneficial effects of using hormesis ultraviolet radiation in vegetable and fruits. Hormesis UV radiation levels may alter plant chemistry by boosting antioxidant capacity, lengthening shelf life, altering fruit sugar content and ripening, and enhancing resistance to pests and diseases, all of which ultimately improve the quality and yield of agricultural products.
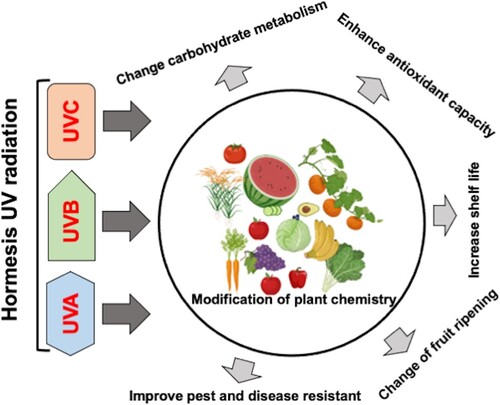
Technological hurdles need to be overcome before exploiting the preharvest benefit of UV radiation
Although the beneficial effects of UV radiation on plant health and nutritional content are well established, there are numerous difficulties in translating these findings into real-world applications. The precise control of UV dosage to guarantee that plants receive the best exposure possible without suffering damage is one of the main issues (Meyer et al. Citation2021). This balance requires sophisticated UV delivery systems with precise control. Integration with current agricultural practices also presents a challenge. It's important to carefully consider logistical and operational aspects when designing UV radiation strategies that seamlessly integrate with a variety of farming techniques. Furthermore, for consistent results, methods must be developed to apply UV radiation consistently across a range of crop types and growth stages (Leszczynski Citation1995; Meyer et al. Citation2021; Palma et al. Citation2021). An additional challenge is the creation of affordable and effective UV radiation technologies. This includes looking into ways to scale up UV radiation applications without using too many resources. Moreover, it is necessary to improve monitoring methods that accurately determine UV dosage and its impact on crops. There is currently a lack of thorough research addressing these issues. Closing these gaps will make it easier to use UV radiation as a practical tool for boosting preharvest agricultural results, which will eventually contribute to better crop quality, longer shelf life, and increased food security.
Research done on the preharvest benefits of UV radiation
Preharvest UVB treatment has been demonstrated to help fruit growth, ripening, coloration, and sugar accumulation quickly (Li and Yamane Citation2021). Pre-harvest UVB could be used as a tool to increase the antioxidant potential and content of polyphenols in basil-stored leaves without harming their visual quality (Beatriz et al. Citation2020). UVB influences anthocyanin accumulation by altering upstream transcriptional regulation (Li et al. Citation2021b). Moreover, the basil leaves’ total flavonoid and total phenol contents, as well as the production of some specific compounds (like luteolin and cinnamic acid), have been reported to increase with the long-term discontinuous application of UVB radiation (Ghasemzadeh et al. Citation2016; Mosadegh et al. Citation2018). Blueberry (Vaccinium corymbosum L.) ripening and fruit coloration have been shown to increase with preharvest long-term UVB exposure, suggesting that the additional fruit coloration brought on by UVB exposure was caused by the direct effects of UVB on anthocyanin metabolism (Li et al. Citation2021b). Consequently, exposure to low doses of UVB radiation can also improve the uniformity of berry ripening within a cluster (Li et al. Citation2021b). Likewise UVB treatments have been shown to affect anthocyanin accumulation through upstream transcriptional regulation, in contrast to anthocyanin synthesis and accumulation that occurs naturally in mature fruits (Peng et al. Citation2022). According to these studies, blueberry fruit development can be controlled by preharvest UVB exposure. Furthermore, UVC preharvest treatment is an eco-friendly method of reducing plant pathogens and improving crop quality because of its bactericidal effects and capacity for assisting plants to defend themselves (Erkan et al. Citation2008; Urban et al. Citation2016; Zhao et al. Citation2021). For instance, Janisiewicz et al. showed that the UVC treatment applied before harvesting is an efficient way to manage Botrytis cinerea in strawberry production (Janisiewicz et al. Citation2016). Besides, UVC radiation has been demonstrated to have a significant impact on the germination of B. cinerea conidia as well as to significantly slow down the growth of mycelia (Darras et al. Citation2012). Preharvest UVC treatment has also been shown to reduce the microbial biomass and incidence rate of fruit surface in the greenhouse while increasing the accumulation of pathogenesis-related protein 1 (PR-1) and B-1,3-glucanase (de Oliveira et al. Citation2016). Future studies should examine the effects of preharvest UV light dosage on fruit quality even though the agricultural practice of using artificial UV light can improve the nutritional quality and sensory experience of fruits.
Research done on the postharvest benefits of UV radiation
UVB radiation may have an impact on plant performance, but it may also have an impact on herbivore and antagonist performance, behavior, and survival (Kuhlmann and Müller Citation2011). The beneficial effects of UV to activate plant resistance must be used with caution because some of the activated specialized metabolites that ward off insects and microorganisms may also be unpalatable to humans, such as a bitter taste (Vanhaelewyn et al. Citation2020). While high UVB exposure can lead to physiological stress, low UVB exposure can promote photomorphogenic alteration, including inhibition of hypocotyl growth, production of flavonoids and anthocyanins, stomata opening, and cotyledon expansion (Ulm and Nagy Citation2005; Jenkins, Citation2009; Rizzini et al. Citation2011). E3 ubiquitin ligase, constitutively photomorphogenic 1 (COP1), and UVR8 are all known to interact with one another and to be involved in the photomorphogenic response (Favory et al. Citation2009). Although excessive UVB can harm plants, several authors have demonstrated that UVB has a stimulatory effect on growth at UVB doses as low as 0.86 μmol·m−2·s−1 (Sun et al. Citation2006) and as high as 4.15 μmol·m−2·s−1 (Hou and Ben Citation1999; Sun et al. Citation2006). Furthermore, Liu et al.'s research showed that the UVB intensity of 3.33 μmol·m−2·s−1 increased the soluble sugar content (22.7–56.7%), catalase activities (27.4–36.4%), peroxidase activities (34.1–50.4%), stem diameter growth (13.6–22.3%), and total ascorbic acid (16.9–23.2%) in cucumber leaves without affecting the net photosynthesis rate (Liu et al. Citation2017). Studies have shown that UVB stress can increase the number of secondary metabolites that are important for human health, including phenol (Wang et al. Citation2017). UVB signaling can also increase the amount of phenol. For instance, it has been proven that mung bean sprouts (Vigna radiata) have a significantly higher nutritional value due to the high accumulation of flavonoids, phenols, and vitamin C after UVB exposure (Wang et al. Citation2017). Due to the low UVB radiation exposure, mung bean sprouts produced more vitamin C, phenols, and flavonoids (Wang et al. Citation2017).
Studies have shown that plants exposed to UVB radiation are more resistant to microbial pathogens and herbivorous insects (Kunz et al. Citation2008; Demkura and Ballaré Citation2012; Qi et al. Citation2018). Additionally, it has been proposed that UVB radiation triggers the development of flavonoid buds, which is controlled by UVR8, activating an indirect defense mechanism against insects (Jenkins, Citation2009). According to Jansen et al. UVB could foster the synthesis of particular antioxidant molecules that act as UVB protective agents (Jansen et al. Citation2008). Studies have shown that subjecting lemons to UVB treatment increased the antifungal activity of flavedo extracts against Penicillium digitatum, and that the increased resistance triggered by UVB was connected to the enhancement of phenolic substances in the peel cells (Ruiz et al. Citation2017). Therefore, the treatment of UVB dose dependence may result in the emergence of plant species with improved stress tolerance. Based on its capacity to increase antioxidant activity, such as in tomatoes (Liu et al. Citation2011), UVB radiation may be considered a contemporary, chemical-free, and sustainable method of reducing the poor quality of post-harvest storage.
Postharvest decay brought on by microorganisms like Alternaria alternata (Fr.: Fr.) Keissl., Botrytis cinerea (Pers.: Fr), and Rhizopus stolonifer (Ehrenb.: Fr.) are a major concern during the handling and distribution of tomatoes (Stevens et al. Citation2004; Charles et al. Citation2009; Obande et al. Citation2011). The effects of UV irradiation on microbial growth and decay in tomato fruits are still being studied (Mditshwa et al. Citation2017). For instance, Obande et al. reported that postharvest UVC treatment at a dosage of 8 kJ m−2 inhibited Penicillium digitatum growth during a 16-day storage period at 16°C (Obande et al. Citation2011). Furthermore, Mukhopadhyay et al. showed that UV treatment at a dosage of 0.60–0.60 kJ m−2 successfully hindered Salmonella enterica and Escherichia coli using tomatoes stored for 25 days at 5°C (Mukhopadhyay et al. Citation2014). Additionally, ‘Trust’ tomatoes that had been exposed to UVC were found to be more resistant to gray mold decay than untreated fruit (Charles et al. Citation2008b). It has been suggested that these disease resistance mechanisms in UVC-treated tomatoes are related to PR proteins with UV-C-activated B-1,3-glucanase and glucanohydrolase activities (Charles et al. Citation2009). According to these studies, UV radiation can be used as a postharvest procedure that is both effective and environmentally friendly to guarantee the general quality and safety of foods like tomatoes. However, little is known about the most recent ways in which UV radiation to enhance horticulture crops. The following are some current advantages of UV radiation for enhancing agricultural products:
Different advantages of UV radiation for plant chemistry and architecture
The impact of UV radiation on the metabolism of carbohydrates, protein, and lipid
Fructose and soluble sugar glucose were shown by Piccin et al. to be more responsible for UVB treatment. UVB-treated Olivastra seggianese plants accumulated less glucose, especially after the second week, which is likely due to a reduction in photosynthetic activity and greater use of this sugar to maintain cellular respiration (Piccini et al. Citation2020) (Table ). Furthermore, it has been demonstrated that the UVB condition increases fructose accumulation, except for at T6, an O. seggianese variety (Piccini et al. Citation2020). According to Rosa et al., the increase in fructose may be the result of stress-induced sucrose degradation or the availability of the substrate for the synthesis of secondary metabolites (such as phenolic and lignin compounds) (Rosa et al. Citation2009). Dias et al. found that olive plants (Olea europaea L) exposed to a low dose of UVB (12 KJ m−2 d−1) produced less sucrose and starch but supported glucose and sorbitol contents (Dias et al. Citation2018) (Table ). A lower concentration of fructose and glucose was found after UVC pretreatment, which may be related to the upregulation of genes involved in sucrose synthesis. UVC pretreatment has been shown to significantly increase sucrose accumulation between days 2 and 8 of storage (Zhou et al. Citation2020). These studies suggested that the concentration of sugar in those crops was significantly impacted by the UVB pretreatment effects on the activities of sucrose metabolism enzymes (Zhou et al. Citation2020). The effects of UV radiation on these sugar components in various fruits could be used as an alternative method to enhance the quality of peaches (Prunus persica L. Beinong2 × 60–24–7) during their shelf life because sugar accumulation and metabolism are essential to fruit quality (Zhou et al. Citation2020).
Table 1. There are different impacts of UVA, UVB, and UVC on agricultural productivity. UV radiation affects many aspects of agriculture, including pre- and post-harvest modification, production of secondary metabolites, delayed ripening, reduced disease and pests, and increased shelf life.
The sweetness, acidity, firmness, nutrients, and aroma of peaches were all inherent qualities and were primarily attributed to the sugar composition and the sugar-to-acid ratio (Xu et al. Citation2022). Given that fructose is 1.7 times sweeter than sucrose (Kroger et al. Citation2006) and is impacted by UVB treatment (Piccini et al. Citation2020), the application of artificial UVB treatment could increase the quality and shelf life of fruits. Rich sugars serve as essential substrates for a variety of secondary metabolites that give plants strong resistance to a variety of stresses in postharvest fruits (Hu et al. Citation2019). Recent research has demonstrated that UVC treatment significantly increased the up-regulation of the phosphoenolpyruvate carboxykinase (PEPCK) gene and the nicotinamide dinucleotide phosphate-malic enzyme (NADP-ME enzyme, which improved the ratio of total soluble sugars to a total organic acid (Onik et al. Citation2019)). To produce agricultural products of the highest caliber, it is crucial to design the proper UV dosage. The quality, shelf life, and flavor of postharvest fruit could then be enhanced by modifying UV dosage. Future studies must, however, establish the proper UV dosages that affect carbohydrate mechanisms without stressing crops.
Excessive UV radiation can cause plant cells to produce ROS, which can result in oxidative stress. Stress causes modifications in protein metabolism, which include the breakdown of defective proteins and an elevation of PR protein and antioxidant enzymes (Vanhaelewyn et al. Citation2020; Abbasi et al. Citation2021). Furthermore, UV radiation can change how genes related to protein synthesis and degradation pathways are expressed, which can affect the rate at which proteins turnover occurs (Charles et al. Citation2009; de Oliveira et al. Citation2016; Mmbando et al. Citation2023). In addition, the synthesis of UV-absorbing proteins like proline and flavonoids, which serve as defense molecules against additional UV damage, can be specifically induced by UV-B radiation, changing the overall protein profile of plants (Del Valle et al. Citation2020; Dias et al. Citation2020; Laoué et al. Citation2022; Martínez-Silvestre et al. Citation2022; Cun et al. Citation2024). Furthermore, the generation of ROS causes membrane damage and lipid peroxidation (Xue et al. Citation2022). Under conditions of oxidative stress, plants produce certain lipids to counteract damage, including antioxidants and UV-absorbing substances like tocopherols, carotenoids, and malondialdehyde (Takshak and Agrawal Citation2014; Severo et al. Citation2016; Badmus et al. Citation2022a; Citation2022b). Moreover, UV radiation modifies the expression of genes related to lipid metabolism, which impacts sterol, phospholipid, and fatty acid synthesis (Robson et al. Citation2015; Piccini et al. Citation2020). Furthermore, UV-B radiation can promote the buildup of cuticular waxes, which act as a shield against UV ray damage (Solovchenko and Merzlyak Citation2003). In general, UV radiation has a major effect on the structure and functionality of plant lipids, which is essential for their ability to respond to environmental stressors.
UV radiation and fruit ripening
Through postharvest storage, UVC treatment has been demonstrated to improve the quality of early-ripening apple (Malus domestica Borkh.) fruit (Onik et al. Citation2019). According to studies (Artés et al. Citation2009; Prajapati et al. Citation2021), UVC treatment on postharvest products is effective at preventing senescence and ripening, maintaining relatively inflated bitter gourd fruit (Momordica charantia L.) firmness, producing flavonoids (such as anthocyanin), and boosting the activity of defense molecules and antioxidants (Table ). Even though it has been demonstrated that UVC treatment on apples can extend the storage life of vegetables and fresh horticultural crops (Darré et al. Citation2022), it has only occasionally been used. Although ethylene production and ACC oxidase (ACO) 1 transcriptional expression was increased, Severo et al. showed that tomato (Solanum lycopersicum L.) fruit did not ripen as quickly as expected (Severo et al. Citation2016). According to that study's findings, UVC-treated fruit's coloration development was slower than that of control fruit and exhibited the fewest signs of senescence (Severo et al. Citation2016). Similar earlier research (Liu et al. Citation2009; Tiecher et al. Citation2013) suggested that UVC treatment could slow down the ripening process and delay the appearance of red coloration in tomatoes. According to Severo et al. the interference of UVC with carotenoids, the main pigments in tomatoes, may be the cause of its effect on the development of fruit coloration (Severo et al. Citation2016). Furthermore, UVC treatment may affect other antioxidant pathways, such as the production of bioactive amines, phenolic compounds, and antioxidant enzymes (Baenas et al. Citation2021). These substances may prevent chlorophyll degradation and/or slow carotenoid degradation (Tiecher et al. Citation2013; Dyshlyuk et al. Citation2020).
According to Severo et al., the effect of UVC on the reduction in the accumulation of b-carotene and lycopene in fruit may also contribute to the lower intensity of the fruit's distinctive red color (Severo et al. Citation2016). Although the test of fresh grapes will be impacted, UV light treatment has been demonstrated to accelerate grape ripening and improve the aroma and polyphenol content of grape peel (Liu et al. Citation2018; Del-Castillo-Alonso et al. Citation2021). Moreover, preharvest exposure to UVC has also been shown to enhance the disease resistance of strawberries (Fragaria ananassa) due to the increased production of bioactive compounds (Xu et al. Citation2017) (Table ). Similar to low-dose UVB treatment before harvest, which also accelerated the ripening and coloring of blueberry fruits, it has been reported that UV light treatment preharvest sped up the ripening of grapes (Sun et al. Citation2023). Preharvest UV irradiation can also significantly increase the amount of soluble sugar in blueberry fruits after ripening (Yang et al. Citation2018). These studies suggested that preharvest UV treatment could be used to modify the color of fresh fruit by accelerating or delaying fruit ripening, depending on the dosage. Since the effects of UV light on increasing ripening are typically associated with enhanced antioxidant capacity, delayed fruit decay, decreased lipid peroxidation, improved preservation of acids and sugar, and enhanced flavonoids accumulation, among others things (Dyshlyuk et al. Citation2020; Baenas et al. Citation2021; Peng et al. Citation2022; Xue et al. Citation2022), this will be of crucial importance in the agriculture production of fresh fruit.
UV radiation and enhancement of antioxidant capacity
The synthesis of effective antioxidant dihydroxy B-ring-substituted flavonoids, such as luteolin derivatives, is enhanced by excessive light exposure or UVB radiation at the expense of other ineffective antioxidant flavonoids, such as kaempferol derivatives (Dias et al. Citation2020; Laoué et al. Citation2022). In addition to playing a crucial role as an antioxidant, flavonoid has also been demonstrated in other studies to offer photoprotection by blocking UV rays (Del Valle et al. Citation2020; Martínez-Silvestre et al. Citation2022) (Table ). For instance, Secale cereale and Centella asiatica's leaf epidermal flavonols play a crucial role in UVB shielding (Bidel et al. Citation2015; Del Valle et al. Citation2020); and pollen grains accumulate flavonoids to protect them from UVB damage and maintain their viability after anthesis (Rozema et al. Citation2001). To defend themselves against UVB radiation stress, some plant species can increase their antioxidant species as well as accumulate UV-absorbing compounds (Brosché and Strid Citation2003; Cun et al. Citation2024). For instance, it has been demonstrated that UV radiation increases the fruit ripening and antioxidant capacity and enzyme activity of highbush blueberry fruits (Li et al. Citation2021b) (Table ). The activation of the secondary metabolic pathway and antioxidant defense system is one of the significant and important reactions that grapes exhibit in response to UVB radiation (Carbonell-bejerano et al. Citation2014; Tripathi et al. Citation2021). For instance, it has been reported that short-term UV-B supplementation improves Withania coagulans ‘ antioxidative defense system, which in turn stimulates secondary metabolism and fights oxidative stress (Tripathi et al. Citation2021). Additionally, the production of antimicrobial phytoalexin was primarily responsible for the effect of UVC light on extending shelf life. Therefore, UV light exposure may be used in the post-harvest processing of fruit to extend the 2- to 3-day selling window and thereby reduce economic loss (Li et al. Citation2017). Luthria et al. showed that tomatoes’ phenolic content was boosted after exposure to UV light, especially caffeic acid, which increased to about 120% of its initial level (Luthria et al. Citation2006). In addition, it has been shown that UV radiation activates the signaling pathways of the COP1 and UVR8 photoperception proteins, leading to the accumulation of anti-UV molecules like flavonoids and tannins (Vanhaelewyn et al. Citation2020; Badmus et al. Citation2022a). The antioxidant defense is known to be activated by UVA and UVB, which shield the plant from the main harmful effects of ROS (Agarwal Citation2007; Tripathi et al. Citation2021).
Despite the precipitation regime and the season, plant exposure to UVB radiation had a positive impact on the quercitrin content of Arbutus unedo leaves. Based on some research (Di Ferdinando et al. Citation2014; Nenadis et al. Citation2015), the UVB-induced increase in quercitrin content in the leaf is related to its high antioxidant efficiency and its role in controlling auxin movement, which in turn activates the morphogenic plant response to UV stress. According to Kang et al., prolonged UVA exposure can increase the phenolic content and growth of sweet basil (Ocimum basilicum L) (Kang et al. Citation2022). The antioxidant capacity of strawberry fruits exposed to high doses of UVC light (4 kJ m−2, 33 W m−2) decreased similarly to the control group (Cote et al. Citation2013). According to Li et al., one of the potential causes of the degradation may have been the dose applied to longan and rambutan, which was higher than the hormetic dose (Li et al. Citation2017). Hormesis is thought to occur when low doses of an agent (hormetic dose) are applied, although high doses may have adverse effects (Vàsquez et al. Citation2020; Volkova et al. Citation2022).
UV radiation and extending shelf life
By reducing the microbial load, UVC technology can be used to effectively prevent foodborne illnesses and extend the shelf life of food without sacrificing its quality. According to Singh et al., UVC irradiation treatment is effective in extending the shelf life of liquid foods while lowering the associated health risks (Singh et al. Citation2020). Low doses of UV light have been demonstrated to activate the production of anti-fungal compounds in the fruits, ripening, softening retard, and reducing chilling injury (Darré et al. Citation2022). As a result, UVC irradiation has shown promise as a different method of microbial disinfection and to lengthen the shelf life of strawberries (Idzwana et al. Citation2020) (Table ). It has been demonstrated that UVC radiation, at a suitable dose, combined with a low storage temperature (10 °C) is an effective way to maintain tomatoes’ postharvest life without negatively affecting quality indicators (Pinheiro et al. Citation2015). UVC has the potential of extending the shelf-life of several economically important vegetables and fruits such as fresh-cut pineapples (Manzocco et al. Citation2016), fresh-cut melon (Manzocco et al. Citation2011), strawberries and blueberries (Sánchez et al. Citation2021; Ortiz-Araque et al. Citation2022), mushrooms (Nunes et al. Citation2024), fresh cut carrot (Li et al. Citation2021a), broccoli (Duarte-Sierra et al. Citation2019), etc. For example, the postharvest appearance of white shimeji mushrooms (Hypsizygus tessellatus) was successfully preserved with a dose of 2 kJ m−2 of UVC (Nunes et al. Citation2024) (Table ). In addition, Erkan et al. reported that strawberry shelf life was prolonged for 4–5 days after UV treatment on the fruit's surface; the UV-treated strawberries were firmer, contained more anthocyanins, had higher titratable acidity, and respired at a slower rate than the untreated strawberries (Erkan et al. Citation2001). In contrast to applying antimicrobial compounds, fruit treated with the highest doses (0.5 kJ m−2) were firmer on day 7 and this dose also improved the product's sensory quality (Idzwana et al. Citation2020). This suggests that UV technology may be another promising technology for preserving fresh crops. This increase in firmness in UVC-treated fruits has been attributed to the radiation's effect on the activity of cell wall-degrading enzymes like pectin methyl esterase 34, endo-1,4-D-glucanase, and -galactosidase, which are increased during ripening and cause cell wall softening, although this activity tends to remain in UVC-treated fruits (Idzwana et al. Citation2020). The softening of peaches (Kan et al. Citation2021), tomatoes (Dyshlyuk et al. Citation2020), and the senescence of mango (Sripong et al. Citation2015; George et al. Citation2016) and broccoli (Duarte-Sierra et al. Citation2020) have all been reported to be effectively delayed by the UVC. Therefore, UV radiation treatment could be used as a non-chemical method of enhancing firmness and postponing ripening in fresh fruits like strawberries, increasing agricultural value while postponing cell activity.
UV radiation and increased resistance to pests and diseases
Numerous scientists are paying close attention to fungi-related diseases because they can eventually result in significant economic losses, particularly when vegetables and fruits are being stored (Zhang and Jiang Citation2019). According to Charles and Arul, UV light can prevent the growth of microorganisms and sanitize fresh food without compromising the quality of the product (Charles and Arul Citation2007). In addition to encoding PR proteins, phytoalexin is induced and accumulated by UVC, which is essential for protecting fresh products from diseases (Vanhaelewyn et al. Citation2020; Abbasi et al. Citation2021). According to reports, UVC is a residue-free physical sterilization technique that has been connected to the anti-fungal effects of fruits and vegetables after harvest (Usall et al. Citation2016). For instance, UVC treatment significantly inhibited Colletotrichum gloeosporioides's mango conidial germination and sporulation (Gunasegaran et al. Citation2018). The effectiveness of disease prevention by UVC treatment has been linked to the activation of defense systems in hosts in addition to the direct inhibition of pathogens (Zhang and Jiang Citation2019). This phenomenon may be helpful because ‘hormesis,’ which is the term for low-dose (2.0-4.4 kJ m−2) UVC treatment, can be used as a suitable non-biological stressor for fruit and vegetables to activate enhanced resistance, thereby impeding fungi (Vanhaelewyn et al. Citation2020; Meyer et al. Citation2021; Darré et al. Citation2022; Volkova et al. Citation2022). For example, the treatment of tomato peel cells with 3.7 kJ m−2 UVC led to the development of more cell wall stacking zones (CWSZ), which could be seen through transmission electron microscopy. This prevented the degradation of CWSZ by fungi's cell wall-degrading enzymes, which in turn prevented the colonization of the tomato peel cells by fungi (Charles et al. Citation2008a). Additionally, it has been suggested that CWSZ, which creates long-term resistance, is associated with increased lignin and phenolic compounds that are influenced by UVC (Del Valle et al. Citation2020). The accumulation of lignin and phenolic compounds were significantly enhanced by the UVC-impacted phenylpropanoid metabolism pathway of tomato peel cells, which increased the inhibitory intensity of CWSZ on microbial colonization (Charles et al. Citation2008b). Moreover, UVC has increased the expression of defense-related enzymes in the fruits of the tomato and Berangan banana (Musa sp. AAA Berangan) (Mohamed et al. Citation2017; Escobar-Bravo et al. Citation2019; Malek et al. Citation2021). It has recently been demonstrated using the UVC antagonist Botrytis cinerea that 2.0 kJ m−2 UVC significantly reduces the lesion diameter of strawberry (Fragaria ananassa Duch. cv. Benihoppe) fruit gray mold and increases the activity of defense enzymes like polyphenol oxidase (PPO), peroxidase (POD), and phenylalanine ammonia lyase (PAL), as well as antioxidant enzymes like superoxide dismutase (SOD) and catalase (CAT) (Jin et al. Citation2017). While UV-C irradiation may be a viable option for mitigating and reducing fungal infection, the detrimental impact on weight loss may restrict the postharvest shelf-life of irradiated blueberries (Sánchez et al. Citation2021).
Despite extensive research on UVB's effects on plants, it has proven challenging to determine whether UVB stress is ‘destructive stress’ or ‘constructive stress’ for the plant (Hideg et al. Citation2013). There aren't many studies on UVB's effects on postharvest diseases of fruits and vegetables, possibly because plants can be exposed to UVB in the wild (Zhang and Jiang Citation2019). Nevertheless, it has been demonstrated that 22 kJ m−2 of UVB treatment improves the phenylpropanoid metabolic pathway of lemon (Citrus limon (L.) Burm. F., cv. Eureka) peel cells, resulting in the development of a cell accumulation area comparable to CWSZ and subsequently increasing the resistance to Penicillium digitatum (Ruiz et al. Citation2016). Moreover, it has been demonstrated that lemons treated with UVB had increased antifungal activity in flavedo extracts resistant to Penicillium digitatum, which further suggested that the increased resistance brought on by UVB was connected to the enhancement of phenolic substances in the peel cells (Ruiz et al. Citation2017). Recently, Mmbando et al. showed that an uninoculated, highly UVB-sensitive transgenic anti-sense line (A-S) Oryza sativa ssp. japonica had improved resistance to the rice blast fungus M. oryzae. This was accompanied by an upregulation of several defense-related genes in the A-S line as compared to the UVB-resistant line (S–C line) (Mmbando et al. Citation2023). The rise in M. oryzae resistance in hosts that had not received pathogen strain inoculations led some researchers to hypothesize that the host defense mechanisms were altered by UVB effects, which were responsible for the development of the resistance in that study. The development of UVB protection mechanisms, such as the production of flavonoids, anthocyanins, and phytoalexins, which are similar to those produced during pathogen infections, may be triggered by UVB radiation (Kunz et al. Citation2006, Citation2008; Demkura and Ballaré Citation2012). Alternatively, host plants may experience UVB radiation in a similar way to how they experience actual pathogens, which trigger the activation of defense-related genes before the pathogen inoculates the plant. However, more research is required to identify ‘UVB hormesis,’ a suitable dose that can instigate defense mechanisms in host plants without harming them.
What needs to be done to utilize UV technology for horticulture in greenhouse or field conditions?
To fully comprehend how various crops react to UV radiation, extensive research is required. For this, the best UV wavelengths, intensities, and exposure times must be identified in order to maximize the positive effects and minimize any potential negative ones. In order to offer growers specific recommendations, these studies ought to cover a range of crop varieties and growth stages. Also, the creation of sophisticated UV delivery systems is crucial. These systems ought to make it possible to precisely regulate UV dosage, ensuring uniform exposure throughout the entire crop canopy. Such systems ought to integrate seamlessly with current cultivation techniques and be flexible to both greenhouse and outdoor environments. To encourage growers to embrace UV technology, education and awareness campaigns are also needed. Training programs must be developed to inform farmers about appropriate application methods, security measures, and the possible advantages of UV radiation on crop performance and yield. More farmers might adopt UV technology if concrete advantages and successful case studies were to be presented. Collaboration among scientists, agricultural extension services, and technology manufacturers is also essential. Through this collaboration, it will be easier to create UV solutions that are affordable, simple to use, and available to a variety of growers. In-depth research is required to evaluate the long-term effects of UV technology adoption on the economy and environment. Understanding how UV-assisted horticulture affects crop yields, nutrient content, and the control of pests and diseases can reveal important information about its general sustainability and viability. Another possibility is to incorporate UV technology into integrated pest management plans. By reducing the need for chemical pesticides, UV radiation can serve as a sustainable pest control method. In the future, UV technology could be combined with precision farming techniques to optimize UV application based on real-time crop conditions and UV sensor feedback.
The real practical application of UV radiation to improving horticulture crops
Targeted strategies that take advantage of UV light's beneficial effects on plant physiology and biochemistry are used in the real-world use of UV radiation to enhance horticulture crops. For example, UV lamps has been set up in greenhouse cultivation to emit particular wavelengths that promote the generation of secondary metabolites as anthocyanins in berries, improving their antioxidant activity (Li and Yamane Citation2021; Peng et al. Citation2022). This increases the crops’ nutritional value while also extending their shelf life. UV treatments can be used in the field at crucial growth stages. The accumulation of flavonoids in leaves, for instance, can increase a plant's resistance to pathogens and pests when young plants are exposed to regulated doses of UV radiation (McLay et al. Citation2020). Apples and grapes have been treated with UV technology in orchards to stimulate fruit pigmentation, improving color and increasing market value (Guerrero et al. Citation2016; Alessandrini et al. Citation2017; Li and Yamane Citation2021). UV radiation can also help with weed control. Herbicide use can be decreased by selectively using UVC light to eradicate weed seedlings without hurting established plants (Brodie Citation2018). These useful applications match consumer preferences and sustainable and organic cultivation methods (Loconsole Citation2021). However, the use of UV technology necessitates careful calibration to prevent negative effects, which emphasizes the need for research-supported recommendations and improved delivery methods.
Strategically applying UVA, UVB, and UVC radiation in agriculture has the potential to significantly increase crop quality and productivity (Heijde and Ulm Citation2012; Loconsole Citation2021). UVA radiation is essential for promoting photosynthesis, plant growth, and other physiological functions (Gao et al. Citation2021). UVA exposure may boost plant resilience to environmental pressures by affecting the manufacturing of secondary metabolites like flavonoids and phenolics, which will ultimately result in higher yields and better crop quality (Nenadis et al. Citation2015; Siipola et al. Citation2015; Kang et al. Citation2022). Furthermore, certain plant responses, such as the generation of phytochemicals like antioxidants and adaptation responses, are dependent on UVB radiation (Agati et al. Citation2013; Loconsole Citation2021). A regulated UVB exposure can promote the build-up of advantageous compounds in crops, increasing their the antioxidant power and nutritional worth (Mosadegh et al. Citation2018; Beatriz et al. Citation2020; Li and Yamane Citation2021; Li et al. Citation2021b; Peng et al. Citation2022). Additionally, UVB radiation can strengthen plant defenses against pests and diseases, making crops more resilient to them and producing better yields (Jenkins, Citation2009; Tripathi et al. Citation2021; Mmbando et al. Citation2023). Moreover, UVC radiation has strong germicidal qualities that can be used in agricultural environments for disinfection and sterilization (Pinheiro et al. Citation2015; Idzwana et al. Citation2020). UVC radiation helps keep plants hygienic by focusing on killing pathogens, pests, and microbiological contaminants. This lowers the likelihood of crop losses from diseases and increases overall productivity (George et al. Citation2016; Duarte-Sierra et al. Citation2019; Dyshlyuk et al. Citation2020; Meyer et al. Citation2021; Sánchez et al. Citation2021; Li et al. Citation2021a; Nunes et al. Citation2024). However, to avoid negative effects on plant health and guarantee the safe and efficient use of UVA, UVB, and UVC technology in agriculture, careful consideration of hormesis dosage through appropriate determination of exposure levels and timing is crucial (Vàsquez et al. Citation2020; Volkova et al. Citation2022).
Moreover, while too much UV radiation can cause stunted growth, decreased yield, and compromised plant health (Hidema and Kumagai Citation2006; Mmbando et al. Citation2020), UV radiation-induced plant stunting in agriculture has a number of advantages. For instance, stunting can result in lower plant height and compact growth, which may boost plant density per unit area and maximize yield potential by optimizing space utilization (Xiao et al. Citation2006). Furthermore, plants that are stunted may be more resilient to environmental stressors such as wind, drought, and pathogens, which would enable them to adapt better to difficult growing conditions (Ueda and Nakamura Citation2011; Mmbando and Hidema Citation2021; Mmbando et al. Citation2023). In general, the strategic utilization of UVA, UVB, and UVC radiation presents opportunities for improving agricultural techniques, raising yields, and advancing the production of food in a sustainable manner.
Conclusion
The review's main emphasis is on the recent use of UV radiation as a beneficial abiotic stress in the pre- or post-harvest enhancement of fresh fruits and vegetables. It has been established that UV radiation treatment is environmentally friendly and has enormous potential in the food industry (Singh et al. Citation2020). It necessitates low-cost equipment, the absence of residual compounds, and the avoidance of chemicals that are bad for both human health and our ecological system. Depending on the dosage, UV radiation can function through a variety of mechanisms. While low or moderate radiation with hormesis effects can increase antioxidant capacity, increase shelf life, delay ripening, and improve host plants’ resistance to pathogens, high UVB or UVC radiation can hurt plants’ growth through DNA damage, reducing their growth and yield. For UV radiation to have a positive impact on agricultural fresh products, the ideal dose with a hormesis effect must first be determined. This review summarized recent research on the effects of UV radiation on plant carbohydrates, antioxidant content, fruit ripening, and shelf life. Changes to these elements with the right amount of UV radiation may present promising non-chemical methods for enhancing the quality and security of fresh produce. Future studies should look into the practice of enhancing the nutritional value and sensory experience of fruits by exposing them to artificial UV radiation. Utilizing pre- and post-harvest UV treatment on crops will improve productivity and quality while lowering hunger and poverty.
Author contribution statement
G.S.M: Conceived, designed, interpreted the data, and wrote the paper.
Acknowledgments
The author will like to thank UDOM for providing office space for research write-ups.
Disclosure statement
No potential conflict of interest was reported by the author(s).
Data availability statement
Data sharing not applicable – no new data generated.
Additional information
Funding
References
- Abbasi BH, Khan T, Khurshid R, Nadeem M, Drouet S, Hano C. 2021. UV-C mediated accumulation of pharmacologically significant phytochemicals under light regimes in in vitro culture of Fagonia indica (L). Sci Rep. 11(1):679. doi:10.1038/s41598-020-79896-6.
- Agarwal S. 2007. Increased antioxidant activity in cassia seedlings under UV-B radiation. Biol Plant. 51:157–160. doi:10.1007/s10535-007-0030-z.
- Agati G, Brunetti C, Di Ferdinando M, Ferrini F, Pollastri S, Tattini M. 2013. Functional roles of flavonoids in photoprotection: new evidence, lessons from the past. Plant Physiol Biochem. 72:35–45. doi:10.1016/j.plaphy.2013.03.014.
- Alessandrini M, Gaiotti F, Belfiore N, Matarese F, Onofrio C. 2017. Influence of vineyard altitude on Glera grape ripening (Vitis vinifera L.): effects on aroma evolution and wine sensory profile. J. Sci. Food Agric. 97(9):2695–2705.
- Apelt A, Bavin L, Dickey E, Gruber T, Kerton B, Norzin T, Gillding ER, Worth P, Yang H. 2019. The effect of UV light intensity on anthocyanin content of richea continentis leaves. F Stud Ecol. 2:1.
- Artés F, Gómez P, Aguayo E, Escalona V, Artés-Hernández F. 2009. Sustainable sanitation techniques for keeping quality and safety of fresh-cut plant commodities. Postharvest Biol Technol. 51(3):287–296. doi:10.1016/j.postharvbio.2008.10.003.
- Badmus UO, Crestani G, Cunningham N, Havaux M, Urban O, Jansen MAK. 2022a. UV radiation induces specific changes in the carotenoid profile of arabidopsis thaliana. Biomolecules. 12(12):1879. doi:10.3390/biom12121879.
- Badmus UO, Crestani G, O’Connell RD, Cunningham N, Jansen MAK. 2022b. UV-B induced accumulation of tocopherol in arabidopsis thaliana is not dependent on individual UV photoreceptors. Plant Stress. 5:100105. doi:10.1016/j.stress.2022.100105.
- Baenas N, Iniesta C, González-Barrio R, Nuñez-Gómez V, Periago MJ, García-Alonso FJ. 2021. Post-harvest use of ultraviolet light (UV) and light emitting diode (LED) to enhance bioactive compounds in refrigerated tomatoes. Molecules. 26(7):1847. doi:10.3390/molecules26071847.
- Beatriz L, Nascimento S, Brunetti C, Agati G, Lo Iacono C, Detti C, Giordani E, Ferrini F, Gori A. 2020. The polyphenol content and antioxidant capacity of ocimum basilicum leaves during storage.: 1–19.
- Bidel LPR, Chomicki G, Bonini F, Mondolot L, Soulé J, Coumans M, La Fisca P, Baissac Y, Petit V, Loiseau A, et al. 2015. Dynamics of flavonol accumulation in leaf tissues under different UV-B regimes in centella asiatica (Apiaceae). Planta. 242:545–559. doi:10.1007/s00425-015-2291-7.
- Brodie G. 2018. The use of physics in weed control. In: Non-chemical weed control. Elsevier; p. 33–59.
- Brosché M, Strid Å. 2003. Molecular events following perception of ultraviolet-B radiation by plants. Physiol Plant. 117(1):1–10. doi:10.1034/j.1399-3054.2003.1170101.x.
- Carbonell-bejerano P, Diago M, Martínez-abaigar J, Martínez-zapater JM, Tardáguila J, Núñez-Olivera E. 2014. Solar ultraviolet radiation is necessary to enhance grapevine fruit ripening transcriptional and phenolic responses. BMC Plant Biol. 14:1–16.
- Charles MT, Arul J. 2007. UV treatment of fresh fruits and vegetables for improved quality: a status report. Stewart Postharvest Rev. 3(3):1–8.
- Charles MT, Goulet A, Arul J. 2008a. Physiological basis of UV-C induced resistance to botrytis cinerea in tomato fruit: IV. biochemical modification of structural barriers. Postharvest Biol Technol. 47(1):41–53. doi:10.1016/j.postharvbio.2007.05.019.
- Charles MT, Makhlouf J, Arul J. 2008b. Physiological basis of UV-C induced resistance to botrytis cinerea in tomato fruit: II. Modification of fruit surface and changes in fungal colonization. Postharvest Biol Technol. 47(1):21–26. doi:10.1016/j.postharvbio.2007.05.014.
- Charles MT, Tano K, Asselin A, Arul J. 2009. Physiological basis of UV-C induced resistance to botrytis cinerea in tomato fruit. V. Constitutive defence enzymes and inducible pathogenesis-related proteins. Postharvest Biol Technol. 51(3):414–424.
- Clayton WA, Albert NW, Thrimawithana AH, McGhie TK, Deroles SC, Schwinn KE, Warren BA, McLachlan ARG, Bowman JL, Jordan BR. et al. 2018. UVR8-mediated induction of flavonoid biosynthesis for UVB tolerance is conserved between the liverwort Marchantia polymorpha and flowering plants. Plant J. 96(3):503–517. doi:10.1111/tpj.14044.
- Cote S, Rodoni L, Miceli E, Concellón A, Civello PM, Vicente AR. 2013. Effect of radiation intensity on the outcome of postharvest UV-C treatments. Postharvest Biol Technol. 83:83–89. doi:10.1016/j.postharvbio.2013.03.009.
- Cun S, Zhang C, Chen J, Qian L, Sun H, Song B. 2024. Effects of UV-B radiation on pollen germination and tube growth: A global meta-analysis. Sci Total Environ. 915:170097. doi:10.1016/j.scitotenv.2024.170097.
- Daher D, Le Gourrierec S, Pérez-Lamela C. 2017. Effect of high pressure processing on the microbial inactivation in fruit preparations and other vegetable based beverages. Agriculture. 7(9):72. doi:10.3390/agriculture7090072.
- Darras AI, Demopoulos V, Tiniakou C. 2012. UV-C irradiation induces defence responses and improves vase-life of cut gerbera flowers. Postharvest Biol Technol. 64(1):168–174. doi:10.1016/j.postharvbio.2011.07.008.
- Darré M, Vicente AR, Cisneros-Zevallos L, Artés-Hernández F. 2022. Postharvest ultraviolet radiation in fruit and vegetables: applications and factors modulating its efficacy on bioactive compounds and microbial growth. Foods. 11(5):653. doi:10.3390/foods11050653.
- Del-Castillo-Alonso M-Á, Monforte L, Tomás-Las-Heras R, Martínez-Abaigar J, Núñez-Olivera E. 2021. To what extent are the effects of UV radiation on grapes conserved in the resulting wines? Plants. 10(8):1678. doi:10.3390/plants10081678.
- Del Valle JC, Buide ML, Whittall JB, Valladares F, Narbona E. 2020. UV radiation increases phenolic compound protection but decreases reproduction in Silene littorea. PLoS One. 15(6):1–18. doi:10.1371/journal.pone.0231611.
- Demkura PV, Ballaré CL. 2012. UVR8 mediates UV-B-induced arabidopsis defense responses against botrytis cinerea by controlling sinapate accumulation. Mol Plant. 5(3):642–652. doi:10.1093/mp/sss025.
- de Oliveira IR, Crizel GR, Severo J, Renard CMGC, Chaves FC, Rombaldi CV. 2016. Preharvest UV-C radiation influences physiological, biochemical, and transcriptional changes in strawberry cv. Camarosa. Plant Physiol Biochem. 108: 391–399. doi:10.1016/j.plaphy.2016.08.012.
- Dias MC, Pinto DCGA, Correia C, Moutinho-Pereira J, Oliveira H, Freitas H, Silva AMS, Santos C. 2018. UV-B radiation modulates physiology and lipophilic metabolite profile in olea europaea. J Plant Physiol. 222:39–50. doi:10.1016/j.jplph.2018.01.004.
- Dias MC, Pinto DCGA, Freitas H, Santos C, Silva AMS. 2020. The antioxidant system in olea europaea to enhanced UV-B radiation also depends on flavonoids and secoiridoids. Phytochemistry. 170:112199. doi:10.1016/j.phytochem.2019.112199.
- Di Ferdinando M, Brunetti C, Agati G, Tattini M. 2014. Multiple functions of polyphenols in plants inhabiting unfavorable Mediterranean areas. Environ Exp Bot. 103:107–116. doi:10.1016/j.envexpbot.2013.09.012.
- Duarte-Sierra A, Charles MT, Arul J. 2019. UV-C hormesis: A means of controlling diseases and delaying senescence in fresh fruits and vegetables during storage. Postharvest pathology of fresh horticultural produce. Boca Raton, FL: CRC Press. p. 539–594.
- Duarte-Sierra A, Hasan SMM, Angers P, Arul J. 2020. UV-B radiation hormesis in broccoli florets: glucosinolates and hydroxy-cinnamates are enhanced by UV-B in florets during storage. Postharvest Biol Technol. 168:111278. doi:10.1016/j.postharvbio.2020.111278.
- Dyshlyuk L, Babich O, Prosekov A, Ivanova S, Pavsky V, Chaplygina T. 2020. The effect of postharvest ultraviolet irradiation on the content of antioxidant compounds and the activity of antioxidant enzymes in tomato. Heliyon. 6:e03288. doi:10.1016/j.heliyon.2020.e03288.
- Ekezie F-GC, Sun D-W, Cheng J-H. 2017. A review on recent advances in cold plasma technology for the food industry: current applications and future trends. Trends Food Sci Technol. 69:46–58. doi:10.1016/j.tifs.2017.08.007.
- Erkan M, Wang CY, Krizek DT. 2001. UV-C irradiation reduces microbial populations and deterioration in cucurbita pepo fruit tissue. Environ Exp Bot. 45(1):1–9. doi:10.1016/S0098-8472(00)00073-3.
- Erkan M, Wang SY, Wang CY. 2008. Effect of UV treatment on antioxidant capacity, antioxidant enzyme activity and decay in strawberry fruit. Postharvest Biol Technol. 48(2):163–171. doi:10.1016/j.postharvbio.2007.09.028.
- Escobar-Bravo R, Chen G, Kim HK, Grosser K, van Dam NM, Leiss KA, Klinkhamer PGL. 2019. Ultraviolet radiation exposure time and intensity modulate tomato resistance to herbivory through activation of jasmonic acid signaling. J Exp Bot. 70(1): 315–327. doi:10.1093/jxb/ery347.
- Escobar-Bravo R, Klinkhamer PGL, Leiss KA. 2017. Interactive effects of UV-B light with abiotic factors on plant growth and chemistry, and their consequences for defense against arthropod herbivores. Front Plant Sci. 8:278. doi:10.3389/fpls.2017.00278.
- Fang F, Lin L, Zhang Q, Lu M, Skvortsova MY, Podolec R, Zhang Q, Pi J, Zhang C, Ulm R, et al. 2022. Mechanisms of UV-B light-induced photoreceptor UVR8 nuclear localization dynamics. New Phytol. 236(5):1824–1837. doi:10.1111/nph.18468.
- Favory J, Stec A, Gruber H, Rizzini L, Oravecz A, Funk M, Albert A, Cloix C, Jenkins GI, Oakeley EJ, et al. 2009. Interaction of COP1 and UVR8 regulates UV-B-induced photomorphogenesis and stress acclimation in arabidopsis. EMBO J. 28(5):591–601. doi:10.1038/emboj.2009.4.
- Fung F, Wang H-S, Menon S. 2018. Food safety in the 21st century. Biomed J. 41(2):88–95. doi:10.1016/j.bj.2018.03.003.
- Gao G, Liu W, Zhao X, Gao K. 2021. Ultraviolet radiation stimulates activity of CO2 concentrating mechanisms in a bloom-forming diatom under reduced CO2 availability. Front Microbiol. 12:651567. doi:10.3389/fmicb.2021.651567.
- George DS, Razali Z, Santhirasegaram V, Somasundram C. 2016. Effect of postharvest ultraviolet-C treatment on the proteome changes in fresh cut mango (Mangifera indica L. cv. Chokanan). J Sci Food Agric. 96(8):2851–2860. doi:10.1002/jsfa.7454.
- Ghasemzadeh A, Ashkani S, Baghdadi A, Pazoki A, Jaafar HZE, Rahmat A. 2016. Improvement in flavonoids and phenolic acids production and pharmaceutical quality of sweet basil (ocimum basilicum L.) by ultraviolet-B irradiation. Molecules. 21(9):1203. doi:10.3390/molecules21091203.
- Guerrero RF, Cantos-Villar E, Puertas B, Richard T. 2016. Daily preharvest UV-C light maintains the high stilbenoid concentration in grapes. J. Agric. Food Chem. 64(25):5139–5147.
- Gunasegaran B, Ding P, Kadir J. 2018. Morphological identification and in vitro evaluation of colletotrichum gloesporioides in’Chok anan’mango using UV-C irradiation. III Asia Pacific symposium on postharvest research. Education and Extension: Aps. 2014. 1213:p. 599–602.
- Heijde M, Ulm R. 2012. UV-B photoreceptor-mediated signalling in plants. Trends Plant Sci. 17(4):230–237. doi:10.1016/j.tplants.2012.01.007.
- Hideg É, Jansen MAK, Strid Å. 2013. UV-B exposure, ROS, and stress: inseparable companions or loosely linked associates? Trends Plant Sci. 18(2):107–115. doi:10.1016/j.tplants.2012.09.003.
- Hidema J, Kumagai T. 2006. Sensitivity of rice to ultraviolet-B radiation. Ann Bot. 97(6):933–942. doi:10.1093/aob/mcl044.
- Hou FJ, Ben GY. 1999. Effect of UV-B radiation on physiological properties in soybean and cucumber seedlings. Chin J Appl Environ. Biol. 5:455–458.
- Hu L, Yang C, Zhang L, Feng J, Xi W. 2019. Effect of light-emitting diodes and ultraviolet irradiation on the soluble sugar, organic acid, and carotenoid content of postharvest sweet oranges (Citrus sinensis (L. Osbeck)). Molecules. 24(19):3440. doi:10.3390/molecules24193440.
- Idzwana MIN, Sen Chou K, Shah RM, Soh NC. 2020. The effect Of ultraviolet light treatment In extend shelf life and preserve the quality of strawberry (Fragaria x ananassa) cv. festival. Int J Food Agric Nat Resour. 1(1):15–18.
- Janisiewicz WJ, Takeda F, Glenn DM, Camp MJ, Jurick WM. 2016. Dark period following UV-C treatment enhances killing of botrytis cinerea conidia and controls gray mold of strawberries. Phytopathology. 106(4):386–394. doi:10.1094/PHYTO-09-15-0240-R.
- Jansen MAK, Hectors K, O’Brien NM, Guisez Y, Potters G. 2008. Plant stress and human health: Do human consumers benefit from UV-B acclimated crops? Plant Sci. 175(4):449–458. doi:10.1016/j.plantsci.2008.04.010.
- Jenkins GI. 2009. Signal transduction in responses to UV-B radiation. Annu Rev Plant Biol. 60:407–431. doi:10.1146/annurev.arplant.59.032607.092953.
- Jenkins GI. 2017. Photomorphogenic responses to ultraviolet-B light. Plant Cell Environ. 40(11):2544–2557. doi:10.1111/pce.12934.
- Jin P, Wang H, Zhang Y, Huang Y, Wang L, Zheng Y. 2017. UV-C enhances resistance against gray mold decay caused by botrytis cinerea in strawberry fruit. Sci Hortic. 225:106–111. doi:10.1016/j.scienta.2017.06.062.
- Kamel RM, El-Kholy MM, Tolba NM, Amer A, Eltarawy AM, Eltarawy AM, Ali LM. 2022. Influence of germicidal ultraviolet radiation UV-C on the quality of Apiaceae spices seeds. Chem Biol Technol Agric. 9(1):89. doi:10.1186/s40538-022-00358-4.
- Kan J, Hui Y, Lin X, Liu Y, Jin C. 2021. Postharvest ultraviolet-C treatment of peach fruit: changes in transcriptome profile focusing on genes involved in softening and senescence. J Food Process Preserv. 45(10):e15813.
- Kang S, Kim JE, Zhen S, Kim J. 2022. Mild-intensity UV-A radiation applied over a long duration can improve the growth and phenolic contents of sweet basil. Front Plant Sci. 12:858433.
- Kondo S, Fiebig A, Okawa K, Ohara H, Kowitcharoen L, Nimitkeatkai H, Kittikorn M, Kim M. 2011. Jasmonic acid, polyamine, and antioxidant levels in apple seedlings as affected by ultraviolet-C irradiation. Plant Growth Regul. 64(1):83–89. doi:10.1007/s10725-010-9539-9.
- Kroger M, Meister K, Kava R. 2006. Low-calorie sweeteners and other sugar substitutes: a review of the safety issues. Compr Rev Food Sci Food Saf. 5(2):35–47. doi:10.1111/j.1541-4337.2006.tb00081.x.
- Kuhlmann F, Müller C. 2011. Impacts of ultraviolet radiation on interactions between plants and herbivorous insects: a chemo-ecological perspective. Prog. Bot. 72:305–347.
- Kunz BA, Cahill DM, Mohr PG, Osmond MJ, Vonarx EJ. 2006. Plant responses to UV radiation and links to pathogen resistance. Int Rev Cytol. 255(06):1–40. doi:10.1016/S0074-7696(06)55001-6.
- Kunz BA, Dando PK, Grice DM, Mohr PG, Schenk PM, Cahill DM. 2008. UV-Induced DNA damage romotes resistance to the biotrophic pathogen hyaloperonospora parasitica in arabidopsis. Plant Physiol. 148(2):1021–1031. doi:10.1104/pp.108.125435.
- Laoué J, Fernandez C, Ormeño E. 2022. Plant flavonoids in Mediterranean species: A focus on flavonols as protective metabolites under climate stress. Plants. 11(2):172. doi:10.3390/plants11020172.
- Leszczynski K. 1995. Assessment and comparison of methods for solar ultraviolet radiation measurements.
- Li L, Li C, Sun J, Xin M, Yi P, He X, Sheng J, Zhou Z, Ling D, Zheng F, et al. 2021a. Synergistic effects of ultraviolet light irradiation and high-oxygen modified atmosphere packaging on physiological quality, microbial growth and lignification metabolism of fresh-cut carrots. Postharvest Biol Technol. 173:111365. doi:10.1016/j.postharvbio.2020.111365.
- Li P, Yu X, Xu B. 2017. Effects of UV-C light exposure and refrigeration on phenolic and antioxidant profiles of subtropical fruits (litchi, longan, and rambutan) in different fruit forms. 2017.
- Li T, Yamane H. 2021. Preharvest long-term exposure to UV-B radiation promotes fruit ripening and modifies stage-specific anthocyanin metabolism in highbush blueberry. Hortic Res. 8(67). doi:10.1038/s41438-021-00503-4.
- Li T, Yamane H, Tao R. 2021b. Preharvest long-term exposure to UV-B radiation promotes fruit ripening and modifies stage-specific anthocyanin metabolism in highbush blueberry. Hortic Res. 8.
- Liu C, Han X, Cai L, Lu X, Ying T, Jiang Z. 2011. Postharvest UV-B irradiation maintains sensory qualities and enhances antioxidant capacity in tomato fruit during storage. Postharvest Biol Technol. 59(3):232–237. doi:10.1016/j.postharvbio.2010.09.003.
- Liu L, Gregan SM, Winefield C, Jordan B. 2018. Comparisons of controlled environment and vineyard experiments in sauvignon blanc grapes reveal similar UV-B signal transduction pathways for flavonol biosynthesis. Plant Sci. 276:44–53. doi:10.1016/j.plantsci.2018.08.003.
- Liu LH, Zabaras D, Bennett LE, Aguas P, Woonton BW. 2009. Effects of UV-C, red light and sun light on the carotenoid content and physical qualities of tomatoes during post-harvest storage. Food Chem. 115(2):495–500. doi:10.1016/j.foodchem.2008.12.042.
- Liu P, Li Q, Li Y, Yu H, Jiang W. 2017. Effect of UV-B radiation treatments on growth, physiology and antioxidant systems of cucumber seedlings in artificial climate chamber. Trans Chinese Soc Agric Eng. 33(17):181–186.
- Loconsole D. 2021. UV lighting in horticulture : a sustainable tool for improving production quality and food safety.
- Luthria DL, Mukhopadhyay S, Krizek DT. 2006. Content of total phenolics and phenolic acids in tomato (Lycopersicon esculentum Mill.) fruits as influenced by cultivar and solar UV radiation. J Food Compos Anal. 19(8):771–777. doi:10.1016/j.jfca.2006.04.005.
- Malek NI, Wan Abdullah WZ, Wan Sembok WZ. 2021. Effects of UV-C radiation in delaying ripening of Berangan banana (Musa sp. AAA Berangan). Univ Malaysia Teren J Undergrad Res. 3(4):173–182. doi:10.46754/umtjur.v3i4.250.
- Manzocco L, Da Pieve S, Maifreni M. 2011. Impact of UV-C light on safety and quality of fresh-cut melon. Innov Food Sci Emerg Technol. 12(1): 13–17. doi:10.1016/j.ifset.2010.11.006.
- Manzocco L, Plazzotta S, Maifreni M, Calligaris S, Anese M, Nicoli MC. 2016. Impact of UV-C light on storage quality of fresh-cut pineapple in two different packages. LWT-Food Sci Technol. 65:1138–1143. doi:10.1016/j.lwt.2015.10.007.
- Martins ACQ, Mota APZ, Carvalho PASV, Passos MAS, Gimenes MA, Guimaraes PM, Brasileiro ACM. 2022. Transcriptome responses of wild arachis to UV-C exposure reveal genes involved in general plant defense and priming. Plants. 11(3):408. doi:10.3390/plants11030408.
- Martínez-Silvestre KE, Santiz-Gómez JA, Luján-Hidalgo MC, Ruiz-Lau N, Sánchez-Roque Y, Gutiérrez-Miceli FA. 2022. Effect of UV-B radiation on flavonoids and phenols accumulation in tempisque (Sideroxylon capiri pittier) callus. Plants. 11(4):473. doi:10.3390/plants11040473.
- Mclay ER, Pontaroli AC, Wargent JJ. 2020. UV-B induced flavonoids contribute to reduced biotrophic disease susceptibility in lettuce seedlings. Front. Plant Sci. 11:594681.
- Mditshwa A, Samukelo L, Samson M, Tesfay Z. 2017. Effect of ultraviolet irradiation on postharvest quality and composition of tomatoes : a review. J Food Sci Technol. 54(10):3025–3035. doi:10.1007/s13197-017-2802-6.
- Meyer P, Van de Poel B, De Coninck B. 2021. UV-B light and its application potential to reduce disease and pest incidence in crops. Hortic Res. 8(1). doi:10.1038/s41438-020-00428-4.
- Mmbando GS, Ando S, Takahashi H, Hidema J. 2023. High ultraviolet - B sensitivity due to lower CPD photolyase activity is needed for biotic stress response to the rice blast fungus, Magnaporthe oryzae. Photochem Photobiol Sci. 0123456789. doi:10.1007/s43630-023-00379-4.
- Mmbando GS, Hidema J. 2021. The trade-off between UVB sensitivity and tolerance against other stresses in African rice species. Turk J Botany 45(SI-1): 601–612. doi:10.3906/bot-2109-38.
- Mmbando GS, Teranishi M, Hidema J. 2020. Very high sensitivity of African rice to artificial ultraviolet-B radiation caused by genotype and quantity of cyclobutane pyrimidine dimer photolyase. Sci Rep. 1–14. doi:10.1038/s41598-020-59720-x.
- Mmbando GS, Teranishi M, Hidema J. 2021. Transgenic rice oryza glaberrima with higher CPD photolyase activity alleviates UVB-caused growth inhibition. GM Crop Food. 12(1):435–448. doi:10.1080/21645698.2021.1977068.
- Mohamed NTS, Ding P, Ghazali HM, Kadir J. 2017. Biochemical and cell wall ultrastructural changes in crown tissue of banana (musa AAA ‘berangan’) fruit as mediated by UVC irradiation against crown rot fungal infection. Postharvest Biol Technol. 128:144–152. doi:10.1016/j.postharvbio.2017.02.004.
- Mosadegh H, Trivellini A, Ferrante A, Lucchesini M, Vernieri P, Mensuali A. 2018. Applications of UV-B lighting to enhance phenolic accumulation of sweet basil. Sci. Hortic. 229:107–116. doi:10.1016/j.scienta.2017.10.043.
- Mújica-Paz H, Valdez-Fragoso A, Samson CT, Welti-Chanes J, Torres JA. 2011. High-pressure processing technologies for the pasteurization and sterilization of foods. Food Bioprocess Technol. 4:969–985. doi:10.1007/s11947-011-0543-5.
- Mukhopadhyay S, Ukuku DO, Juneja V, Fan X. 2014. Effects of UV-C treatment on inactivation of salmonella enterica and escherichia coli O157: H7 on grape tomato surface and stem scars, microbial loads, and quality. Food Control. 44:110–117. doi:10.1016/j.foodcont.2014.03.027.
- Nenadis N, Llorens L, Koufogianni A, Díaz L, Font J, Gonzalez JA, Verdaguer D. 2015. Interactive effects of UV radiation and reduced precipitation on the seasonal leaf phenolic content/composition and the antioxidant activity of naturally growing Arbutus unedo plants. J Photochem Photobiol B Biol. 153:435–444. doi:10.1016/j.jphotobiol.2015.10.016.
- Nunes MPC, Vespucci IL, Rimoli PAR, Morgado CMA, de Campos AJ. 2024. Postharvest of fresh white Shimeji mushroom subjected to UV-C radiation. Heliyon 10(3):e25115.
- Obande MA, Tucker GA, Shama G. 2011. Effect of preharvest UV-C treatment of tomatoes (Solanum lycopersicon Mill.) on ripening and pathogen resistance. Postharvest Biol Technol. 62(2):188–192. doi:10.1016/j.postharvbio.2011.06.001.
- Onik JC, Xie Y, Duan Y, Hu X, Wang Z, Lin Q. 2019. UV-C treatment promotes quality of early ripening apple fruit by regulating malate metabolizing genes during postharvest storage. PLoS One. 14(4):e0215472.
- Ortiz-Araque LC, Darré M, Civello PM, Vicente A. 2022. Short UV-C treatments to extend the shelf-life of fresh-Cut strawberries (Fragaria x ananassa Duch cv. Camarosa). Ing e Investig. 42:3.
- Otake M, Okamoto Yoshiyama K, Yamaguchi H, Hidema J. 2021. 222 nm ultraviolet radiation C causes more severe damage to guard cells and epidermal cells of arabidopsis plants than does 254 nm ultraviolet radiation. Photochem Photobiol Sci. 20:1675–1683. doi:10.1007/s43630-021-00123-w.
- Palma CFF, Castro-Alves V, Morales LO, Rosenqvist E, Ottosen C-O, Strid Å. 2021. Spectral composition of light affects sensitivity to UV-B and photoinhibition in cucumber. Front Plant Sci. 11:610011. doi:10.3389/fpls.2020.610011.
- Peng H, Pang Y, Liao Q, Wang F. 2022. The effect of preharvest UV light irradiation on berries quality : A review.: 1–14.
- Piccini C, Cai G, Dias MC, Romi M, Longo R, Cantini C. 2020. UV-B Radiation affects photosynthesis-related processes of Two Italian Olea europaea (L). Var Diff. 9(12):1712.
- Pinheiro J, Alegria C, Abreu M, Gonçalves EM, Silva CLM. 2015. Use of UV-C postharvest treatment for extending fresh whole tomato (Solanum lycopersicum, cv. Zinac). Shelf-Life. 52(8):5066–5074. doi:10.1007/s13197-014-1550-0.
- Podolec R, Lau K, Wagnon TB, Hothorn M, Ulm R. 2021. A constitutively monomeric UVR8 photoreceptor confers enhanced UV-B photomorphogenesis. Proc Natl Acad Sci. 118(6):e2017284118. doi:10.1073/pnas.2017284118.
- Prajapati U, Asrey R, Varghese E, Singh AK, Singh MP. 2021. Effects of postharvest ultraviolet-C treatment on shelf-life and quality of bitter gourd fruit during storage. Food Packag Shelf Life. 28:100665. doi:10.1016/j.fpsl.2021.100665.
- Qi J, Zhang M, Lu C, Hettenhausen C, Tan Q, Cao G, Zhu X, Wu G, Wu J. 2018. Ultraviolet-B enhances the resistance of multiple plant species to lepidopteran insect herbivory through the jasmonic acid pathway. Sci Rep. 8(1):1–9. doi:10.1038/s41598-017-18600-7.
- Rai N, Morales LO, Aphalo PJ. 2021. Perception of solar UV radiation by plants: photoreceptors and mechanisms. Plant Physiol. 186(3):1382–1396. doi:10.1093/plphys/kiab162.
- Rizzini L, Favory J-J, Cloix C, Faggionato D, O’Hara A, Kaiserli E, Baumeister R, Schäfer E, Nagy F, Jenkins GI, et al. 2011. Perception of UV-B by the arabidopsis UVR8 protein. Science (80-.). 332(6025):103–106.
- Robson TM, Klem K, Urban O, Jansen MAK. 2015. Re-interpreting plant morphological responses to UV-B radiation. Plant Cell Environ. 38(5):856–866. doi:10.1111/pce.12374.
- Rosa M, Prado C, Podazza G, Interdonato R, González JA, Hilal M, Prado F. 2009. Soluble sugars. Plant Signal Behav. 4:388–393. doi:10.4161/psb.4.5.8294.
- Rozema J, Noordijk AJ, Broekman RA, Van Beem A, Meijkamp BM, De Bakker NVJ. 2001. (Poly) phenolic compounds in pollen and spores of antarctic plants as indicators of solar UV-B–A new proxy for the reconstruction of past solar UV-B? Plant Ecol. 154(1): 9–26.
- Ruiz VE, Cerioni L, Zampini IC, Cuello S, Isla MI, Hilal M, Rapisarda VA. 2017. UV-B radiation on lemons enhances antifungal activity of flavedo extracts against penicillium digitatum. LWT-Food Sci Technol. 85:96–103. doi:10.1016/j.lwt.2017.07.002.
- Ruiz VE, Interdonato R, Cerioni L, Albornoz P, Ramallo J, Prado FE, Hilal M, Rapisarda VA. 2016. Short-term UV-B exposure induces metabolic and anatomical changes in peel of harvested lemons contributing in fruit protection against green mold. J Photochem Photobiol B Biol. 159:59–65. doi:10.1016/j.jphotobiol.2016.03.016.
- Sánchez GJ, Contigiani EV, Coronel MB, Alzamora SM, García-Loredo A, Nieto AB. 2021. Study of UV-C treatments on postharvest life of blueberries ‘O’Neal’and correlation between structure and quality parameters. Heliyon. 7:6.
- Saxena S, Joshi P, Grimm B, Arora S. 2011. Alleviation of ultraviolet-C-induced oxidative damage through overexpression of cytosolic ascorbate peroxidase. Biologia (Bratisl). 66(6):1052–1059. doi:10.2478/s11756-011-0120-4.
- Schreiner M, Mewis I, Neugart S, Zrenner R, Glaab J, Wiesner M, Jansen MAK. 2016. UV-B elicitation of secondary plant metabolites. III-Nitride Ultrav Emit Technol Appl. 387–414.
- Severo J, Tiecher A, Pirrello J, Regad F, Latché A, Pech J-C, Bouzayen M, Rombaldi CV. 2016. UV-C radiation modifies the ripening and accumulation of ethylene response factor (ERF) transcripts in tomato fruit. Postharvest Biol Technol 102:9–16. doi:10.1016/j.postharvbio.2015.02.001.
- Siipola SM, Kotilainen T, Sipari N, Morales LO, Lindfors AV, Robson TM, Aphalo PJ. 2015. Epidermal UV-A absorbance and whole-leaf flavonoid composition in pea respond more to solar blue light than to solar UV radiation. Plant Cell Environ. 38(5):941–952. doi:10.1111/pce.12403.
- Singh H, Bhardwaj SK, Khatri M, Kim K., Bhardwaj N. 2020. UVC radiation for food safety: An emerging technology for the microbial disinfection of food products. Chem Eng J. 128084. doi:10.1016/j.cej.2020.128084.
- Solovchenko A, Merzlyak M. 2003. Optical properties and contribution of cuticle to UV protection in plants: experiments with apple fruit. Photochem Photobiol Sci. 2(8):861–866. doi:10.1039/b302478d.
- Soni A, Oey I, Silcock P, Bremer P. 2016. Bacillus spores in the food industry: A review on resistance and response to novel inactivation technologies. Compr Rev Food Sci Food Saf. 15(6):1139–1148. doi:10.1111/1541-4337.12231.
- Sripong K, Jitareerat P, Tsuyumu S, Uthairatanakij A, Srilaong V, Wongs-Aree C, Ma G, Zhang L, Kato M. 2015. Combined treatment with hot water and UV-C elicits disease resistance against anthracnose and improves the quality of harvested mangoes. Crop Prot. 77:1–8. doi:10.1016/j.cropro.2015.07.004.
- Stevens C, Khan VA, Lu JY, Wilson CL, Pusey PL, Igwegbe ECK, Kabwe K, Mafolo Y, Liu J, Chalutz E, Droby S. 1997. Integration of ultraviolet (UV-C) light with yeast treatment for control of postharvest storage rots of fruits and vegetables. Biol Control. 10(2):98–103. doi:10.1006/bcon.1997.0551.
- Stevens C, Liu J, Khan VA, Lu JY, Kabwe MK, Wilson CL, Igwegbe ECK, Chalutz E, Droby S. 2004. The effects of low-dose ultraviolet light-C treatment on polygalacturonase activity, delay ripening and rhizopus soft rot development of tomatoes. Crop Prot. 23(6):551–554. doi:10.1016/j.cropro.2003.10.007.
- Stockenhuber R, Akiyama R, Tissot N, Milosavljevic S, Yamazaki M, Wyler M, Arongaus AB, Podolec R, Sato Y, Widmer A, et al. 2024. UV RESISTANCE LOCUS 8–mediated UV-B response Is required alongside CRYPTOCHROME 1 for plant survival in sunlight under field conditions. Plant Cell Physiol. 65(1):35–48. doi:10.1093/pcp/pcad113.
- Sun LH, Li ZH, Duan LS, Wei ZG, Wang Q. 2006. Effect of UV-B radiation on the growth and photosynthesis of cucumber (Cucumis sativus L. seedlings). Acta Agric Boreali-Sin. 21:79–82.
- Sun M, Jordan B, Creasy G, Zhu Y-F. 2023. UV-B Radiation induced the changes in the amount of amino acids. Phenolics and Aroma Compounds in Vitis Vinifera cv. Pinot Noir Berry Under Field Conditions. Foods. 12(12):2350.
- Takshak S, Agrawal SB. 2014. Effect of ultraviolet-B radiation on biomass production, lipid peroxidation, reactive oxygen species, and antioxidants in withania somnifera. Biol. Plant. 58:328–334. doi:10.1007/s10535-014-0390-0.
- Tiecher A, de Paula LA, Chaves FC, Rombaldi CV. 2013. UV-C effect on ethylene, polyamines and the regulation of tomato fruit ripening. Postharvest Biol. Technol. 86: 230–239. doi:10.1016/j.postharvbio.2013.07.016.
- Tripathi D, Meena RP, Pandey-Rai S. 2021. Short term UV-B radiation mediated modulation of physiological traits and withanolides production in Withania coagulans (L.) dunal under in-vitro condition. Physiol Mol Biol Plants. 27(8):1823–1835. doi:10.1007/s12298-021-01046-7.
- Ueda T, Nakamura C. 2011. Ultraviolet-defense mechanisms in higher plants. Biotechnol Biotechnol Equip. 25(1):2177–2182. doi:10.5504/BBEQ.2011.0001.
- Ulm R, Nagy F. 2005. Signalling and gene regulation in response to ultraviolet light. Curr Opin Plant Biol. 8(5):477–482. doi:10.1016/j.pbi.2005.07.004.
- Urban L, Charles F, de Miranda MRA, Aarrouf J. 2016. Understanding the physiological effects of UV-C light and exploiting its agronomic potential before and after harvest. Plant Physiol Biochem. 105: 1–11. doi:10.1016/j.plaphy.2016.04.004.
- Usall J, Ippolito A, Sisquella M, Neri F. 2016. Physical treatments to control postharvest diseases of fresh fruits and vegetables. Postharvest Biol Technol. 122:30–40. doi:10.1016/j.postharvbio.2016.05.002.
- Vanhaelewyn L, Van Der Straeten D, De Coninck B, Vandenbussche F. 2020. Ultraviolet radiation from a plant perspective: The plant-microorganism context. Front Plant Sci. 11: 1984.
- Vàsquez H, Ouhibi C, Forges M, Lizzi Y, Urban L, Aarrouf J. 2020. Hormetic doses of UV-C light decrease the susceptibility of tomato plants to botrytis cinerea infection. J Phytopathol. 168(9):524–532. doi:10.1111/jph.12930.
- Volkova PY, Bondarenko EV, Kazakova EA. 2022. Radiation hormesis in plants. Curr Opin Toxicol. 30:100334. doi:10.1016/j.cotox.2022.02.007.
- Wang H, Gui M, Tian X, Xin X, Wang T, Wang T, li J. 2017. Effects of UV-B on vitamin C, phenolics, flavonoids and their related enzyme activities in mung bean sprouts (Vigna radiata). Int J Food Sci Technol. 52(3):827–833. doi:10.1111/ijfs.13345.
- Xiao S, Chen S, Zhao L, Wang G. 2006. Density effects on plant height growth and inequality in sunflower populations. J Integr Plant Biol. 48(5):513–519. doi:10.1111/j.1744-7909.2006.00265.x.
- Xu G, Li C, Qin S, Xiao W, Fu X, Chen X, Li L, Li D. 2022. Changes in sucrose and sorbitol metabolism cause differences in the intrinsic quality of peach fruits cultivated in field and greenhouse environments. Agronomy. 12(11):2877. doi:10.3390/agronomy12112877.
- Xu Y, Luo Z, Charles MT, Rolland D, Roussel D. 2017. Pre-harvest UV-C irradiation triggers VOCs accumulation with alteration of antioxidant enzymes and phytohormones in strawberry leaves. J Plant Physiol. 218:265–274. doi:10.1016/j.jplph.2017.09.002.
- Xue S, Zang Y, Chen J, Shang S, Gao L, Tang X. 2022. Ultraviolet-B radiation stress triggers reactive oxygen species and regulates the antioxidant defense and photosynthesis systems of intertidal red algae neoporphyra haitanensis. Front Mar Sci. 9:1043462. doi:10.3389/fmars.2022.1043462.
- Yang J, Li B, Shi W, Gong Z, Chen L, Hou Z. 2018. Transcriptional activation of anthocyanin biosynthesis in developing fruit of blueberries (Vaccinium corymbosum L.) by preharvest and postharvest UV irradiation. J Agric Food Chem. 66(42):10931–10942. doi:10.1021/acs.jafc.8b03081.
- Zhang W, Jiang W. 2019. UV treatment improved the quality of postharvest fruits and vegetables by inducing resistance. Trends Food Sci Technol. 92:71–80. doi:10.1016/j.tifs.2019.08.012.
- Zhao Y, Zuo J, Yuan S, Shi W, Shi J, Feng B, Wang Q. 2021. UV-C Treatment maintains the sensory quality, antioxidant activity and flavor of pepino fruit during postharvest storage. Foods. 2021(10):2964. doi:10.3390/foods10122964.
- Zhou H, Zhang X, Su M, Du J, Li X, Ye Z.-W. 2020. Effects of ultraviolet-c pretreatment on sugar metabolism in yellow peaches during shelf life. HortScience. 55(4):416–423. doi:10.21273/HORTSCI14554-19.