Abstract
The epitaxial lateral growth of single-crystal diamond (SCD) using a plate-to-plate microwave plasma chemical vapor deposition (MPCVD) reactor under high pressure is investigated. The radicals’ distribution in H2/CH4 plasma as a function of pressure was locally detected by optical emission spectroscopy (OES). Raman spectroscopy and optical microscope were employed to analyze the properties of SCD deposited in different pressure. The OES results show that radicals’ distribution along the substrate direction is symmetrical under 20 kPa pressure. The symmetrical distribution of radicals at 20 kPa is in favor of epitaxial lateral growth SCD around the seed and without polycrystalline diamond (PCD) rim. When the pressure is increased to 21.5 kPa, the optical emission spectra center of plasma shifts close to the microwave reflector where is far away from the microwave source. The contact state between the diamond seed and the plasma is deteriorated and the PCD rim occurs in the plasma uncovered area. While the epitaxial lateral growth pattern occurs in the plasma covered area and the lateral growth rate of this region improves with the increase of pressure. A higher growth rate does not result in good quality; meanwhile, the diamond growth step spacing and direction become inconsistent in the transition zone as a function of pressure increasing. Finally, the overall effective lateral expansion area does not increase with the improvement of pressure. Therefore, the uniform and symmetrical distributed plasma is more conducive to the epitaxial lateral growth of SCD, and the effective expansion growth SCD can be realized at 20 kPa.
1. Introduction
Diamond has outstanding physical and chemical properties, such as high hardness, high thermal conductivity (2000 W·m−1·K−1 at room temperature), wide band gap (5.47 eV), high mobility, high light transmittance and wide light transmission range. Not only has it been widely used in traditional mechanical processing, heat sinks, vacuum/optical windows and other industrial applications, but also diamond has potential application in cutting-edge technology fields such as quantum computing, radiation/ultraviolet detection, and integrated circuit power devices [Citation1–4]. Compared with the conventional microcrystalline diamond (MCD) thin film, single-crystal diamond (SCD) has outstanding electrical and optical characteristic due to the nonexistence of abundant grain boundaries in the film. These unique properties greatly extend the application fields of SCD, recently attracting more and more research interest in the preparation and application of large-size, high-quality SCD. Aharonovich et al. [Citation1] reported homoepitaxial growth of single-crystal diamond membranes for quantum information processing and epitaxial growth of good quality SCD even on damaged diamond template. Wan et al. [Citation2] based on the excellent solid-state qubits of SCD and combined with semiconductor process technology, the largest size of quantum chip was fabricated. Delfaure et al. [Citation3] applied SCD membranes in the structure of betavoltaic cells and SCD can realize of long-life radioisotope based micro-batteries.
In order to meet the demand for high-speed synthesis of high quality and large SCD applied in the forefront research, a high power density microwave plasma source is required, the general method is to increase the power supply or increase the deposition pressure [Citation4–6]. Without raising the cost of research, high-pressure epitaxial growth of SCD is more feasible. However, under high deposition pressure, the volume of the microwave plasma ball is not conducive to the preparation of large SCD. The epitaxial lateral growth provides a possibility to achieve high-speed epitaxial growth of large SCD under high pressure. Ichikawa and Schreck et al. [Citation7,Citation8] reported epitaxial lateral overgrowth of high quality SCD with low dislocation density using Ir/MgO and SiO2 masks. Nad et al[Citation9] studied the structure of seed holder on SCD growth and successful lateral growth of SCD in a “pocket holder” under 31.99 kPa pressure. Zhao et al. [Citation10] compared the quality of SCD grown on HPHT and CVD seed substrates under high pressure (25–27 kPa) and also observed the lateral growth phenomenon of SCD. The above papers focus on researching the application of SCD, and studying the influence of heterogeneous substrate, seeds holder structure, and seed type on the quality of SCD epitaxial growth. However, the influence of the radicals’ distribution on epitaxial growth of SCD was rarely discussed, and the epitaxial quality and growth rate of SCD are directly related to the distribution of plasma radicals around the diamond seed. To figure out the effect of radicals’ distribution on SCD lateral growth, we carried out the experiments in a plate-to-plate MPCVD reactor with semi-open substrate under high pressure (≥20 kPa). Emission spectroscopy was employed to study the effect of pressure on the plasma radicals distribution during SCD lateral growth. At last, combined with the results of the Raman spectroscopy and surface morphology, the influence of radicals’ distribution on the epitaxial lateral growth of SCD was analyzed.
2. Experiments
Homoepitaxial lateral growth of SCD was carried out using a plate-to-plate MPCVD system, shows the schematic diagram of MPCVD and SCD seed on a semi-open substrate of Mo. More details about the reactor can be found in [Citation5], in which our work on development of novel reactor configuration for diamond synthesis has been reported. During microwave plasma CVD processes, H2/CH4 mixture gas was used with methane gas volume fraction of 3.0 vol.% at pressure of 15–25 kPa, and the epitaxial lateral growth time was 6–50 h. The input microwave power was 1.25 kW during the growth. And the seed temperature was 760 °C– 890 °C which was monitored by the IRCON IR-AH infrared thermometer.
The <100> oriented HPHT type Ib diamond seed (∼3 mm × 3 mm × 1 mm) was placed in a semi-open Mo holder, as shown in . The diameter of the holder was 12 mm, the width of recess was 4 mm and the height of the vertical step between the upper surface of diamond seed and the upper surface of holder was 0.6 mm before growth. Since the seed will undergo multiple discontinuous growths, both sides of the seed have to be polished and ultrasonic cleaned with acetone. shows optical microscopy images of as-grown SCD seed after polishing, the PCD rim around the seed was polished to flat by an automatic polishing machine with cast iron plate, and the black region outside of the red dashed line is the PCD rim remnant. The top surface roughness of the CVD layer after polishing was about 50 nm.
During epitaxial lateral growth of SCD, along the narrow side of the compressed waveguide reactor, the Maya2000PRO 2 D FFT-CCD spectrometer (Ocean Optics) was employed to online measure H2/CH4 (3 vol.%) plasma under different pressures. The integration time of the spectrometer was 30 ms and the average sampling number was 10. The focusing lens was located on a two-dimensional adjustable stage which was parallel to the upper surface of the substrate, and the center point of the substrate was acted as the zero point of spectrum measurement as shown in .
After epitaxial lateral growth of SCD, the morphology of SCD samples were characterized by BH200M optical microscope and HIROX RH-2000 3 D digital microscopy. Raman spectra were obtained by Thermofisher DXR laser Raman spectrometer to determine the diamond purity. The thickness of the SCD samples were measured by a Koslo digital thickness gauge, and the measurement accuracy is 0.001 mm.
3. Results and discussion
3.1 Optical spectroscopy for epitaxial lateral growth of SCD
In order to study the effect of deposition pressure on radicals distribution in H2/CH4 (3 vol.%) plasma during epitaxial lateral growth of SCD, the H2/CH4 (3 vol.%) plasma was spatially diagnosed by OES. In the measurement of plasma, the center of lens was flush with the substrate, the center position of substrate was acted as the zero point, and diamond seed was placed in the area of −2 mm to 2 mm. shows C2 (516.0 8 nm) radicals distribution along the substrate as a function of pressure. The intensity of C2 (516.08 nm) increased sharply in the center region of plasma (−2 mm to 2 mm) with the increase of pressure. On the one hand, the increase of pressure accelerated the dissociation of methane, and the concentration of C2 (516.08 nm) radicals increased. On the other hand, the shrinkage of plasma ball volume under high pressure resulted in radicals aggregating in the central region which showed the relatively concentration of C2 (516.08 nm) radicals in this region was high. In addition, when the pressure is between 15 and 20 kPa, the center of the plasma coincided with the centerline of the seed holder. While the pressure rose to 21.5 kPa, the center of the plasma shifted to the right where is far away from the microwave source without modifying any structure of MPCVD reactor. The change of radicals’ distribution directly reflects the change of electromagnetic field in microwave plasma. The shift of the plasma center shows that adjusting deposition pressure can effectively control the electromagnetic field in a plate-to-plate structure microwave reactor. In turn, we can control the plasma contact state with diamond seed through adjusting the process pressure.
shows the emission spectra distribution of H2/CH4 (3 vol.%) plasma along the substrate at 23 kPa, the plasma center deviates from the seed holder center. And the intensity of Hβ, Hγ, CH and C2 radicals in the region of 1 mm to 4 mm were higher than the radicals intensity in the axisymmetric region (−4 mm to −1 mm) caused two different coverage state of diamond seeds by plasma. These two states were in contrast, then we will combine the results of SCD epitaxial lateral growth with the OES results to analyze the influence of the contact state (radicals distribution) between diamond seed and H2/CH4 (3 vol.%) plasma on the morphology, quality and growth rate of SCD. Until now, the role of detectable radicals for the diamond precursors is considerable controversy [Citation11–15]. By referring to the general trend of carbon-related radicals’ emission, we propose that the other radicals may have similar distribution [Citation15–17].
3.2 Influence of deposition pressure on epitaxial lateral growth of SCD
shows the images of SCD samples grown at different pressures with methane gas volume fraction of 3.0 vol.% and the input microwave power was 1.25 kW. The other growth parameters for three samples are summarized in . The whole top surface and edge region of sample (a) growth in SCD pattern without PCD rim under 20 kPa pressure. The black edge around sample (a) is actually the PCD rim left by the previous experimental growth. Before this experiment, the sample used for epitaxial lateral growth was polished to a flat surface, as shown in . –A4) and shows the optical microscopy images of SCD sample (a) grown under 20 kPa, there is no polycrystalline diamond phase grown on all edges and corners. The SCD layer grown on the former PCD rim remnant around the seed as shown in , indicated that the SCD layer was epitaxially grown from the center to the edge of HPHT diamond seed. As a result, the top surface and edge regions were in SCD growth pattern. This result is consistent with the result of SCD growth without PCD rim on a “pocket holder” reported by Nad et al. [Citation9]. Because the radicals concentration in the center of the plasma was higher under a high pressure (20 kPa, as shown in ,and the SCD layer in the center region grown rapidly. Then the seed formed an off-axis direction that the epitaxial layer after 9 h growth has developed into an off-angle of 1.3°-1.5° on the (100) crystal face. And the top surface area of sample (a) has increased to ∼1.17 times from 3.13 mm × 3.22 mm to 3.38 mm × 3.5 mm. While these self-formed special structures were conducive to epitaxial lateral growth of SCD from the center of the HPHT seed towards the edge of the seed with high quality. Meanwhile, the study by Mayr and Klein [Citation18,Citation19] verified the feasibility of this believed structure for epitaxial lateral growth of SCD.
Figure 6. Optical microscopy images of as-grown SCD edge regions, (A1–A4) 20 kPa; (B1–B4) 21.5 kPa; (C1–C4) 23 kPa.
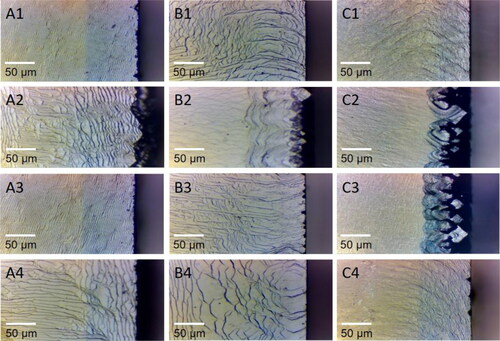
Figure 7. (a) Optical microscopy images of SCD sample on corners grown under 20 kPa. (b and c) Optical microscopy image viewed on the side of sample (a).
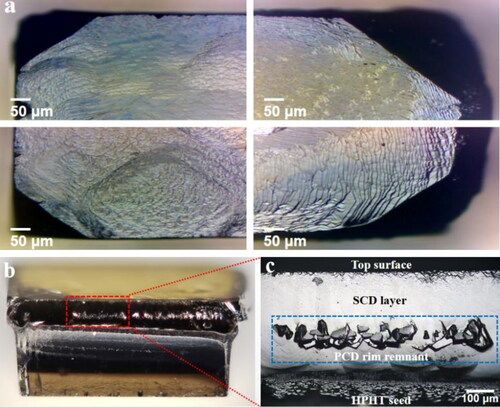
Table 1. Parameters for SCD growth.
With the increase of pressure, discontinuous PCD appeared at the edge of sample (b) () at 21.5 kPa, as shown in . As the pressure rose continuously, the sample (c)() grown at 23 kPa has two sides ( and C3) occurred continuous PCD rim. For the plasma center shifted as a function of pressure increasing, the contact state between seeds and plasma were deteriorated in the region from −4 mm to −1 mm, as shown in and Citation4. The temperature of uncovered seed sides (B2 and C2) is lower than the optimum epitaxial growth temperature of 850–1050 °C [Citation5,Citation20,Citation21] due to the heat of substrate is supplied by microwave plasma self-heating. The distribution of seed surface temperature has a direct effect on the epitaxial growth of SCD, which was not conducive to the fast growth of the crystal faces at lower temperature, especially in the directions of <110> and <111 > [Citation20–22]. At the same time, due to the plasma center deviation, the edge effect [Citation9,Citation20] on the side of seed away from the plasma center was prominent, which has accelerated secondary nucleation, formed polycrystalline phase and eventually grown into a PCD rim. Due to the appearance of PCD rim on sample (b) and (c), the area of lateral expansion growth region of samples (b) and (c) decreased.
Although PCD rim began to appear in one or two sides of seed (b) and (c) with the increase of deposition pressure, the three samples all showed a high-quality epitaxial lateral growth pattern on the other edge regions () and formed the corresponding lateral epitaxial growth area as shown in and Citation8. displays optical photograph of SCD lateral growth transition zone and the corresponding Raman spectrum. In , the layered morphology in epitaxial lateral growth region is consistent with that of the transition region. With the increase of deposition pressure, the SCD typically growth step spacing and direction in epitaxial lateral expansion region of samples (b) and (c) became inconsistent. When the pressure ≥21.5 kPa pressure, in the plasma fully covered region from 1 mm to 4 mm, there was high concentration of carbon source for growth and high temperature of seed substrate, as shown in . The diffusion length and speed of radicals were high under a higher temperature in the plasma fully covered region, resulted in a wide growth step spacing and uneven morphology distribution. The Raman spectra results in show that all three samples have sharp diamond peaks near 1332 cm−1, and the fluorescence backgrounds of samples (a) and (b) are relatively straight. And for sample (c) which grown at 23 kPa, a wave-packet appears at 1460 cm−1 indicates the non-diamond phase exists in the SCD growth layer [Citation23,Citation24].
Figure 8. Optical photograph of SCD lateral growth transition zone and the corresponding Raman spectrum, (a) 20 kPa; (b) 21.5 kPa; and (c) 23 kPa.
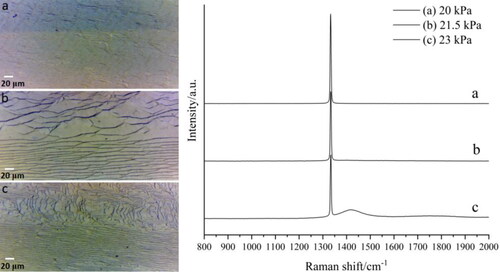
shows the results of the SCD epitaxial lateral growth rate. Under 20 kPa pressure, the vertical growth rate of top surface and the lateral growth rate of edge regions were same, both were about 15.25 μm/h. When the pressure rose from 20 kPa to 23 kPa, the vertical growth rate of top surface increased 33.31% to 20.33 μm/h, while the lateral growth rate of seed edge increased 37.04% to 20.94 μm/h. The ratio of lateral growth rate to vertical growth rate has slightly increased. When the deposition pressure ≥21.5 kPa, the concentration of carbon radicals source was increased in the plasma fully covered area (from 1 mm to 4 mm, as shown in and Citation4, which leads to both the lateral growth rate and the vertical growth rate increase, but the lateral growth rate is slightly faster than the vertical growth rate, resulted in the increasing of the ratio of lateral growth rate to vertical growth rate. The lateral growth rate in a certain region can be improved by increasing the deposition pressure, at the same time it will cause uneven distribution of surface morphology and deterioration of crystal quality. Therefore, by rationally designing the geometric structure of seed holder and optimizing the coordination with SCD growth parameters to obtain the microwave plasma with high power density and uniform distribution of radicals is the prerequisite for rapid epitaxial lateral growth of high-quality, large SCD.
4. Summary
Investigation of SCD epitaxial lateral growth by a plate-to-plate MPCVD under high pressure (≥20 kPa), using H2/CH4 (3 vol.%) as feed gas. OES was employed to spatially study the influence of pressure on the radicals’ distribution in plasma. And we analyzed the relationship between radicals distribution and epitaxial lateral growth of SCD. It is found that the increase of pressure would cause plasma center shift far away from microwave source and the radicals aggregate in the center region. So we can modify the contact state between H2/CH4 plasma and diamond seed by adjusting the pressure, and the epitaxial lateral growth behavior of SCD under different plasma states was studied. The morphology and quality of SCD were characterized by optical microscope, stereoscopic microscope and Raman spectrometer. The OES information and SCD samples test results have been integrated and analyzed, we found that the C2 radicals were distributed symmetrically along the substrate at 20 kPa, and the SCD sample growth without PCD rim. The morphology and quality distribution were uniform, and the vertical growth rate of top surface was the same as the lateral growth rate. The radicals’ concentration in the central region in the plasma improved with the increase of pressure, and the center is shifted, resulted in a poor contact state between diamond seed and the plasma. Meanwhile, PCD rim has appeared on the two sides of SCD sample at 23 kPa. The diamond seed edge region completely covered by the plasma still shows the SCD growth pattern. At 23 kPa, the epitaxial lateral growth was increased to 20.94 μm/h, but the uniformity of SCD surface morphology has deteriorated, and the crystal quality has decreased. The above results show that microwave plasma with uniform and symmetrical radicals’ distribution is more suitable for epitaxial lateral growth of SCD. In these kinds of plasma state, the temperature distribution on seed surface is more uniform, and the precursor radicals’ concentration in the central area is slightly higher than that of in edge region which is conducive to form an off-axis direction structure, thereby epitaxial lateral expansion growth of SCD can be realized.
Disclosure statement
No potential conflict of interest was reported by the author(s).
Additional information
Funding
Notes on contributors
Wei Cao
Mr. Wei Cao is a PhD student from Wuhan Institute of Technology. His main research interests include plasmatechnology and functional thin films.
Deng Gao
Mr. Deng Gao studying in Wuhan Institute of Technology as a graduate student. His current research topic is about the epitaxial growth of single crystal diamond by MPCVD.
Hongyang Zhao
Prof. Hongyang Zhao is working in Wuhan Institute of Technology, as a professor. Her main research interests include synthesis and characterization of functional photoelectric crystals.
Zhibin Ma
Prof. Zhibin Ma is a PhD supervisor. The main research interests include plasma technology and MP CVD diamond.
Reference
- Aharonovich I, Lee JC, Magyar AP, et al. Homoepitaxial growth of single crystal diamond membranes for quantum information processing. Adv Mater. 2012;24(10):OP54–OP59.
- Wan NH, Lu T-J, Chen KC, et al. Large-scale integration of artificial atoms in hybrid photonic circuits. Nature. 2020;583(7815):226–231.
- Delfaure C, Pomorski M, de Sanoit J, et al. Single crystal CVD diamond membranes for betavoltaic cells. Appl Phys Lett. 2016;108(25):252105.
- Muehle M, Asmussen J, Becker MF, et al. Extending microwave plasma assisted CVD SCD growth to pressures of 400 Torr. Diamond Relat Mater. 2017;79:150–163.
- Zhibin M, Chao W, Wang J, et al. Development of a plate-to-plate MPCVD reactor configuration for diamond synthesis. Diamond Relat Mater. 2016;66:135–140.
- Lu J, Gu Y, Grotjohn TA, et al. Experimentally defining the safe and efficient, high pressure microwave plasma assisted CVD operating regime for single crystal diamond synthesis. Diamond Relat. Mater. 2013;37:17–28.
- Ichikawa K, Kurone K, Kodama H, et al. High crystalline quality heteroepitaxial diamond using grid-patterned nucleation and growth on Ir. Diamond Relat Mater. 2019;94:92–100.
- Schreck M, Mayr M, Weinl M, et al. Liftoff of single crystal diamond by epitaxial lateral overgrowth using SiO2 masks. Diamond Relat Mater. 2020;101:107606.
- Nad S, Gu Y. j, Asmussen J. Growth strategies for large and high quality single crystal diamond substrates. Diamond Relat Mater. 2015;60:26–34.
- Zhao Y, Guo Y, Lin L, et al. Comparison of the quality of single-crystal diamonds grown on two types of seed substrates by MPCVD. J Cryst Growth. 2018;491:89–96.
- Chatei H, Bougdira J, Rémy M, et al. Effect of nitrogen concentration on plasma reactivity and diamond growth in a H2-CH4-N2 microwave discharge. Diamond Relat Mater. 1997;6(1):107–119.
- Liao Y, Li CH, Ye ZY, et al. Analysis of optical emission spectroscopy in diamond chemical vapor deposition. Diamond Relat Mater. 2000;9(9/10):1716–1721.
- Sharma R, Woehrl N, Vrućinić M, et al. Effect of microwave power and C2 emission intensity on structural and surface properties of nanocrystalline diamond films. Thin Solid Films. 2011;519(22):7632–7637.
- Mankelevich YA, May P. W. New insights into the mechanism of CVD diamond growth: Single crystal diamond in MW PECVD reactors. Diamond Relat Mater. 2008;17(7–10):1021–1028.
- Liang Q, Chin CY, Lai J, et al. Enhanced growth of high quality single crystal diamond by microwave plasma assisted chemical vapor deposition at high gas pressures. Appl Phys Lett. 2009;94(2):024103.
- Hemawan KW, Hemley RJ. Optical emission diagnostics of plasmas in chemical vapor deposition of single-crystal diamond. J Vac Sci Technol A. 2015;33(6):061302.
- Bogdanov SA, Gorbachev AM, Vikharev AL, et al. Study of microwave discharge at high power density conditions in diamond chemical vapor deposition reactor by optical emission spectroscopy. Diamond Relat. Mater. 2019;97:107407.
- Mayr M, Fischer M, Klein O, et al. Interaction between surface structures and threading dislocations during epitaxial diamond growth. Phys Status Solidi A. 2015;212(11):2480–2486.
- Klein O, Mayr M, Fischer M, et al. Propagation and annihilation of threading dislocations during off-axis growth of heteroepitaxial diamond films. Diamond Relat Mater. 2016;65:53–58.
- Nad S, Charris A, Asmussen J. MPACVD growth of single crystalline diamond substrates with PCD rimless and expanding surfaces. Appl Phys Lett. 2016;109(16):162103.
- Tallaire A, Achard J, Brinza O, et al. Growth strategy for controlling dislocation densities and crystal morphologies of single crystal diamond by using pyramidal-shape substrates. Diamond Relat Mater. 2013;33:71–77.
- Brinza O, Achard J, Silva F, et al. Dependence of CVD diamond growth rate on substrate orientation as a function of process parameters in the high microwave power density regime. Phys Status Solidi A. 2008;205(9):2114–2120.
- Silva EL, Mishra YK, Fernandes AJS, et al. Direct Synthesis of Electrowettable Carbon Nanowall–Diamond Hybrid Materials from Sacrificial Ceramic Templates Using HFCVD. Adv Mater Interfaces. 2017;4(10):1700019.
- Ferrari AC, Robertson J. Origin of the 1150 cm−1 Raman mode in nanocrystalline diamond. Phys Rev B. 2001;63(12):121405.