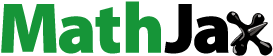
ABSTRACT
A composite material prepared from municipal solid waste-derived biochar and montmorillonite (MSW-BC-MMT) was studied as a remediation medium for the removal of oxytetracycline (OTC) from aqueous systems. The morphological characterization using SEM images revealed that the composite had a heterogeneous flaky, plate-like surface appearance. Based on N2 adsorption experiments and compared to the pristine biochar, the composite showed an increased specific surface area by 1.5 folds. The three-parameter Redlich Peterson isotherm model and two-parameter Temkin isotherm model were best fitted with R2 = 0.998 and 0.997, respectively, both for the pristine biochar and the MSW-BC-MMT composite. Additionally, the kinetics was best described using the pseudo-second-order model, suggesting that OTC adsorption onto the composite takes place via chemisorption. A capacity of 233 mg g−1 was observed for the MSW-BC-MMT, which is suggested to be predominantly governed by π-π electron donor–acceptor interactions, electrostatic attraction and surface complexation. As evidenced by FTIR results, OTC was presumed to be adsorbed via hydrogen bonding as well. The value addition of MSW by producing MSW-derived biochar is considered a favorable solution for solid waste management. Therefore, the production of the MSW-BC-MMT composite becomes useful due to its significance in acting as a simultaneous MSW management technique and a low-cost material for antibiotic OTC remediation.
GRAPHICAL ABSTRACT
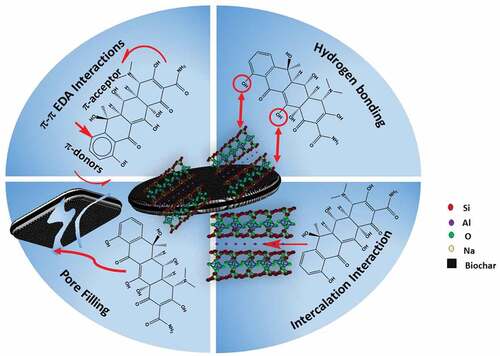
1. Introduction
The worldwide annual production of antibiotics amounts to a total of 100–200 Megatons. Antibiotics consist of a popular sub-class of pharmaceuticals that are used to remediate infections and improve immunity in living organisms, as well as to promote the growth and development of livestock (Martínez-Olivas et al., Citation2020). Due to their widespread application and the persistent nature, antibiotics and their metabolites are found to contaminate in wastewaters, soil and municipal sewage waste (Daghrir & Drogui, Citation2013; Zupanc et al., Citation2013). Prolonged exposure to small dosages of these contaminants can lead to deleterious health impacts, such as endocrine disruption, chronic toxicity and antibiotic resistance. Oxytetracycline (OTC) belongs to the class of antibiotics called tetracyclines (Chopra & Roberts, Citation2001). In 2003, OTC production made up 65% of the total global output in China. It is mainly used in animal farming applications and aquaculture that are mainly accountable for its low costs and high antibacterial activity (Yan et al., Citation2018). Almost 70–90% of the active ingredient and metabolites are released to the water environment because of its scarce adsorption rates by both humans and animals. Thereby, OTC is excreted in its active form from livestock, which in turn results in OTC contamination in soils through its application of livestock waste manure (Massé et al., Citation2014). For instance, recently, OTC concentration was the highest among the other antibiotics (30.7 ng L−1 in wastewater treatment plant effluents) found in the water of Chaobai riven in China (Y. Zhang et al., Citation2020). This was mainly due to the high consumption by farm animals, whereby greater than 3 tonnes of OTC were released into the environment (Chen et al., Citation2018). Intensive aquaculture studied in Korea also showed an increased level of OTC in the influent waters of concentration of about 9935 ng L−1, the highest compared to the other classes of antibiotics present in the stream (Choi et al., Citation2020). Due to these reasons and the highly hydrophilic nature of OTC leading to a high persistency in aqueous media, it is essential to remove OTC from the environment. Oxytetracycline can exist in its cationic, zwitterionic and anionic forms (pKa1 = 3.22; pKa2 = 7.46; pKa3 = 8.94; Luo et al., Citation2018; Premarathna et al., Citation2019). Oxytetracycline can remain in the soil up to 40 days, and its degradation depends on the pH, light and the ambient redox conditions (Y. Liu et al., Citation2016).
For the remediation of OTC, conventional biological methods or high costs physical-chemical treatments are used, whereby adsorption is considered as one of the highly efficient methods. Among the various known remediation applications, adsorption has gained an elevated research interest (Anastopoulos et al., Citation2019; Giannakoudakis et al., Citation2019) as it can lead to an efficient removal of pharmaceuticals contaminants, and especially antibiotics, from aquatic matrixes without producing harmful metabolites during the removal process (Peiris et al., Citation2019; Saroyan et al., Citation2019). Researchers covered adsorbents such as activated carbon, polymeric resins, clays, nanomaterials and even hydroxyapatite where they have shown significantly higher capacity for the remediation of varied antibiotics and other organic compounds. However, feasibility to extend over a large-scale operation suffers, as they incur high costs and are incapable of regeneration (Liang et al., Citation2019; Martínez-Olivas et al., Citation2020; Ramanayaka et al., Citation2020). Biomass-derived adsorbent, biochar (BC), has received a continual incremental focus as bio-adsorbents over the past few years (Ashiq et al., Citation2019). This was mainly attributed to its rich surface chemistry both in terms of heterogeneity and enhanced functional groups that facilitate adsorption of organic compounds, for instance, a higher aromaticity present in biochar (Ashiq et al., Citation2019; Peiris et al., Citation2017; Rajapaksha et al., Citation2016). Owing to the simplicity of the production process and the ubiquitous nature of the feedstock type used, biochar is considered as an economically viable substitute for other conventional adsorbents for remediating contaminants such as pharmaceuticals and their metabolites (Wathudura et al., Citation2020). Biochar-based adsorptive removal of OTC has been carried out previously using maize straw, cassava and corn stover with capacities of 26, 10 and 246.3 mg L−1, respectively (Aghababaei et al., Citation2017; M. Zhang et al., Citation2019). Generally, biochar is known to interact through π-π electron donor–acceptor (EDA) interactions due to higher aromaticity and proven to be advantageous for positive electrostatic interaction with antibiotics (Zhou et al., Citation2017). Therefore, multiple polar interactions are most probable with tetracycline-type antibiotics through their hydrophilic counterparts.
Another crucial perspective considering sustainability and environmental protection is the solid waste production from modern and developed societies. Solid waste management is a definite problem, and recently, and the disposal of municipal solid wastes (MSW) is of great concern (Wijesekara et al., Citation2014). Conversion of these solid wastes to biochar has proven to be feasible both in terms of economic and environmental standpoint. Most of the previous studies showed that the biochar derived from municipal solid wastes yields low specific surface area compared to other pyrolyzed biomasses, due to lower level of crucial compounds that are responsible for the formation of porosity during synthesis, lignin, for instance, present in the organic fractions of solid waste feedstock. However, within the context of the circular economy, the possibility of integrating the waste through pyrolysis and to design and develop novel synthetic protocols can be a way forward to reuse, recycle and convert solid waste to an adsorbent for pollutant mitigation. Modifications such as magnetization, acid modifications, base modifications and impregnation of clay minerals with biochar were attempted by various researchers to improve the adsorption capacity (De S. Dos Santos et al., Citation2021; Yao et al., Citation2014). Montmorillonite (MMT) is one such example of a ubiquitous, naturally occurring clay mineral. Its structure comprises of an octahedral sheet in between two tetrahedral sheets, making up a 2:1-type layered clay mineral (Jiang et al., Citation2013; Wu et al., Citation2010). The interlayer spaces of the mineral contain an equal amount of cations and anions, balancing out the charges (Dening et al., Citation2016). Montmorillonite has the ability to scavenge metal ions and organic contaminants owing to the presence of active sites on its surface, the high cation exchange capacity and the large specific surface area of the clay. These properties allow MMT to uptake higher amounts of OTC than other types of non-swelling clay types.
Moreover, the porous structure of biochar allows the clay particles to disperse on the biochar surface or in the pore interior of the biochar to produce clay mineral biochar composites. Thus, by combining the advantages of both biochar and clay minerals, increased adsorption performance can be harnessed (Premarathna et al., Citation2019). It was reported by Liang et al. that for cauliflower leaf-based BC-MMT composite, the capacity was 55.8 mg g−1 as compared to the capacity for raw biochar, 22.4 mg g−1 (Qiao et al., Citation2020). Based on the previously reported studies, no further investigations were reported about the modification of biochar–clay support to adsorb tetracycline-based antibiotics, and no detailed consideration about the effectiveness of the OTC removal by composites have been reported. Therefore, the overall goal of this study was to develop a novel biochar-clay-based adsorbent combining the advantage of both adsorbents: biochar and montmorillonite. Moreover, a research gap is created as the potential of MSW to be converted to the composite MSW-BC-MMT has not been explored in the recent studies. The presented research attempts to bridge this gap and will allow the production of MSW-BC-MMT composites to simultaneously address MSW management and the cost-effective remediation of OTC from aqueous systems.
2. Materials and methods
2.1. Reagents
All reagents used were of analytical grade. Oxytetracycline hydrochloride monohydrate was obtained from HIMEDIA Laboratories (India) of purity >95% and used without further purification. Montmorillonite, Cloisite-Na+ form, was obtained from Southern Clay (USA). The sodium hydroxide and nitric acid were obtained from Sigma Aldrich (USA).
2.2. Biochar–montmorillonite composite preparation
The partially dried municipal solid wastes (MSW) were collected from the Gohagoda Dumpsite, Kandy, Sri Lanka. The major portion of the waste rejection of MSW (raw material for biochar production) comprises coconut husks (36%) following by other organic matter (37%) and wood chips (11%) on a weight basis. Total perishable content was 89% while the non-biodegradable fraction was 11%, which included polythene, plastics and some stones. After separating the organic fraction, the MSW was pyrolyzed at 450 °C in a stepwise program at a heating rate of 15 °C min−1 for 4 hours inside a muffle furnace (Nabertherm, Germany) in an oxygen-free environment. The obtained pyrolyzed municipal solid wastes (MSW-BC) were cooled down before exposing it to the atmosphere to avoid the formation of ash. The biochar was then washed, oven-dried and sieved through a 2-mm mesh before utilizing it in subsequent experiments, to further eliminate any unwanted non-carbon materials that do not take part in the pyrolyzing, and further utilized for the batch experiments.
To obtain the composite, MMT suspension was obtained by adding 50 g of MMT into 2 L of deionized. The derived slurry was then shaken for 12 hours at 150 rpm at ambient conditions. About 50 g of MSW-BC that was prepared as above was added to the slurry and shaken for further 4 hours. Afterward, the clay–biochar slurry was washed, centrifuged, filtered, and oven-dried at 60 °C to obtain the MSW–biochar–montmorillonite composite (MSW-BC-MMT). The composite was then sieved through a 2-mm mesh before using it for further experiments.
2.3. Characterization of the MSW–biochar and MSW-BC-MMT composite
The crystalline structures were identified based on the powdered X-ray diffraction (PXRD) patterns, which were obtained using a Rigaku, Ultima IV diffractometer (Japan). It was operated at 40 kV and 40 mA, using the Cu Kα line at 1.54 Aᴼ as the radiation source. The surface chemical functionalities were estimated via a Fourier transform infrared (FTIR) spectrometer. The spectra were obtained using a Thermo Scientific, Nicolet iS10 spectrometer (USA) after scanning across 500 to 4000 cm−1. The infrared spectra were obtained for pristine materials and composites before and after OTC adsorption. The textural features were determined based on the isotherms obtained from the N2 physisorption tests using a N2 gas sorption analyzer, NOVA-1200, Quantachrome Corp (Boynton Beach, FL, USA). The specific surface area (SBET) was determined using the Brunauer–Emmett–Teller (BET) method. The morphology of the composite and biochar were studied using a Scanning electron microscope (SEM) Hitachi SU6600 (Japan). The ORION 210A pH meter was used for pH determinations.
2.4. Batch sorption experiments
To understand the effects of solution’s initial pH, reaction time and the OTC initial loading, batch adsorption experiments were conducted by varying each of the parameters independently. The effect of the solution initial pH on the adsorption of OTC was studied by adjusting the pH of the solution using HNO3 and NaOH (0.1 M) for the range of pH values between 4 and 9. The initial concentration of OTC was kept as 25 mg L−1 to study the optimum removal under different initial pH. Dosage of 2 g L−1 of each adsorbent was shaken with OTC for known time duration at 150 rpm. Once equilibrated, the final OTC concentration was determined using a Shimadzu UV160A UV/Visible spectrophotometer (Kyoto, Japan) at a wavelength of 278 nm. After recording the pH after equilibration, the solutions were centrifuged and filtered. OTC solution with a known concentration, without the adsorbent, was used as the control. Throughout the experiment, ultrapure water was used (resistivity of 18.3 MΩ) and in triplicate measurements. Replicas were performed for all the adsorption batch studies and the resulting concentrations, analyzed by UV/Visible spectrophotometer, were averaged and reported here. After the concentrations were deduced from the UV/Visible spectrophotometer, the efficiency of the adsorbents was determined in terms of the adsorption capacity , expressed in mg g−1. The removal efficiency against OTC and the adsorption capacity of the biochar adsorbents and their composites are quantified using the equation below:
Where is the volume of the solution (L),
is the weight of adsorbent used for the batch experiment (g), Ci and Cf are the initial and the final concentration (mg L−1), respectively. The kinetic and isotherm experiments were performed under the optimum pH conditions obtained from the edge experiment as above. The mechanism of adsorption was studied using stimulations of the experimental kinetic data obtained from the kinetic batch adsorption. The following are the models been used to simulate the experimental data. All the results obtained in this section are reported as the average of three duplicates performed and for each of the adsorbents prepared: MSW-BC and MSW-BC composite with MMT.
The adsorption kinetics were modeled using the pseudo-second-order (PSO) and the Elovich kinetic models to determine the mechanism of sorption ((2)-(3)). Adsorption kinetics of OTC on the MSW-BC-MMT composite was investigated at room temperature by using an adsorbent dosage of 2 g L−1 with an OTC concentration of 25 mg L−1 at room temperature. The contact times for the kinetic experiments were 10, 20, 40, 60, 120, 240, 480 and 960 minutes. At the end of each run, the final concentration was determined using the above-mentioned UV-Vis spectroscopy method.
Where is the adsorption at equilibrium (mg g−1),
is the adsorption over time (mg g−1),
(min−1) and
(g.mg−1.min−1) are the rate constant of the pseudo-first and second-order sorption, respectively, and
is the time in minutes (Ezzati, Citation2020).
The Elovich equation can be written as below:
Where is the initial adsorption rate (g.mg−1.min−1) and
is the adsorption constant (g.mg−1.min−1) [39]. Kinetic models simulate the diffusion pathways occurring on the MSW-biochar and composites, which represent the kinetics involved in the batches performed over a range of time.
Adsorption isotherms for the MSW-BC-MMT were determined by using a sorbent dosage of 2 g L−1 and by preparing an OTC concentration series with concentrations ranging in between 10 and 250 mg L−1. The vessels were shaken in a mechanical shaker at room temperature for 8 hours (as followed for the kinetic experiment). The experimental isotherm data were made to fit using non-linear models viz., Langmuir, Freundlich, Redlich-Peterson and Temkin model to provide for the base for the physio-chemical mechanisms and validating its applicability of the adsorption taking place between the solid–liquid interface. The adsorption parameters for OTC adsorption in this study were mainly determined using the Temkin and Redlich Peterson isotherm models.
The isotherm models and its related parameters are as follows ((4)-(6)):
Where is equilibrium concentration,
is the adsorption of CPX per gram of biochar sorbents (mg.g−1),
is the Langmuir constant associated with adsorption capacity at its maximum adsorption capacity (mg.g−1),
is the Langmuir equilibrium (L.mol−1), and
and
are Freundlich constant related to the non-linearity to the adsorption (Foo & Hameed, Citation2010).
(L g−1),
(constant) and
(mg−1) are the isotherm constant for the Redlich–Peterson isotherm equation (Redlich and Peterson, Citation1959).
Origin 2018 software was used for the modeling of all the equations using the non-linear curve fitting dialog, and the optimum adsorption capacities of the OTC were evaluated with both the equilibrium time and the concentrations. The right model was selected based on the correlation coefficient (R2), and the one that gets closest to 1 was chosen as the model for the particular experimental data series.
3. Results and discussion
3.1. Physicochemical characterizations of MSW-biochar and MSW-BC-MMT composite
The morphological features of municipal solid wastes-derived biochar (MSW-BC) and its composite with montmorillonite (MSW-BC-MMT) were examined using SEM imaging (Figure ). The surface of the MSW-BC sample showed an ordered template-like morphology, with well-organized uniform in size cylindrical-shaped cages-structure, which can be accountable to the heterogeneity of the varied wastes used during the preparation of the biochar, whereby a high amount of organic matter is present (). In the case of the composite material, the modification led to the appealing of the surface of the biochar, as well as to a “plate” and “flaky”-like structures, as observed in . This confirms the attachment of MMT with MSW-BC (S. Liu et al., Citation2019), further indicating the presence of inter-spatial layers in the synthesized composites as well that are attributed from the clays. Moreover, the ordered cylindrical cages appeared of wider diameter, a feature that can have a positive impact on the adsorption efficiency, due to a better penetration through the cages to the active adsorption centers (Giannakoudakis et al., Citation2019; Spagnoli et al., Citation2017).
The analysis of the textural features estimated from the nitrogen sorption (Table ) revealed two effects upon the composite formation; first, that the specific surface area (SBET) was almost doubled for the composite compared to the pristine biochar.
Table 1. BET surface analysis results and interlayer spacings (d001) in MSW-BC, MSW-BC-MMT and their changes with OTC interaction
3.1.1. Analysis of the PXRD spectra
The powder X-ray diffraction patterns (Figure ) revealed the amorphous nature of biochar, which is in good relation with the previous studies for the formation of biochar at a similar pyrolysis temperature of 450 °C (X. Zhang et al., Citation2013). The two individual ingredients of the composite, MMT and MSW-BC, presented their respective characteristic reflections. MSW-BC showed an amorphous nature with some reflections of low intensity to be linked to the presence of a trace amount of pure minerals. Montmorillonite showed various characteristic features of the mineral. In the case of the composite MSW-BC-MMT, the characteristic reflections linked to the MMT phase were shown, although the interlayer spacing was decreased to 12.4 A° from 15.5 A° of the pristine MMT. The incorporation with the amorphous MSW-BC and/or the synthetic protocol led to the expansion of the interfacial spacing of MMT. This is can be attributed to the intercalation of organic molecules released from the biochar derived from MSW into the interlayer space of montmorillonite. By virtue of binding the surface-active sites of the montmorillonite, which is swelling clay, the functional groups present in MSW-BC led to the grafting of the plate edges. Another key feature of the diffraction pattern as compared to the unmodified MMT and MSW-BC is that a new peak forms at 2Ɵ = 27.1 A° that corresponds to the isolation from the MMT when the composites are prepared, wherein the peak appeared to be more intense with the incorporation of the biochar substrate and the newer peak emerged with the treatment with OTC at 2Ɵ = 23.2 A°. The insertion of MSW-BC and OTC into the layers of the MMT through intercalation or/and exfoliation is achieved through the composite formation. However, the introduction of OTC to the composite leads to an increase in the interlayer spacing to 18.24 A°, thereby decreasing the basal reflection to a lower level, further sandwiching the interlamellar spaces. This can be attributable to the intercalation of OTC with the cations present in the interlayers of the montmorillonite. OTC with different functional molecules gets accommodated into the interlayer space of the MSW-BC-MMT composite. This is further confirmed from the infrared spectra with tricarbonyl amide anions of the zwitterionic state of OTC, interacting with the cations present in the interfacial layers of the clay, which makes it shrink to a significant extent. A previous study on clay-based adsorbents kaolinite, rectorite and palygorskite (Chang et al., Citation2009) for OTC removal reported similar observation for the increase and then decreased in the basal reflection.
3.1.1. Analysis of FTIR spectra
As shown in the FTIR spectra in , within the finger-print region from 1100 to 1700 cm−1, the OTC spectrum showed a plethora of bands, as reported also in previous works (Barbooti et al., Citation2014;). The band at 1448 cm−1 is attributed to the C= C bonds from the main structure of OTC, and the band at 1609 cm−1 is attributed to the C=O bond of the third ring. Furthermore, the band at 3080 cm−1 is ascribed to –OH bonds and they are observed at higher bands as well, which accounts for the induced hydrogen bonds occurring between the consecutive rings of the OTC (Jia et al., Citation2013). Dips at 1575 and 1545 cm−1 are characteristic bands of the –NH2 vibrations and amide –NH bonds, respectively (Yuan et al., Citation2019). The characteristic band of MMT at 3630 cm−1 was attributed to the vibrations of hydroxyl functionalities, while the stretching vibration of Si–O–Si was indicated at 1030 cm−1. Both these bands are characteristic and occurring due to the make-up of the pristine clay itself. However, for the synthesized adsorbent, the FTIR spectrum demonstrates the influence of montmorillonite on the biochar for the composite.
Figure 3. FTIR spectrum of pure (a) MSW-BC, MMT and OTC material along with the treated and untreated composites. (b) Enlarged spectrum from the wavelengths of 1200–1700 cm−1.
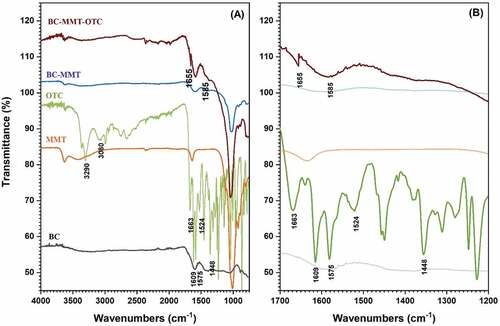
After OTC adsorption, most of the bands were unchanged, indicating the absence of drastic modifications made to the composite upon the interaction with OTC. However, there is a slight variation in the bands, which show fewer interactions of the functional groups with the composite material. Complex formation between active sites of clay minerals and OTC is evident by the band for IR shift from 1663 to 1655 cm−1. The intense bands of OTC given at 1609 and 1575 cm−1 due to the carbonyl group and the –NH group, respectively, were observed to disappear, which indicated a strong bond formation, presumably due to the formation of hydrogen bonds (). This observation is supported by literature published by several authors on tetracycline complexation onto clay-based adsorbents.
3.2. Batch adsorption studies
3.2.1. Edge experiments
The solution pH is an intricate factor that governs the involved interactions/mechanisms of adsorption as it influences the surface charge of the adsorbent and the degree of ionization of the adsorbate and as a direct result to affect the removal efficiency (Peiris et al., Citation2017). Even though the maximum adsorption capacity took place at two different pH, according to Figure , both MSW-BC and MSW-BC-MMT followed the same pattern, where the capacity was first seen to increase and then decrease. Pristine MSW-BC showed a maximum adsorption capacity at pH 7, and wherein for the MSW-BC-MMT composite, the maximum is at pH 6, consequently conclusive to the strong dependency of adsorption on the solution pH. Since the point of zero charges of MSW-BC was found to be 6.7, the surface is positive when pH < 6.7 and negative when pH > 6.7, thereby, corroborating with the result obtained for high adsorption at pH 6 for the composite.
Contrastingly, the isomorphic substitution in clay layers and variable charges in edge sites of the montmorillonite make the MSW-BC-MMT composite surface to be negative over a wide pH range (Jayawardhana et al., Citation2019; Spagnoli et al., Citation2017). However, tetracycline-type antibiotics, due to the simultaneous deprotonation and protonation of the dimethyl-amino group and the phenolic-diketone group, respectively, get adsorbed by negatively or positively charged adsorbent using cation exchange, surface complexation or through interfacial intercalation with the presence of the montmorillonite present in the composite. Considering the speciation of OTC, at pH below 3.3, OTC exists predominantly in the cationic form. Upon increasing the pH from 3.3 to about 7.3, the fraction of OTC that exists in the zwitterion form gradually increases. The anionic species of the OTC become predominant after pH 7.3 (Aghababaei et al., Citation2017). This allows for the selective adsorption of the anionic species, i.e. tricarbonyl amide from the zwitterionic state of the OTC on the positively charged adsorbent, which is the MSW–biochar present in the pristine and composite counterpart (Ramanayaka et al., Citation2020). In addition, OTC predominantly binds with the oppositely charged fractions of the adsorbent surface through physisorption process, viz. electrostatic interactions or van der Wall’s. This interaction is caused by the combined effects arising from both the biochar surface and the clay of the composite, allowing a selective adsorption to take place (Almasri et al., Citation2019; Jalil et al., Citation2017; Ramanayaka et al., Citation2020).
In general, it can be concluded that the adsorption capacity for both MSW-BC and MSW-BC-MMT showed increasing capacities within pH 4–7, a trend in good relation with the results presented in the literature (Peiris et al., Citation2017; Rivera-Utrilla et al., Citation2013). Additionally, the formation of zwitterionic species and the change in MSW-BC surface charge as a function of pH could promote the formation of electrostatic attractions as well (Peiris et al., Citation2017). When the solution pH is basic, interactions with both the MSW-BC and the composite becomes repulsive due to strong electrostatic interactions with the OTC analyte that exists in the anionic form, thereby decreasing the adsorption capacity significantly (Lian et al., Citation2013). Despite the repulsive forces, the composite depicted higher adsorption capacities after pH 7, which could be attributed to the interfacial intercalation between the crystal structures of the montmorillonite clay present in the MSW-BC-MMT composite. This result is further confirmed with the PXRD spectra through the basal reflection, as exemplified earlier (Peiris et al., Citation2017).
3.2.2. Inferences from isotherm and kinetic fittings
The increase in OTC sorption affinity on the composite was increased with increasing concentration of OTC as was depicted in Figure . Though MSW-BC-MMT demonstrated inclined affinity with increasing OTC concentration followed by equilibrium, MSW-BC did not reach equilibrium with increasing OTC loading. The maximum sorption for MSW-BC-MMT was reached when the initial concentration was 300 mg L−1 (Figure ). As far as finding an optimum performance of the synthesized adsorbents is concerned, an optimum range was observed for interactions for over 12 hours. However, the adsorption continues for the new biochar material with an increase in the initial concentrations, thereby not achieving an equilibrium. Nevertheless, the performance was better for the composite, and the optimum value is known to be proceeding for the real-time applications, unlike the pristine material where the optimum is unknown.
Figure 5. Redlich–Peterson and Temkin isotherm models for the adsorption of OTC by MSW-BC-MMT composite.
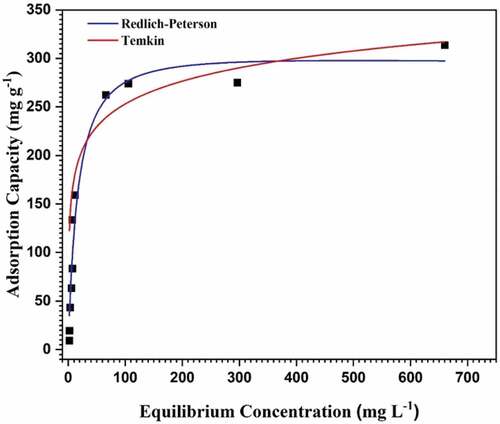
The Redlich–Peterson and Temkin isotherm model was the best-fitted (R2 = 0.998 and 0.997, respectively) for MSW-BC-MMT composite (Table ). Redlich–Peterson is inclusive of both Langmuir and Freundlich isotherm models. Hence, the adsorption of OTC occurred on to heterogeneous surfaces of the adsorbents (Yao et al., Citation2014). The value of constant obtained in the Redlich–Peterson model is closer to unity, implying that the most plausible mechanism for a monolayer coverage of OTC adsorption onto the synthesized composite as depicted in the Langmuir isotherm, which is a particular case of Redlich–Peterson when the constant is closer to one (Figure ; Ho & Ofomaja, Citation2006). However, the Temkin isotherm model described the effects of indirect OTC loading on the composite for adsorption process where the heat of adsorption is taken into account to signify the binding energies in the monolayer layer as postulated would have a linear declination due to an increase in the dosage as was taken up to 800 mg L−1 in this study.
Table 2. Kinetic and isotherm model parameters for the adsorption of OTC onto MSW-BC-MMT
Influence of time for the MSW-BC-MMT composite was studied up to 24 hours as shown in Figure , wherein rapid adsorption was observed for the first 170 minutes, obtaining an adsorption capacity of 86 mg g−1, which is due to the easily available vacant sites for OTC to bind on before it gets saturated. The removal of OTC is further equilibrated after 220 minutes. Kinetic data of MSW-BC-MMT composite was best fitted to the pseudo-second-order model (R2 = 0.999; Figure and Table ), where both Elovich and pseudo-second-order were also fitted. Elovich kinetic fit (Figure ) gave the least R2 significantly compared to pseudo-second-order kinetic models. Thus, due to the high heterogeneity of the synthesized adsorbent, OTC adsorption occurred predominantly via chemisorption due to the varied functional groups present in both the composite and antibiotic, which compensate for many governing electrostatic interactions as exemplified in the sections below (Premarathna et al., Citation2019).
4. Adsorption mechanism
The proposed interactions/removal mechanisms of OTC adsorption by both MSW-BC and MSW-BC-MMT are collected in Figure . Since OTC is pH-dependent and hence its charge alters, the mode of adsorption solely depends on the surface charge for possible electrostatic interactions occurred. OTC is easily protonated at pH<3.5, where the dimethyl-amino group exists in cationic forms and beyond pH 7.5, OTC predominantly exists as an anionic form. MSW-BC-MMT composite is capable of interacting with OTC through two distinct modes: (i) interfacial intercalation interactions attributed to the clay counterpart (Jiang et al., Citation2013); (ii) surface complexation both attributing from the biochar counterpart (Song et al., Citation2020).
Figure 7. Conceptual illustration of the possible mechanisms of OTC adsorption on MSW-BC-MMT composite.
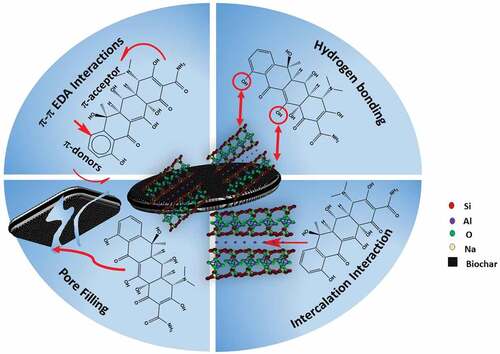
As reported in the ultimate and the proximate analysis by Jayawardhana et al. (Jayawardhana et al., Citation2019), a lower H/C ratio was observed, which indicated a higher carbonized structure and the surface charges through the zeta-potential (point of zero charges) was along with the neutral range (6.7), which further facilitates the adsorption of OTC (both OTC+ and OTC −) as was confirmed in the characterization results. Thus, from the results obtained from the adsorption batches, the cationic forms of OTC bind electrostatically with negatively charged MSW-BC surface through π-π electron donor–acceptor (EDA) interactions attributing from the existing nature of the biochar as was exemplified in the pH edge results (Ge et al., Citation2020). The adsorption isotherm fitting results obtained from Reldich–Peterson and Temkin models concluded that the adsorption occurred as a result of both physisorption and chemisorption processes. The multilayer adsorption process can, thus, be postulated due to the heterogeneous nature of the composite.
These results corroborated with the results obtained in the FTIR spectra, wherein small modifications after adsorption appeared in the spectra whose bands belong to the functional groups of OTC. This spectrum is not seen in the pristine MSW-BC, especially for the band from 1663 to 1655 cm−1 for the composite, indicating a complex formation between the charged OTC and the adsorbents. PXRD spectra results show the expansion of interlayer for the synthesized MSW-BC and compression of the interlayer for the composite, as exemplified earlier. Thus, from both FTIR and PXRD spectra, the ketone carbonyl group present in the OTC was directly coordinated with the interlayer cations present in the montmorillonite as shown by the reduction of the basal reflection shown in the X-ray spectra and similar results corroborated in Kulshrestha et al. (Citation2004). The carbonyl group present in the OTC is also postulated to create a hydrogen bond with the hydroxyl group of the hydrated biochar surfaces and further coordinating to the interlayer cation. The multi-functionality of OTC makes it easy to combine with the composites for improved removal and thus providing more adsorptive sites. This is demonstrated by Aristilde et al., Citation2016), wherein the expansion and compression of interlayer space after adsorption of OTC antibiotic has resulted in the presence of montmorillonite in the composite (Figure ).
5. Conclusions
The production of biochar using municipal solid waste contributes to solid waste management. Going a step further, the utilization of this biochar (MSW-BC) toward the creation of a composite (MSW-biochar-MMT) with montmorillonite led to a material of a high removal efficiency against oxytetracycline from aqueous systems. According to the results obtained, the increased efficiency of OTC adsorption onto the composite was due to high surface chemical heterogeneity, elevated specific surface area and interlayer spacing resulting from the incorporation of MMT to MSW-BC. Electrostatic interactions, hydrogen bonding and π-π electron donor–acceptor interactions are the possible involved interactions/adsorption mechanisms. The pseudo-second-order model and Elovich kinetic models were used to describe the kinetics of adsorption and provided evidence for the existence of chemical bonding. Isotherm models dictated that Redlich Peterson model as the best fit from which the capacity of adsorption was deduced to be 232.5 mg g−1. MSW-BC-MMT composite have proven advantageous over the other traditional adsorbents based on these results obtained: (i) the method followed to synthesized MSW-BC-MMT is less complex, environmentally friendly and low cost on the chemicals used. The source of biochar can be obtained from waste biomass as agricultural residues, etc., whereby re-utilizing waste. MSW-BC-MMT composite becomes a potential low-cost adsorbent while providing a viable solution for solid waste management as well; (ii) treatment process is simple stirring technique and easily operated based on the concentrations; (iii) composites offered excellent OTC removal ability compared to their pristine counterpart. Therefore, it can be seen that MSW-BC-MMT holds the potential for remediating antibiotic contaminated natural water samples in the future.
PUBLIC INTEREST STATEMENT
Antibiotics are widely used in human medicine and aquaculture and, hence, are found in the environmental waters due to excretion and manure use. They are potentially able to produce adverse effects to aquatic organisms and have negative impacts on human health by developing antibiotic resistance, and therefore, the removal of antibiotics is difficult due to their stability. This research project aims to remove oxytetracycline hydrochloride (OTC), which is a common antibiotic used in human and veterinary farms, via adsorption process using a composite developed using municipal solid waste-derived biochar and clay mineral (Montmorillonite). This composite material was able to remove OTC efficiently at pH 6 via adsorption as a multilayer process. This composite has proven advantageous over the other traditional adsorbents due to low cost, simple and environmental friendliness. Simultaneously, the utilization of municipal solid waste for the production of this biochar composite contributes to sustainable solid waste management.
Acknowledgements
This work was carried out with the support of the Research Council (ASP/01/RE/SCI/2017/83), Faculty of Applied Sciences, University of Sri Jayewardenepura, Sri Lanka. Analytical support from the Instrument Centre, Faculty of Applied Sciences, University of Sri Jayewardenepura, Sri Lanka, is also acknowledged.
Disclosure statement
No potential conflict of interest was reported by the author(s).
Correction Statement
This article has been corrected with minor changes. These changes do not impact the academic content of the article.
Additional information
Funding
Notes on contributors
Meththika Vithanage
Vithanage group of the Ecosphere Resilience Research Centre (ERRC), University of Sri Jayewardenepura, led by Prof. Meththika Vithanage focuses on research on atmosphere, water, soil and waste. The presence of contaminants in emerging concern (CECs) in aqueous environments and other contaminants such as trace metals, herbicides, etc. that comes from the anthropogenic origin are increasing their prevalent use in the fast-moving urban economy and “consumerism” society. These contaminants get leached into the environment and water where treatment gets costly or involve in re-formulation into other dangerous compounds. At ERRC, we monitor water and wastewaters for their quality, especially drinking water and wastewaters like landfill leachate and hospital wastewater. We further focus at the material level for remediating contaminants from water, i.e. trace metals, dyes, antibiotics, pesticides etc. Surface modification of bio-geo-based sorbents selective for both organic and inorganic contaminant uptake is where our expertise lies.
References
- Aghababaei, A., Ncibi, M. C., & Sillanpää, M. (2017). Optimized removal of oxytetracycline and cadmium from contaminated waters using chemically-activated and pyrolyzed biochars from forest and wood-processing residues. Bioresource Technology, 239 , 28–36. https://doi.org/10.1016/j.biortech.2017.04.119
- Almasri, D. A., Saleh, N. B., Atieh, M. A., McKay, G., & Ahzi, S. (2019). Adsorption of phosphate on iron oxide doped halloysite nanotubes. Scientific Reports, 9(1), 1–13. https://doi.org/10.1038/s41598-019-39035-2
- Anastopoulos, I., Robalds, A., Tran, H. N., Mitrogiannis, D., Giannakoudakis, D. A., Hosseini-Bandegharaei, A., & Dotto, G. L. (2019). Removal of heavy metals by leaves-derived biosorbents. Environmental Chemistry Letters, 17(2), 755–766. https://doi.org/10.1007/s10311-018-00829-x
- Aristilde, L., Lanson, B., Miéhé-Brendlé, J., Marichal, C., & Charlet, L. (2016). Enhanced interlayer trapping of a tetracycline antibiotic within montmorillonite layers in the presence of Ca and Mg. Journal of Colloid and Interface Science, 464, 153–159. https://doi.org/10.1016/j.jcis.2015.11.027
- Ashiq, A., Sarkar, B., Adassooriya, N., Walpita, J., Rajapaksha, A. U., Ok, Y. S., & Vithanage, M. (2019). Sorption process of municipal solid waste biochar-montmorillonite composite for ciprofloxacin removal in aqueous media. Chemosphere, 236, 124384. https://doi.org/10.1016/j.chemosphere.2019.124384
- Barbooti, M. M., Su, H., Punamiya, P., & Sarkar, D. (2014). Oxytetracycline sorption onto Iraqi montmorillonite. International Journal of Environmental Science and Technology, 11(1), 69–76. https://doi.org/10.1007/s13762-013-0361-6
- Chang, P. H., Li, Z., Yu, T.-L., Munkhbayer, S., Kuo, T.-H., Hung, Y.-C., Jean, J.-S., & Lin, K.-H. (2009). Sorptive removal of tetracycline from water by palygorskite. Journal of Hazardous Materials, 165(1–3), 148–155. https://doi.org/10.1016/j.jhazmat.2008.09.113
- Chen, H., Jing, L., Teng, Y., Wang, J. (2018). Multimedia fate modeling and risk assessment of antibiotics in a water-scarce megacity. Journal of Hazardous Materials, 348(August 2017), 75–83. https://doi.org/10.1016/j.jhazmat.2018.01.033
- Choi, S., Sim, W., Jang, D., Yoon, Y., Ryu, J., Oh, J., Woo, J.-S., Kim, Y. M., & Lee, Y. (2020). Antibiotics in coastal aquaculture waters: Occurrence and elimination efficiency in oxidative water treatment processes. Journal of Hazardous Materials, 396(March), 122585. https://doi.org/10.1016/j.jhazmat.2020.122585
- Chopra, I., & Roberts, M. (2001). Tetracycline antibiotics: Mode of action, applications, molecular biology, and epidemiology of bacterial resistance. Microbiology and Molecular Biology Reviews, 65(2), 232–260. https://doi.org/10.1128/mmbr.65.2.232-260.2001
- Daghrir, R., & Drogui, P. (2013). Tetracycline antibiotics in the environment: A review. Environmental Chemistry Letters, 11(3), 209–227. https://doi.org/10.1007/s10311-013-0404-8
- de S. Dos Santos, G. E., Lins, P. V. D. S., Oliveira, L. M. T. D. M., Silva, E. O. D., Anastopoulos, I., Erto, A., Giannakoudakis, D. A., Almeida, A. R. F. D., Duarte, J. L. D. S., & Meili, L. (2021). Layered double hydroxides/biochar composites as adsorbents for water remediation applications: Recent trends and perspectives. Journal of Cleaner Production, 284, 124755. https://doi.org/10.1016/j.jclepro.2020.124755
- Dening, T. J., Joyce, P., Rao, S., Thomas, N., & Prestidge, C. A. (2016). Nanostructured montmorillonite clay for controlling the lipase-mediated digestion of medium chain triglycerides. ACS Applied Materials and Interfaces, 8(48), 32732–32742. https://doi.org/10.1021/acsami.6b13599
- Ezzati, R. (2020). Derivation of pseudo-first-order, pseudo-second-order and modified pseudo-first-order rate equations from Langmuir and Freundlich isotherms for adsorption. Chemical Engineering Journal, 392(July), 123705. https://doi.org/10.1016/j.cej.2019.123705
- Foo, K. Y., & Hameed, B. H. (2010). Insights into the modeling of adsorption isotherm systems. Chemical Engineering Journal, 156(1), 2–10. https://doi.org/10.1016/j.cej.2009.09.013
- Ge, S., Foong, S. Y., Ma, N. L., Liew, R. K., Wan Mahari, W. A., Xia, C., Yek, P. N. Y., Peng, W., Nam, W. L., Lim, X. Y., Liew, C. M., Chong, C. C., Sonne, C., & Lam, S. S. (2020). Vacuum pyrolysis incorporating microwave heating and base mixture modification: An integrated approach to transform biowaste into eco-friendly bioenergy products. Renewable and Sustainable Energy Reviews, 127(February), 109871. https://doi.org/10.1016/j.rser.2020.109871
- Giannakoudakis, D. A., Barczak, M., Florent, M., & Bandosz, T. J. (2019). Analysis of interactions of mustard gas surrogate vapors with porous carbon textiles. Chemical Engineering Journal, 362, 758–766. https://doi.org/10.1016/j.cej.2019.01.064
- Ho, Y.-S., & Ofomaja, A. E. (2006). Biosorption thermodynamics of cadmium on coconut copra meal as biosorbent. Biochemical Engineering Journal, 30(2), 117–123. https://doi.org/10.1016/j.bej.2006.02.012
- Jalil, M. E. R., Baschini, M., & Sapag, K. (2017). Removal of ciprofloxacin from aqueous solutions using pillared clays. Materials, 10(12), 17–19. https://doi.org/10.3390/ma10121345
- Jayawardhana, Y., Gunatilake, S. R., Mahatantila, K., Ginige, M. P., & Vithanage, M. (2019). Sorptive removal of toluene and m-xylene by municipal solid waste biochar: Simultaneous municipal solid waste management and remediation of volatile organic compounds. Journal of Environmental Management, 238, 323–330. https://doi.org/10.1016/j.jenvman.2019.02.097
- Jia, M., Wang, F., Bian, Y., Jin, X., song, Y., Kengara, F. O., Xu, R., & Jiang, X. (2013). Effects of pH and metal ions on oxytetracycline sorption to maize-straw-derived biochar. Bioresource Technology, 136, 87–93. https://doi.org/10.1016/j.biortech.2013.02.098
- Jiang, W. T., Wang, C. J., & Li, Z. (2013). Intercalation of ciprofloxacin accompanied by dehydration in rectorite. Applied Clay Science, 74, 74–80. https://doi.org/10.1016/j.clay.2012.07.009
- Kulshrestha, P., Giese, R. F., & Aga, D. S. (2004). Investigating the molecular interactions of oxytetracycline in clay and organic matter: Insights on factors affecting its mobility in soil. Environmental Science and Technology, 38(15), 4097–4105. https://doi.org/10.1021/es034856q
- Lian, F., Song, Z., Liu, Z., Zhu, L., & Xing, B. (2013). Mechanistic understanding of tetracycline sorption on waste tire powder and its chars as affected by Cu2+ and pH. Environmental Pollution, 178, 264–270. https://doi.org/10.1016/j.envpol.2013.03.014
- Liang, G., Wang, Z., Yang, X., Qin, T., Xie, X., Zhao, J., & Li, S. (2019). Efficient removal of oxytetracycline from aqueous solution using magnetic montmorillonite-biochar composite prepared by one step pyrolysis. Science of the Total Environment, 695, 133800. https://doi.org/10.1016/j.scitotenv.2019.133800
- Liu, Y., He, X., Fu, Y., & Dionysiou, D. D. (2016). Kinetics and mechanism investigation on the destruction of oxytetracycline by UV-254 nm activation of persulfate. Journal of Hazardous Materials, 305, 229–239. https://doi.org/10.1016/j.jhazmat.2015.11.043
- Liu, S., Liu, Y., Jiang, L., Zeng, G., Li, Y., Zeng, Z., Wang, X., & Ning, Q. (2019). Removal of 17β-Estradiol from water by adsorption onto montmorillonite-carbon hybrids derived from pyrolysis carbonization of carboxymethyl cellulose. Journal of Environmental Management, 236(January), 25–33. https://doi.org/10.1016/j.jenvman.2019.01.064
- Luo, J., Li, X., Ge, C., Müller, K., Yu, H., Huang, P., Li, J., Tsang, D. C. W., Bolan, N. S., Rinklebe, J., & Wang, H. (2018). Sorption of norfloxacin, sulfamerazine and oxytetracycline by KOH-modified biochar under single and ternary systems. Bioresource Technology, 263, 385–392. https://doi.org/10.1016/j.biortech.2018.05.022
- Martínez-Olivas, A., Torres-Pérez, J., Balderas-Hernández, P., Reyes-López, S. Y. (2020). Oxytetracycline sorption onto synthetized materials from hydroxyapatite and aluminosilicates. Water, Air, and Soil Pollution, 231(6), 1–21. https://doi.org/10.1007/s11270-020-04638-3
- Massé, D. I., Saady, N. M. C., & Gilbert, Y. (2014). Potential of biological processes to eliminate antibiotics in livestock manure: An overview. Animals, 4(2), 146–163. https://doi.org/10.3390/ani4020146
- Peiris, C., Gunatilake, S. R., Mlsna, T. E., Mohan, D., & Vithanage, M. (2017). Biochar based removal of antibiotic sulfonamides and tetracyclines in aquatic environments: A critical review. Bioresource Technology, 246(May), 150–159. https://doi.org/10.1016/j.biortech.2017.07.150
- Peiris, C., Nayanathara, O., Navarathna, C. M., Jayawardhana, Y., Nawalage, S., Burk, G., Karunanayake, A. G., Madduri, S. B., Vithanage, M., Kaumal, M., Mlsna, T. E., Hassan, E. B., Abeysundara, S., Ferez, F., & Gunatilake, S. R. (2019). The influence of three acid modifications on the physicochemical characteristics of tea-waste biochar pyrolyzed at different temperatures: A comparative study. RSC Advances, 9(31), 17612–17622. https://doi.org/10.1039/c9ra02729g
- Premarathna, K. S. D., Rajapaksha, A. U., Adassoriya, N., Sarkar, B., Sirimuthu, N. M. S., Cooray, A., Ok, Y. S., & Vithanage, M. (2019). Clay-biochar composites for sorptive removal of tetracycline antibiotic in aqueous media. Journal of Environmental Management, 238, 315–322. https://doi.org/10.1016/j.jenvman.2019.02.069
- Qiao, L., Liu, D., Yuan, X., Wang, Q., Ma, Q. (2020). Generation and prediction of construction and demolitionwaste using exponential smoothing method: A case study of Shandong Province, China. Sustainability (Switzerland), 12(12). https://doi.org/10.3390/su12125094
- Rajapaksha, A. U., Chen, S. S., Tsang, D. C. W., Zhang, M., Vithanage, M., Mandal, S., Gao, B., Bolan, N. S., & Ok, Y. S. (2016). Engineered/designer biochar for contaminant removal/immobilization from soil and water: Potential and implication of biochar modification. Chemosphere, 148, 276–291. https://doi.org/10.1016/j.chemosphere.2016.01.043
- Ramanayaka, S., Sarkar, B., Cooray, A. T., Ok, Y. S., & Vithanage, M. (2020). Halloysite nanoclay supported adsorptive removal of oxytetracycline antibiotic from aqueous media. Journal of Hazardous Materials, 384, 121301. https://doi.org/10.1016/j.jhazmat.2019.121301
- Redlich, O. J. D. L., & Peterson, D. L. (1959). A useful adsorption isotherm. Journal of physical chemistry, 63(6), 1024–1024.
- Rivera-Utrilla, J., Gómez-Pacheco, C. V., Sánchez-Polo, M., López-Peñalver, J. J., & Ocampo-Pérez, R. (2013). Tetracycline removal from water by adsorption/bioadsorption on activated carbons and sludge-derived adsorbents. Journal of Environmental Management, 131, 16–24. https://doi.org/10.1016/j.jenvman.2013.09.024
- Saroyan, H. S., Bele, S., Giannakoudakis, D. A., Samanidou, V. F., Bandosz, T. J., & Deliyanni, E. A. (2019). Degradation of endocrine disruptor, bisphenol-A, on an mixed oxidation state manganese oxide/modified graphite oxide composite: A role of carbonaceous phase. Journal of Colloid and Interface Science, 539, 516–524. https://doi.org/10.1016/j.jcis.2018.12.088
- Song, J., Zhang, S., Li, G., Du, Q., & Yang, F. (2020). Preparation of montmorillonite modified biochar with various temperatures and their mechanism for Zn ion removal. Journal of Hazardous Materials, 391, 121692. https://doi.org/10.1016/j.jhazmat.2019.121692
- Spagnoli, A. A., Giannakoudakis, D. A., & Bashkova, S. (2017). Adsorption of methylene blue on cashew nut shell based carbons activated with zinc chloride: The role of surface and structural parameters. Journal of Molecular Liquids, 229, 465–471. https://doi.org/10.1016/j.molliq.2016.12.106
- Wathudura, P. D., Peiris, C., Navarathna, C. M., Mlsna, T. E., Kaumal, M. N., Vithanage, M., & Gunatilake, S. R. (2020). Microwave and open vessel digestion methods for biochar. Chemosphere, 239, 124788. https://doi.org/10.1016/j.chemosphere.2019.124788
- Wijesekara, S. S. R. M. D. H. R., Mayakaduwa, S. S., Siriwardana, A. R., de Silva, N., Basnayake, B. F. A., Kawamoto, K., & Vithanage, M. (2014). Fate and transport of pollutants through a municipal solid waste landfill leachate in Sri Lanka. Environmental Earth Sciences, 72(5), 1707–1719. https://doi.org/10.1007/s12665-014-3075-2
- Wu, Q., Li, Z., Hong, H., Yin, K., & Tie, L. (2010). Adsorption and intercalation of ciprofloxacin on montmorillonite. Applied Clay Science, 50(2), 204–211. https://doi.org/10.1016/j.clay.2010.08.001
- Yan, W., Guo, Y., Xiao, Y., Wang, S., Ding, R., Jiang, J., Gang, H., Wang, H., Yang, J., & Zhao, F. (2018). The changes of bacterial communities and antibiotic resistance genes in microbial fuel cells during long-term oxytetracycline processing. Water Research, 142, 105–114. https://doi.org/10.1016/j.watres.2018.05.047
- Yao, Y., Gao, B., Fang, J., Zhang, M., Chen, H., Zhou, Y., Creamer, A. E., Sun, Y., & Yang, L. (2014). Characterization and environmental applications of clay-biochar composites. Chemical Engineering Journal, 242, 136–143. https://doi.org/10.1016/j.cej.2013.12.062
- Yuan, L., Yan, M., Huang, Z., He, K., Zeng, G., Chen, A., Hu, L., Li, H., Peng, M., Huang, T., & Chen, G. (2019). Influences of pH and metal ions on the interactions of oxytetracycline onto nano-hydroxyapatite and their co-adsorption behavior in aqueous solution. Journal of Colloid and Interface Science, 541, 101–113. https://doi.org/10.1016/j.jcis.2019.01.078
- Zhang, Y., Chen, H., Jing, L., & Teng, Y. (2020). Ecotoxicological risk assessment and source apportionment of antibiotics in the waters and sediments of a peri-urban river. Science of the Total Environment, 731, 139128. https://doi.org/10.1016/j.scitotenv.2020.139128
- Zhang, M., Meng, J., Liu, Q., Gu, S., Zhao, L., Dong, M., Zhang, J., Hou, H., & Guo, Z. (2019). Corn stover-derived biochar for efficient adsorption of oxytetracycline from wastewater. Journal of Materials Research, 34(17), 3050–3060. https://doi.org/10.1557/jmr.2019.198
- Zhou, Y., Liu, X., Xiang, Y., Wang, P., Zhang, J., Zhang, F., Wei, J., Luo, L., Lei, M., & Tang, L. (2017). Modification of biochar derived from sawdust and its application in removal of tetracycline and copper from aqueous solution: Adsorption mechanism and modelling. Bioresource Technology, 245(July 2018), 266–273. https://doi.org/10.1016/j.biortech.2017.08.178
- Zupanc, M., Kosjek, T., Petkovšek, M., Dular, M., Kompare, B., Širok, B., Blažeka, Ž., & Heath, E. (2013). Removal of pharmaceuticals from wastewater by biological processes, hydrodynamic cavitation and UV treatment. Ultrasonics Sonochemistry, 20(4), 1104–1112. https://doi.org/10.1016/j.ultsonch.2012.12.003
- Zupanc, M., Kosjek, T., Petkovšek, M., Dular, M., Kompare, B., Širok, B., and Heath, E. (2013). Removal of pharmaceuticals from wastewater by biological processes, hydrodynamic cavitation and UV treatment. Ultrasonics sonochemistry, 20(4), 1104–1112.