ABSTRACT
Although the role of plants in wetland ecosystems is largely known, not many native plant species have been used in constructed wetlands, especially in sub-Saharan Africa to treat oilfield wastewater, an emerging contaminant of considerable concern to both academics and policy makers owing to its deleterious effects on aquatic organisms and human health. This study assessed the contaminant removal efficiencies of three indigenous wetland plants (Typha latifolia, Ruellia simplex and Alternanthera philoxeroides) in a small-field-scale free water surface flow constructed wetland to polish effluent treated in a conventional oily wastewater treatment plant. Statistical significant differences in removal efficiencies were recorded between the planted wetlands and the unplanted wetland for all parameters examined, with the exception of total phosphorus. The results also showed that the removal efficiencies for oil and grease, biochemical oxygen demand, chemical oxygen demand and total dissolved solids were highest in wetland planted with Alternanthera philoxeroides followed by Typha latifolia planted wetland. Whereas the removal of nitrate and total phosphorus were highest in wetland planted with Typha latifolia, the removal of coliform bacteria was highest in Ruellia simplex planted wetland. The study showed that free water surface flow constructed wetland has the potential to improve quality of effluent treated with conventional oily wastewater treatment plant. The study is very significant because it has extensive practical application in the oil and gas industry in terms of improving the contaminant removal efficiency and thereby limiting adverse environmental and human health impacts. Also, wetland treatment systems are nature-based solution to environmental problems because they are built on sound ecological principles and for that matter they are sustainable in terms of the capital cost involved, energy consumption and technological requirement.
Introduction
Poorly treated wastewater is a common source of water pollution and often has serious effects on human health and other organisms because it contains chemicals, nutrients and microbial contaminants at concentrations that are harmful to biodiversity, especially in aquatic ecosystems (Corcoran, Citation2010; Calheiros et al., Citation2007; Yadav et al., Citation2019). In particular, developing countries are faced with a serious challenge of wastewater management that has resulted in large volumes of partially, or untreated wastewater being released into the environment (Alufasi et al., Citation2017; Arthur et al., Citation2005;Babatunde et al., Citation2008). Oilfields wastewater treatment is required to meet disposal regulatory limits or to meet beneficial use standard. Cooper and Findlater (Citation1999), Saravanan et al. (Citation2011) and Vymazal et al. (Citation1998) all point out that different types of macrophyte-based wastewater treatment systems can be combined with conventional treatment plants to exploit the advantages of each system to polish effluents from conventional wastewater treatment plants. Constructed wetlands are artificial ecological sanitation technologies that mimic closely the structure of natural wetlands for the treatment of wastewater (Yang et al., Citation2007). Treatment wetlands represent an alternative or an add on to conventional wastewater treatment systems for management of various types of wastewater including municipal wastewater, household wastewater and industrial wastewater (Brix, Citation2003; Cooper, Citation2005; Crites et al., Citation2005; Gabr, Citation2022; Licata et al., Citation2019; Igunnu & Chen, Citation2014).
Macrophytes are an important component of wetlands and their presence or absence is used to characterise and define the boundaries of wetlands (Brix, Citation2003Rodriguez-Dominguez et al., Citation2022). Plants are known to be crucial influencing factors to the removal efficiency in constructed wetland because they are considered to be the main biological component and change directly or indirectly the primary removal processes of pollutants over time (Vymazal et al., Citation2021; Wu et al., Citation2015). They perform key functions in wetlands in relation to wastewater treatment. These include the provision of good conditions for physical filtration, regulation of hydraulic velocity, prevention of vertical flow systems from clogging and provision of huge surface area for microbial attachment and growth. Not that only, wetland macrophytes mediate transfer of oxygen to the rhizosphere by leakage from roots which increases aerobic degradation of organic matter and nitrification of nitrogenous compounds and plants’ uptake of nutrients in low-loaded systems (surface flow systems; Fan et al., Citation2021; Vymazal et al., Citation2021; Brix, Citation2003).
Plants have also been noted to play important role in removing pollutants and hazardous substances from the environment (El Berkaoui et al., Citation2022). For the purpose of this study, Typha latifolia (commonly called cattail or bulrush), Ruellia simplex (also known as Mexican petunia or Mexican bluebell) and Alternanthera philoxeroides (popularly referred to as alligator weeds or joy weeds) were selected because they were found to be widely distributed geographically in the waste management facility where the study was conducted and have certain unique morphological characteristics.
Typha latifolia is the second most widely used plant in constructed wetlands in Africa for treating domestic wastewater, storm run-off and other kinds of wastewater including industrial wastewater (Wild et al., Citation2001). It is an emergent, perennial herbaceous plant capable of growing prolifically from thick underground rhizomes to form a dense rhizome mat, with detached portions of rhizomes floating and establishing new colonies (Wagner et al., Citation1999). The plant is able to grow in a variety of climate including the tropical, subtropical and temperate regions. Ruellia simplex is also another plant able to tolerate a wide variety of soil types including heavy clay, acidic, sandy, loamy and occasionally wet soils (Ezcurra & Daniel, Citation2007; Langeland et al., Citation2008; Wagner et al., Citation1999). Literature search indicate that Alternanthera philoxeroides has its roots in a solid substrate and spread in a tangled mat over the water surface. The plants have hollow stems and can grow to about 1 m tall (Babatunde et al., Citation2008). The leaves are thick, non-succulent, and oppositely elliptically arranged (Srivastava et al., Citation2008). The plant has excellent filtration apparatus (Abbasi et al., Citation2019).
Yet, hitherto no study has been done in the context of the burgeoning oil and gas sector in Ghana, to ascertain the potential of constructed wetlands planted with indigenous plants to augment conventional treatment of oilfields wastewater. Although the role of plants in wetland ecosystems is largely known, not many native plant species have been used in constructed wetlands, especially in sub-Saharan Africa, particularly Ghana (Mekonnen et al., Citation2014). For instance, Typha latifolia have been extensively used to treat different wastewater in constructed wetlands (Babatunde et al., Citation2008; Wu et al., Citation2015); however, the contaminant removal efficiency of the plant with respect to polishing oilfield wastewater has not been assessed. Not only that, although Ruellia simplex is able to tolerate moderately to high saline environment and establish well under wet conditions, only few of such fibrous species have been used in wetland wastewater treatment systems to date (Freyre & Tripp, Citation2014). Similarly, studies have reported the use of joy weeds in treating greywater with high chemical oxygen demand similar to that of oilfields wastewater, the use of this plant in constructed wetlands is sparse (Abbasi & Abbasi, Citation2010; Abbasi et al., Citation2019: Sample et al., Citation2013). In the light of the above literature, there is therefore the need to assess the potential of native plant species widely distributed geographically in sub-Saharan Africa in constructed wetlands to solve the numerous wastewater challenge in the continent and the sub-region. This is a fundamental motivation for the present study.
This study sought to assess the contaminant removal efficiencies of three indigenous wetland plants in a small field scale free water surface flow constructed wetland as an add on to polish oilfields effluent treated in a conventional oily wastewater treatment plant. The study specifically assessed the comparative removal efficiencies of Typha latifolia, Ruellia simplex and Alternanthera philoxeroides to remove selected contaminants in a free water surface flow constructed wetland.
Materials and methods
Study area
Shama district has a land area of approximately 215 km2 and shares boundary with the Sekondi Takoradi Metropolitan Assembly (STMA) to the west and the Komenda Edina Eguafo Abirem (KEEA) district to the east and the north by the Mpohor Wassa East district. The area lies within the lowlands of the country with the elevation in most areas less than 80 metres. According to Oduro (Citation2015), the landscape is generally undulating with an average height of about 70 m. The highest elevation ranges between 150 and 200 metres above sea level. There are also few areas, mainly the valleys of the main river that do not exceed 150 metres above sea level. The district is drained by the Pra River which flows southwards and meets the sea at Shama. The Pra River delta comprises vast areas of marshlands while its estuary is associated with mangrove forests, dunes with tropical foliage, and various species of birds which perhaps are migratory and 60 diverse fauna (Oduro, Citation2015). Some coastal wetlands and mangrove forests in the district have been degraded through filing and harvesting for fuel wood. However, as compared to STMA, wetland areas and overall natural ecosystems in the Shama district is less stressed. The District lies within the tropical climatic zone and experiences two (2) rainy seasons. The major season: March-July and its minor season are from September—November. It experiences a mean rainfall of about 138 cm with a lower rainfall of 100 cm and highest rainfall 170 cm. About 70% of the rainfall occurs in the wet season. Relatively mild temperatures are experienced in the District ranging between 22 °C and 28 °C (Oduro, Citation2015). Temperature conditions readily support crop cultivation including cassava, oil palm, maize, coconut and vegetables among others. Soil Parent materials at the coastal areas have faulty shelves and sand of various types resting on hard basement of granite, gneiss and schists (Oduro, Citation2015). Non coastal areas have lower Birimian, Dixcove granite and Cape Coast granite soils. Sandy to sandy loams is predominant.
Construction of the free water surface flow constructed wetland
Free water surface flow constructed wetland system was used in this study because of its close resemblance to natural wetland and its reliability to remove biological oxygen demand and chemical oxygen demand, given sufficient hydraulic retention time (Norton, Citation2014; Verhoeven & Meuleman, Citation1999). The project was sited within the premises of an oilfields wastewater management facility. The site was cleared of preexisting vegetation and the dimensions marked on the ground to create shallow basins. Given the size of land, the aspect ratio of the shallow wetland basins was designed to be 1:5. Trenches were dug to a depth of about 0.60 m to enable the root to extend with a slight incline (~1°) from inlet to outlet to allow gravity movement of the water (Weber & Legge, Citation2008). The trench was then lined with an impermeable polymer or water proof membrane to prevent seepage. To prevent surface flow from entering the system, the borders of the basins were raised about 0.48 m above ground level. Plumbing work was done to lay major pipe lines from entry to the exit of wastewater from the system.
Planting of the indigenous plant species
The lined cells were filled with the excavated soils to about 0.30 m. This was to serve as a soil layer to support the roots of the wetland vegetation and also acts as media/sediment in the wetland. The soil was harrowed and planted with healthy, very tender, early growing Typha latifolia, Ruellia simplex and Alternanthera philoxeroides found in waterways near the wastewater management facility.
The set up in Figure shows the arrangement of the media and plants across the four wetland cells. Being a pilot study, the set up was roofed with transparent sheets to prevent precipitation at the onset of wastewater treatment. The water tanks bearing the wastewater are connected to the tap (inlet) from which the amount of water discharged is regulated. The outlet from which the effluent is discharged is positioned posterior to the cell. Eighty individuals were planted diagonally per wetland cell. The wetland basins/cells were immediately flooded with fresh water to aid growing of the newly transplanted plants. Wetland cell 1 was planted with Typha latifolia, cell 2 with Ruellia simplex, cell 3 with Alternanthera philoxeroides and cell 4 ‘without plant’ (control) as shown in Figure . The treatment wetland was maintained with daily watering during morning and evening to maintain the water level in the wetland and occasional pruning for a period of about 16 weeks to ensure proper acclimatiation. Some of the pruned leaves provided litter which served as submerged surfaces that provided physical substrate for the periphytic-attached growth of organisms responsible for most of the biological treatment. Twenty plants were systematically randomly selected and their growth rate by height measured after about two weeks of transplanting. The measurements were taken three times a week and the the mean weekly growth rate for the three plants recorded till the onset of the wastewater treatment.
Figure 1. Schematic diagram of free surface flow constructed wetland cells with 3 different native plants.
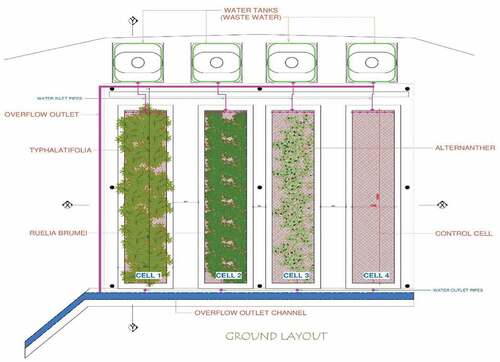
The biomass of the plant types were determined by cutting plants at ground level, put in a plastic bag and weighed immediately. The plants were air dry at room temperature for about two months until constant weight was obtained.
Experimental treatment
Hydraulic load of 2000 L (2 m3) was introduced into each of the four wetland cells for a retention time of three days (seventy-two hours). Effluents were sampled from the outlet for analysis. The study was repeated weekly for a period of five months. Parameters which are of priority to the waste management facility were selected for examination. These included total dissolved solids (TDS), conductivity, biological oxygen demand (BOD), chemical oxygen demand (COD), oil and grease (O&G), total coliform, total phosphorus and nitrate (NO3−). EC was determined using a calibrated standard cell electrode connected to a standard meter at a constant temperature based on APHA 2510, whiles TDS was measured gravimetrically based on APHA 2540C. BOD in this study was measured using respirometric method based on method APHA 10099, COD was determined using reactor digestion method based on APHA 8000 while oil and grease was measured using hexane extractable gravimetric method based on APHA 5520B. Determination of TP was by acid persulfate digestion method based on APHA 8190, nitrate by cadmium reduction method based on APHA 8039 and total coliform by plate count method based on (ISO-4833-2, 2013).
Removal efficiency was computed using the formula:
Removal efficiency (RE)% = [(influent concentration- effluent concentration)/ influent concentration] *100
Biomass of Standing plant = Dry Weight of standing plant (final weight)/Plot Area
Plot Area = 1 m*5 m = 5 m2, 80 plants per area
Water Content of plant = Fresh Weight of plant—Dry Weight of plant
Data analysis
The data were entered in Microsoft Excel, processed and exported to STATA 13 MP (StataCorp, College Station, TX, USA) for analysis. Summary statistics of the contaminants removal efficiencies of the experimental plants were performed. One-way ANOVA was used to compare the differences in mean removal efficiencies of the three plant species.
Results
There were significant heterogeneities in the concentrations of contaminants in the oilfield wastewater. The conventional treatment of oilfield wastewater was successful in reducing the level of nitrates below the discharge limit unlike the concentrations of oil and grease, BOD, COD, TDS and total phosphorus, as observed in . It was this apparent lack of success in removal or reduction in concentrations that made it imperative to augment the conventional treatment system with constructed wetlands.
Table 1. Characteristics of effluent after conventional treatment and discharge limit
Total coliform ranged between 111,000.00 and 24,196,000.00 MPN/100 ml with a regulatory discharge limit of 400 MPN/100 ml. All four wetland cells had soil/media as substrate, and this was characterised by proximate analysis. Measures of central tendency (mean) and measures of the dispersion (minimum, maximum, standard deviation) of the soil are shown in .
Table 2. Characteristics of media (soil) in the free surface flow constructed wetlands
It is evident from that 68% of the values of all the parameters in the dataset lie ±1 SD away from the mean. The only exception is the values for electrical conductivity, 95% of values are less than two standard deviations (±2SD) away from the mean. indicates that the values of EC were more dispersed. The frequency distribution for this dispersed dataset would still show a normal distribution but when plotted on a graph the shape of the curve will be flatter.
Descriptives for plant height, biomass and water content of the three plant species are shown in . The measurements for plant height indicate that the values were more dispersed around the mean height.
Table 3. Descriptive statistics for plant growth (height), biomass and water content of the three plant species
There were statistically significant differences in removal efficiencies between the treatments and control for all parameters except total phosphorus, as observed in . The order of magnitude of removal efficiency for oil and grease, BOD, COD, electrical conductivity and TDS was Alternanthera philoxeroides > Typha latifolia > Ruellia simplex. The order of magnitude of removal efficiency for total phosphorus was Typha latifolia > Alternanthera philoxeroides > Ruellia simplex. The order of magnitude of removal efficiency for nitrate was Typha latifolia > Ruellia simplex > Alternanthera philoxeroides. The order of magnitude of removal efficiency for total coliform was Ruellia simplex > Alternanthera philoxeroides > Typha latifolia. Comparison of mean contaminant concentrations () using ANOVA gave indication of the overall difference between the treatments and the control experiment.
Table 4. Contaminants removal efficiency of the native plants in a free water surface flow constructed wetland
Discussion
Generally, the results show heterogeneity in removal efficiency across plant types with unplanted wetlands recording the least removal efficiency in all parameters; an indication that macrophyte plays an important role in wastewater treatment in treatment wetlands (Brix, Citation2003). This aligns with observation by LaRiviere et al. (Citation2002) that planted wetlands facilitate removal of dissolved solids from wastewater by uptake mechanism. The removal efficiency of the planted wetlands were also observed to vary with the plant type which could be attributable to the unique morphological make-up of the plants. For instance, wetlands planted with Althernanthera philoxeroides recorded the highest removal efficiency for oil and grease, BOD, COD and salt content (see, ; Note: salt content is measured in terms of electrical conductivity and TDS) . By implication, this might mean that Althernanthera philoxeroides has certain morphological features such as extensive roots and stem that support excellent filtration and bacterial growth (Abbasi & Abbasi, Citation2010) indicates that a significant proportion of wastewater BOD, pathogen and nutrients are removed through physical process of filtration and sedimentation. Soluble organics are also degraded aerobically and anaerobically by bacterial attached to plant surfaces and sediments. Lagos et al. (Citation2015) indicate that the ability of the rhizosphere of the plant to harbour diverse forms of bacterial community promotes contaminant removal. Macrophyte with high bacterial population have increased rate at which BOD is used for cell synthesis and energy production in the wetland (Kataki et al., Citation2021). Non-settling and colloidal particles are also removed partially by bacterial growth that results in microbial decay of other organic pollutants (Saeed & Sun, Citation2012). Plant with morphological features that favour these processes are likely to record high removal efficiencies for organic pollutants.
The relatively higher reduction in electrical conductivity and TDS in Alternanthera philoxeroides planted wetland () could be due to the natural ability of the plant survive to in highly saline environment; possibly because it has adaptive features such as excretory glands and bladder hairs that remove salts from salt-sensitive metabolic sites (Koyro et al., Citation2008). The observed reduction in salt content could partly be due to the presence of algal traces observed in the Alternanthera philoxeroides planted wetland cell. El Sergany et al. (Citation2014) achieved between 13% and 63% removal of TDS in an experiment to desalinate brine with algae. This observation is also supported by Sooknah and Wilkie (Citation2004) who found that algal activities brought about 52% reduction in conductivity in algal controls and pennyworth grown setup with traces of algae. Alternanthera philoxeroides, unlike Typha and Ruellia had its stems and leaves in the water, hence unable to attenuate sunlight to curtail the growth of algae. Although this is usually a challenge, other authors have found it useful in the treatment of electrical conductivity and TDS.
The removal efficiency for total coliform was comparable in all the planted wetland cells, but slightly lower in the unplanted wetland cell. This observation is in line with findings by García et al. (Citation2008), where planted wetlands recorded higher removal efficiencies than unplanted wetland (control). The study recorded removal efficiencies for total coliform between 44% and 50%. This is slightly lower than observations made by authors such as Weber and Legge (Citation2008), and Kadlec (Citation2009) where coliform removal ranged between 65% and 99%. observed that the the removal efficiency of pathogenic organisms in free water surface flow constructed wetlands were a little lower compared with subsurface flow constructed wetlands. Coliform removal was found to be slightly higher in wetland planted with Ruellia simplex. Vymazal (Citation2011) found that some wetland plant species produce root exudates which are antibiotics and are able to contribute to the removal of bacterial communities in constructed wetland. Others produce tannic and gallic acids (anti-bacterial substances), which according to Kinnear et al. (Citation2008), are responsible for antimicrobial activity, though other compounds may probably be involved as well. Weber and Legge (Citation2008) state that plant type is among the latent variables that influence the removal efficiencies. This could be seen in where different plants recorded different removal efficiencies for total coliform. The observed higher total coliform removal recorded by wetland planted with Ruellia simplex could be due to the unique morphology of the plant such as fibrous root system and physiology of the plants (Abbasi & Abbasi, Citation2010; Kadlec, Citation2000; Verhoeven & Meuleman,Citation1999; Vymazal, Citation2005).
Although it is generally believed that factors such as sunlight, pH level and dissolved oxygen concentration cause bacterial die-off, García et al. (Citation2008) explains that macrophyte-dependent mechanisms are comparatively more dominant in causing bacterial die-off. They hold the view that the presence of macrophytes indirectly contribute to bacterial die off through such mechanisms as conductivity modification, adsorption, aggregation, filtration, gas transport and enhancement of biofilm development.
The present study showed significant differences in mean removal efficiency of Alternanthera philoxeroides, Typha latifolia and Ruellia simplex with respect to nutrients (nitrogen and phosphorus). Wetland planted by Typha latifolia recorded the highest removal for nutrients. Abbasi and Abbasi (Citation2010) indicate that the rate of pollutant removal and storage is a function of the growth rate and biomass of standing plant per unit area. The mean growth rate of Typha latifolia before wastewater treatment was found to be about two times higher than Ruellia simplex and about three times higher than that of Alternanthera philoxeroides (). The high growth rate of Typha latifolia might have enhanced its nutrient removal efficiency in the wastewater. Andries et al. (Citation2018) point out that morphological factors of the vegetation condition the area or surface absorbed from the root system is one of three factors that affect the extraction of nutrients by plants.
Phosphorus and nitrogen removal are related to standing plant biomass so plants with larger biomass per unit area have greater potential to store nutrients than plants with a lower standing crop of biomass per unit area. Biomass was found to be highest in Alternanthera philoxeroides followed by Ruellia simplex and Typha latifolia (). However, high nutrient removal may be shortlived due to poor nutrient locking ability as a result of lack of frequent harvesting which could cause dead and decompose tissues to release the nutrients back into the water (Kadlec & Wallace, Citation2008; Vymazal, Citation2007). In this study, the observation was counter intuitive. Typha latifolia with the least standing plant biomass recorded the highest nitrate and total phosphorus removal, probably due to its extensive supportive tissue and greater potential for storing nutrients compared with Alternanthera philoxeroides and Ruellia simplex (see, Abbasi & Abbasi, Citation2010). Mechanism for nitrogen removal includes plant uptake, ammonia volatiliation and bacterial nitrification and denitrification. Out of these, plant uptake has the highest nitrogen removal potential in constructed wetlands. Nitrification of ammonium is the mechanism for nitrogen removal in vegetated constructed wetland. This process occurs in the oxidised root zone of macrophytes which is enhanced beneath stands of plants which transport large quantities of oxygen (Abbasi & Abbasi, Citation2010). Nutrient removal in vegetated constructed wetland may not only depend on the biomass of standing plant, but other environmental conditions such as temperature, pH, amount of dissolved oxygen and the redox condition of the wetland (Vymazal et al., Citation1998; Yang et al., Citation2007). Phosphorus removal depends on adsorption and desorption, precipitation and dissolution, plant and microbial uptake, fragmentation and leaching, mineralisation, sedimentation, and burial that take place in the constructed wetland (Sedlak, Citation2018; Vohla et al., Citation2011; Wang & Li, Citation2010). Consistent with this study, some studies (Ayaz et al., Citation2012; Ballantine & Tanner, Citation2010; Wu et al., Citation2015) have shown that plant uptake and harvest can be effective in removing phosphorus from macrophyte based constructed wetland. The comparatively high removal (about 32%) in the control could be due the adsorption-precipitation reaction of with Calcium and clay minerals in the soil used (Brix, Citation1997). shows the calcium content of the soil used.
Conclusion
This study sought to assess the contaminant removal efficiencies of three indigenous plant species, Typha latifolia, Ruellia simplex and Alternanthera philoxeroides, which are widely distributed in an oilfield wastewater management facility in Ghana. The study was carried out in a small field scale free surface flow constructed wetland to serve as an add-on for polishing oilfields effluent treated from a conventional wastewater treatment plant. The results showed heterogeneity in removal efficiency across plant types and across the parameters studied, with unplanted wetlands recording the least removal efficiency on all parameters. This implies that presence of plants in constructed wetland enhances contaminant removal efficiency. Among the three plant species, Alternanthera philoxeroides and Typha latifolia (all with rhizomatous root system) recorded higher mean removal efficiencies than Ruellia simplex (with fibrous root system) for most of the parameters studied. The removal efficiency of the plants varied with the plant type which could be attributed to the uniqueness in their morphological make-up. Organics and salt contaminants removal were more effective in Alternanthera philoxeroides planted wetland, whiles Phosphorus and nitrogen removal were high in Typha latifolia planted wetland with Ruellia simplex planted wetland performing well in total coliform removal. This means that mixed planting of these species could be considered if the contaminants selected for this study are to be thoroughly removed. However, allelopathic influence of these plants species should be studied if they are to be planted in the same constructed wetland. Antibacterial characteristics of root exudate of the plant types could also be further studied to establish how it contribute toward the removal of total coliform in constructed wetlands. The removal efficiencies of Ruellia simplex in the study suggest that it may not be a good wetland plant for produced water treatment, but could be explored for other kinds of wastewater that are not extensively polluted.
Acknowledgements
This study was funded by the Regional Water and Environmental Sanitation Centre Kumasi (RWESCK) at the Kwame Nkrumah University of Science and Technology, Kumasi with funding from Ghana Government through the World Bank under the Africa Centre’s of Excellence project.’ The views expressed in this paper do not reflect those of the World Bank, Ghana Government and KNUST.
Funding
This work was supported by The World Bank Group through Africa Centre for Excellence through Ghana Government.
Disclosure statement
No potential conflict of interest was reported by the author(s).
References
- Abbasi, T., & Abbasi, S. A. (2010). Factors which facilitate waste water treatment by aquatic weeds–the mechanism of the weeds’ purifying action. International Journal of Environmental Studies, 67(3), 349–10. https://doi.org/10.1080/00207230902978380
- Abbasi, S. A., Tabassum-Abbasi, P. G., & Tauseef, S. M. (2019). Potential of joyweed Alternanthera sessilis for rapid treatment of domestic sewage in SHEFROL® bioreactor. International Journal of Phytoremediation, 21(2), 160–169. https://doi.org/10.1080/15226514.2018.1488814
- Alufasi, R., Gere, J., Chakauya, E., Lebea, P., Parawira, W., & Chingwaru, W. (2017). Mechanisms of pathogen removal by macrophytes in constructed wetlands. Environmental Technology Reviews, 6(1), 135–144. https://doi.org/10.1080/21622515.2017.1325940
- Andries, R. G., Matos, A. T. D., & Freitas, W. D. S. (2018). Estimation of plant productivity and nutrient extraction capacity along the length of horizontal subsurface flow constructed wetland treating swine wastewater. Revista Ambiente & Água, 13(3). http://www.scielo.br/scielo.php?pid=S1980-993X2018000300301&script=sci_arttext
- Arthur, J. D., Langhus, B. G., & Patel, C. (2005). Technical summary of oil & gas produced water treatment technologies. All Consulting.
- Ayaz, S. Ç., Aktaş, Ö., Findik, N., & Akça, L. (2012). Phosphorus removal and effect of adsorbent type in a constructed wetland system. Desalination and Water Treatment, 37(1–3), 152–159. https://doi.org/10.1080/19443994.2012.661267
- Babatunde, A. O., Zhao, Y. Q., O’neill, M., & O’sullivan, B. (2008). Constructed wetlands for environmental pollution control: A review of developments, research and practice in Ireland. Environment International, 34(1), 116–126. https://doi.org/10.1016/j.envint.2007.06.013
- Ballantine, D. J., & Tanner, C. C. (2010). Substrate and filter materials to enhance phosphorus removal in constructed wetlands treating diffuse farm runoff: A review. New Zealand Journal of Agricultural Research, 53(1), 71–95. https://doi.org/10.1080/00288231003685843
- Brix, H. (1997). Do macrophytes play a role in constructed treatment wetlands? Water Science and Technology, 35(5), 11–17.
- Brix, H. (2003). Plants used in constructed wetlands and their functions. 1st International Seminar on the Use of Aquatic Macrophytes for Wastewater Treatment in Constructed Wetlands, Edit. Dias V., Vymazal J. Lisboa, Portugal, Hydrobiologia (2011) 674, 133–156. https://doi.org/10.1007/s10750-011-0738-9
- Calheiros, C. S., Rangel, A. O., & Castro, P. M. (2007). Constructed wetland systems vegetated with different plants applied to the treatment of tannery wastewater. Water Research, 41(8), 1790–1798. https://doi.org/10.1016/j.watres.2007.01.012
- Cooper, P. F., & Findlater, B. C. (1999). In A review of the design and performance of vertical-flow and hybrid reed bed treatment systems. Water Science and Technology, 40(3), 1-9.
- Cooper, P. F. (2005). The performance of vertical flow constructed wetland systems with special reference to the significance of oxygen transfer and hydraulic loading rates. Water Science and Technology, 51(9), 81–90. https://doi.org/10.2166/wst.2005.0293
- Corcoran, E. (Ed.). (2010). Sick water?: The central role of wastewater management in sustainable development: A rapid response assessment. UNEP/Earthprint. http://purl.unep.org/sdg/SDGIO_00000048
- Crites, R. W., Middlebrooks, E. J., & Reed, S. C. (2005). Natural wastewater treatment systems. CRC Press.
- El Berkaoui, M., El Adnani, M., Hakkou, R., Ouhammou, A., Bendaou, N., & Smouni, A. (2022). Assessment of the transfer of trace metals to spontaneous plants on abandoned pyrrhotite mine. Potential Application for Phytostabilization of Phosphate Wastes. Plants, 11(2), 179. https://doi.org/10.3390/min12030334
- El Sergany, R., El Fadly, M., & El Nadi, A. (2014). Brine desalination by using algae ponds under nature conditions. American Journal of Environmental Engineering, 4(4), 75–79. https://doi.org/10.5923/j.ajee.20140404.02
- Ezcurra, C., & Daniel, T. F. (2007). Ruellia simplex, an older and overlooked name for ruellia tweediana and ruellia coerulea (Acanthaceae). Darwiniana, 45, 201–203. ID: 83647548.
- Fan, X., Du, Y., Luo, B., Han, W., Niu, S., Gu, W., & Ge, Y. (2021). Increasing plant diversity to mitigate net greenhouse effect of wastewater treatment in floating constructed wetlands. Journal of Cleaner Production, 314,127955. https://doi.org/10.1016/j.jclepro.2021.127955
- Freyre, R., & Tripp, E. (2014). Artificial hybridization between US native Ruellia caroliniensis and invasive Ruellia simplex: Crossability, morphological diagnosis, and molecular characterization. Hort Science, 49(8), 991–996. https://doi.org/10.21273/HORTSCI.49.8.991
- Gabr, M. E. (2022). Design methodology for sewage water treatment system comprised of Imhoff‘s tank and a subsurface horizontal flow constructed wetland: A case study Dakhla Oasis, Egypt. Journal of Environmental Science and Health, Part A, 57(1), 52-64. https://doi.org/10.1080/10934529.2022.2026735
- García, M., Soto, F., González, J. M., & Bécares, E. (2008). A comparison of bacterial removal efficiencies in constructed wetlands and algae-based systems. Ecological Engineering, 32(3), 238–243. https://doi.org/10.1016/j.ecoleng.2007.11.012
- Igunnu, E. T., & Chen, G. Z. (2014). Produced water treatment technologies. International Journal of Low-Carbon Technologies, 9(3), 157–177. https://doi.org/10.1093/ijlct/cts049
- Kadlec, R. H. (2000). The inadequacy of first-order treatment wetland models. Ecological Engineering, 15(1–2), 105–119.
- Kadlec, R. H., & Wallace, S. (2008). Treatment Wetlands (2nd ed). CRC Press.
- Kadlec, R. H. (2009). Comparison of free water and horizontal subsurface treatment wetlands. Ecological Engineering, 35(2), 159–174. https://doi.org/10.1016/j.ecoleng.2008.04.008
- Kataki, S., Chatterjee, S., Vairale, M. G., Sharma, S., Dwivedi, S. K., & Gupta, D. K. (2021). Constructed wetland, an eco-technology for wastewater treatment: A review on various aspects of microbial fuel cell integration, low temperature strategies and life cycle impact of the technology. Renewable and Sustainable Energy Reviews, 148, 111261. https://doi.org/10.1016/j.rser.2021.111261
- Kinnear, S. H. W., Fabbro, L. D., & Duivenvoorden, L. J. (2008). Variable growth responses of water thyme (Hydrilla verticillata) to whole-cell extracts of Cylindrospermopsis raciborskii. Archives of Environmental Contamination and Toxicology, 54(2), 187–194. https://doi.org/10.1007/s00244-007-9026-0
- Koyro, H. W., Geissler, N., Hussin, S., Debez, A., & Huchzermeyer, B. (2008). Strategies of halophytes to survive in a salty environment. Abiotic stress and plant responses. IK International Publishing House.
- Lagos, L., Maruyama, F., Nannipieri, P., Mora, M. L., Ogram, A., & Jorquera, M. A. (2015). Current overview on the study of bacteria in the rhizosphere by modern molecular techniques: A mini‒review. Journal of Soil Science and Plant Nutrition, 15(2), 504–523. https://doi.org/10.4236/nr.2015.64026
- Langeland, K. A., Cherry, H. M., McCormick, C. M., & Burks, K. C. (2008). Identification and biology of nonnative plants in Florida’s natural areas. Univ. Florida, Inst. Food Agr. Sci. Commun. Serv. Gainesville, FL.
- LaRiviere, D., Autenreith, R. L., & Bonner, J. (2002). Oxidation of rhizosphere sediments by Alternanthera philoxeroides: Roots to quicker petroleum degradation? In Arctic And Marine Oilspill Program Technical Seminar, Vol. 1 (pp. 341–354).
- Licata, M., Gennaro, M. C., Tuttolomondo, T., Leto, C., & La Bella, S. (2019). Research focusing on plant performance in constructed wetlands and agronomic application of treated wastewater–A set of experimental studies in Sicily (Italy). PloS one, 14(7), e0219445. https://doi.org/10.1371/journal.pone.0219445
- Mekonnen, A., Leta, S., & Njau, K. N. (2014). Wastewater treatment performance efficiency of constructed wetlands in African countries: A review. Water Science and Technology, 71(1), 1–8. https://doi.org/10.2166/wst.2014.483
- Norton, S. (2014, Removal mechanisms in constructed wastewater wetlands. eng. iastate. edu/~, http://home.eng.iastate.edu/~tge/ce421-521/stephen. pdf
- Oduro, E. (2015). Perceived effect of the integrated coastal fisheries governance programme on food security status of fishing households in shama district in the Western Region of Ghana (Doctoral dissertation, University of Cape Coast).
- Rodriguez-Dominguez, M. A., Bonefeld, B. E., Ambye-Jensen, M., Brix, H., & Arias, C. A. (2022). The use of treatment wetlands plants for protein and cellulose valorization in biorefinery platform. Science of the Total Environment, 810, 152376. https://doi.org/10.1016/j.scitotenv.2021.152376
- Saeed, T., & Sun, G. (2012). A review on nitrogen and organics removal mechanisms in subsurface flow constructed wetlands: Dependency on environmental parameters, operating conditions and supporting media. Journal of Environmental Management, 112, 429–448. https://doi.org/10.1016/j.jenvman.2012.08.011
- Sample, D., Wang, C. Y., & Fox, L. (2013). Innovative Best Management Fact Sheet. 1. Floating Treatment Wetlands.
- Saravanan, S., Nethala, S., Pattnaik, S., Tripathi, A., Moorthi, A., & Selvamurugan, N. (2011). Preparation, characterization and antimicrobial activity of a bio-composite scaffold containing chitosan/nano-hydroxyapatite/nano-silver for bone tissue engineering. International Journal of Biological Macromolecules, 49(2), 188–193. https://doi.org/10.1016/j.ijbiomac.2011.04.010
- Scholz, M., & Lee, B. H. (2005). Constructed wetlands: A review. International Journal of Environmental Studies, 62(4), 421–447. https://doi.org/10.1080/00207230500119783
- Sedlak, R. (2018). Phosphorus and nitrogen removal from municipal wastewater: Principles and practice. Routledge.
- Sekyi, R. (2011). AKOBEN: Ghana’s new initiative for environmental performance rating and disclosure in the mining sector. In Proceedings Tailings and Mine Waste (pp. 1–20).
- Sooknah, R. D., & Wilkie, A. C. (2004). Nutrient removal by floating aquatic macrophytes cultured in anaerobically digested flushed dairy manure wastewater. Ecological Engineering, 22(1), 27–42. https://doi.org/10.1016/j.ecoleng.2004.01.004
- Srivastava, J., Gupta, A., & Chandra, H. (2008). Managing water quality with aquatic macrophytes. Reviews in Environmental Science and Bio/Technology, 7(3), 255–266. https://doi.org/10.1007/s11157-008-9135-x
- Verhoeven, J. T., & Meuleman, A. F. (1999). Wetlands for wastewater treatment: Opportunities and limitations. Ecological Engineering, 12(1–2), 5–12. https://doi.org/10.1016/S0925-8574(98)00050-0
- Vohla, C., Kõiv, M., Bavor, H. J., Chazarenc, F., & Mander, Ü. (2011). Filter materials for phosphorus removal from wastewater in treatment wetlands—A review. Ecological Engineering, 37(1), 70–89. https://doi.org/10.1016/j.ecoleng.2009.08.003
- Vymazal, J., Brix, H., Cooper, P. F., Green, M. B., & Haberl, R. (Eds.). (1998). Constructed wetlands for wastewater treatment in Europe. Backhuys Publishers.
- Vymazal, J. (2005). Constructed wetlands for wastewater treatment. Ecological Engineering, 25(5), 475–477. https://doi.org/10.1016/j.ecoleng.2005.07.002
- Vymazal, J. (2007). Removal of nutrients in various types of constructed wetlands. Science of the Total Environment, 380(1–3), 48–65. https://doi.org/10.1016/j.scitotenv.2006.09.014
- Vymazal, J. (2011). Constructed wetlands for wastewater treatment: Five decades of experience. Environmental Science and Technology, 45(1), 65–69. https://doi.org/10.1021/es101403q
- Vymazal, J., Zhao, Y., & Mander, Ü. (2021). Recent research challenges in constructed wetlands for wastewater treatment: A review. Ecological Engineering, 169(13), 106318. https://doi.org/10.1016/j.ecoleng.2021.106318
- Wagner, W. L., Herbst, D. R., & Sohmer, S. H. (1999). Manual of the flowering plants of Hawaiʻi. University of HawaiʻiPress.
- Wang, Q., & Li, Y. (2010). Phosphorus adsorption and desorption behavior on sediments of different origins. Journal of Soils and Sediments, 10(6), 1159–1173. https://doi.org/10.1007/s11368-010-0211-9
- Weber, K. P., & Legge, R. L. (2008). Pathogen removal in constructed wetlands. In Wetlands: ecology, conservation and restoration, chapter 5 (pp. 176–211). Nova Science Publishers, Inc.
- Wild, U., Kamp, T., Lenz, A., Heinz, S., & Pfadenhauer, J. (2001). Cultivation of Typha spp. in constructed wetlands for peatland restoration. Ecological Engineering, 17(1), 49–54. https://doi.org/10.1016/S0925-8574(00)00133-6
- Wu, H., Zhang, J., Ngo, H. H., Guo, W., Hu, Z., Liang, S., & Liu, H. (2015). A review on the sustainability of constructed wetlands for wastewater treatment: Design and operation. Bioresource Technology, 175, 594–601. https://doi.org/10.1016/j.biortech.2014.10.068
- Yadav, M., Gupta, R., & Sharma, R. K. (2019). Green and sustainable pathways for wastewater purification. In Advances in water purification techniques (pp. 355–383). Elsevier.
- Yang, Q., Chen, Z. H., Zhao, J. G., & Gu, B. H. (2007). Contaminant removal of domestic wastewater by constructed wetlands: Effects of plant species. Journal of Integrative Plant Biology, 49(4), 437–446. https://doi.org/10.1111/j.1744-7909.2007.00389.x
- Yang, J., Qi, Y., Li, H., & Xu, G. (2018). Comparison of nitrogen and phosphorus purification effects of different wetland plants on eutrophic water. In IOP Conference Series: Earth and Environmental Science, 113( 1), 012042. IOP Publishing.