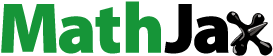
ABSTRACT
Organochlorine pesticides have been banned in Mexico since 1991, but they are still present in ecosystems, representing a health risk. The Navachiste Lagoon Complex is a RAMSAR site and a fishing area for various edible species. The objective of this study was to determine the cancer health risks posed by the consumption of crab Callinectes bellicosus from the Navachiste Complex, collected from April 2014 to January 2015; and to compare analyte ratios of selected against their metabolites to determine if recent sources of pesticides being bioaccumulated of this species. The highest average organochlorine concentrations were detected for γ -HCH, α-HCH, and δ-HCH with 1.9 × 10−5, 1.7 × 10−5, and 1.3 × 10−5 mg kg−1, respectively. Significant seasonal variations were found in aldrin and heptachlor (autumn), α-HCH and dieldrin (spring), and heptachlor epoxide (spring and summer) (p < 0.05). The highest organochlorine average exposure risk for consumption in the 95 percentile range was for endosulfan II (0.007 mg kg−1 d−1). According to our findings, the consumption at 0.204 g d−1 of edible tissue of crab from the Navachiste Lagoon Complex does not pose cancer health risk.
Introduction
Among the different kinds of pesticides, the organochlorine pesticides (OCP) are considered as persistent organic pollutants (POPs), and are prohibited in most of the G20 countries, to which Mexico belongs (G20, The G20 countries, Citation2017). The residues of OCP end up incorporated in the environment through agricultural drains (Barakat et al., Citation2017; Kachi et al., Citation2016; Lee et al., Citation2015; Lupi et al., Citation2016; Toan, Citation2015). One of the largest agriculture areas of Mexico is the Guasave Valley (>110 000 ha) with two agricultural cycles (autumn-winter and spring-summer) under intensive agriculture (CitationSIAP), that surrounds the ~24,000 ha of the Navachiste Lagoon Complex (NAV). Since agriculture intensity contributes to the ecological pollution with OCP residues which increase the OCP bioavailability in the trophic web of marine ecosystems (Lupi et al., Citation2016), during these two culture cycles the NAV is subject to high OCP pollution pressure. In intensive agriculture, pesticides are applied during the four seasons of the year (Silveira-Gramont et al., Citation2018), with a strong likelihood of generating OCP loads to the atmosphere and runoff (Li et al., Citation2011). Indeed OCP residues have been reported in biota and sediments of the Gulf of California and nearby coastal zones of the study area (Arellano-Aguilar et al., Citation2017; Garcia Hernandez et al., Citation2015; Granados-Galván et al., Citation2015; Reyes-Montiel et al., Citation2013). NAV possesses a very important biodiversity richness, with artisanal fisheries being important to the region (Aguilar-Gonzalez et al., Citation2014; Carrasquilla-Henao et al., Citation2013), and the presence of OCP residues in the seafood can represent a potential cancer risk for humans (Polder et al., Citation2014). Due to the feeding habits of omnivorous and detritivore species, such as the crab Callinectes spp., this organism can bioaccumulate and biomagnify the OCPs, present in the ecosystem, in their edible tissues (Magalhães et al., Citation2012; Tekin & Pazi, Citation2017). Probably, the Callinectes bellicosus crab of the NAV complex bioaccumulates OCP residues in the edible tissues, and its consumption could represent a health risk for humans. In this sense, due to the commercial importance and constant consumption of C. bellicosus from the NAV complex in the international markets, the main objective of this study was to determine the cancer health risks posed by the consumption of crab Callinectes bellicosus from the Navachiste Complex, collected from April 2014 to January 2015; and compare analyte ratios of selected against their metabolites to determine if recent sources of pesticides being bioaccumulated of this species.
Material and methods
Ninety-four samples of the crab species, C. bellicosus, were seasonally collected from April 2014 to January 2015 (24 samples in 27 April 2014 in 26 July 2014 in November 2014 and 17 in January 2015; Figure ) in the NAV, Sinaloa, Mexico.
Samples were collected with traps and wrapped in aluminum foils, and then individually kept in a 40-L cooler with ice and transported to the Pollution Laboratory at the CIIDIR-SINALOA. The water parameters of the NAV (pH, temperature, salinity, and dissolved oxygen) were recorded with a multiparameter HANNA® HI-9828 instrument (HANNA Instruments, USA). All edible crab tissues were extracted and analyzed in the laboratory, following the EPA 8081b method (USEPA, Citation2007). Extraction of OCPs, detection and quantification of analytes were performed with pesticides-grade reagents and chemical solvents. OCPs were extracted through maceration of tissues with hexane and anhydrous sodium sulfate, followed by sonication for 15 minutes (Bransonic® ultrasonic cleaner). The extracted hexane was purified with a clean-up column (fiberglass wool, alumina, Florisil®, silica gel, and anhydrous sodium sulfate, at a proportion of 2:1:1:1:3, respectively). The extracts were concentrated and completely dried under a hood, and then resuspended in 2 mL of isooctane. Quantification of OCPs was performed in a gas chromatograph XL (PerkinElmer®) with 63Ni-EDC (electron capture detector). The analytical chromatographic column was an Elite-CLP2 capillary (PerkinElmer®). The programmed conditions were: oven 120°C (hold 1 min) to 240°C at 4°C/min; EDC at 300°C; injector 260°C; 2 µL of samples injected; split-splitless on; attenuation 16; nitrogen gas carrier at 8.7 psi; nitrogen make-up at 30 mL/min. Limits of detection, average recovery, and average recovery of fortification samples were used to ensure quality controls and a standard pesticide 8081, standard mix, and pesticides surrogate spike mix (SUPELCO® part number: CRM46845 and CRM48460, respectively) were used following the statistical calculations described in the EPA method 8081b (USEPA, Citation2007; Table ).
Table 1. Limits of detection, average recovery, and average recovery of fortification samples and coefficient of variation
Results were analyzed using ANOVA test (p ˂ 0.05, α = 0.05) to determine differences among OCP concentrations per season, and a post-hoc Tukey HSD test calculator (Vasavada, Citation2014a) with Scheffé, Bonferroni, and Holm multiple comparison was applied (p > 0.05, α = 0.05) to determine significant differences. A Pearson correlation test (p ˂ 0.05) was applied (Vasavada & Spearman, Citation2014b) to compare between OCP concentrations. The average concentration of each OCP and by OCP family was calculated. The potential health risk for the OCP residues consumption was determined assuming an adult body weight (BW) of 70 kg and life expectancy in Mexico of 75.2 years (USEPA, Guidance for Assessing Chemical Contaminant Data for Use,). The estimated daily organochlorine intake risk (EDI) of the C. bellicosus consumption was calculated based on the international MLRs guidelines (USEPA, Integrated Risk Information System (IRIS), Citation2013), and through the equation EDI = ( × FCR)/BW, were
= OCP concentration in C. bellicosus edible tissue (mg kg−1), FCR =Food consume rate of C. callinectes (mg kg−1). The risk to develop cancer for the consumption of the blue crab from NAV, were calculated trough the Hazard Risk (HR = EDI/CBC), were CBC = Cancer benchmark concentration (mg kg−1). To evaluate the toxicity of cumulative effect of pesticides, a cumulative hazard index was calculated per sample using the equation
CBC = Cancer Benchmark Concentration. CBC of C. bellicosus meat were evaluated based in international regulated Oral Slope Factors (OSF; EC, Citation2005; USEPA, Integrated Risk Information System (IRIS), Citation2013), using the equation CBC = Risk level (1 ×10−6 probability to develop cancer in a lifetime) × (BW) ⁄ (FCR × OSF; Adeleye et al., Citation2019).
Results and discussion
Twenty different OCPs were detected, within seven families: Σdrines (aldrin, dieldrin, endrin, endrin aldehyde, endrin ketone), Σhexachlorocyclohexanes (α-HCH, γ-HCH, β-HCH, δ-HCH), Σchlordanes (γ-chlordane, α-chlordane), Σendosulfans (endosulfan I, endosulfan II, endosulfan sulfate), ΣDDTs (4-4’-DDT, 4-4’-DDD, 4-4’-DDE), Σheptachlors (heptachlor, heptachlor epoxide), and methoxychlor. The highest occurrence of OCPs C. bellicosus edible tissue were δ-HCH, aldrin and γ-HCH, and they were the most frequent among collecting seasons Summer, Autumn and Winter; followed in frequency by season by heptachlor epoxide, endrin, α-HCH and β-HCH (Figure ). Endosulfan sulfate (17.6 %), endrin ketone (12.2 %), endosulfan II (9.1 %), and Metoxichlor (8.6 %) had the highest percentage by seasons (Spring, Summer, Autumn, and Winter, respectively). The overall average concentrations were 57.2, 33.9, 29.7, and 92.6 ng kg−1, for Spring, Summer, Autumn, and Winter, respectively (Figure ).
Figure 2. Total (A) and average (B) detected OCP concentrations (mg kg−1) in C. bellicosus from NAV.
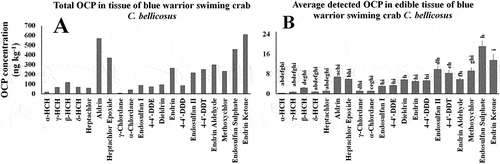
Different letters in the charts mean significant differences groups (p < 0.05).
Figure 3. Average total OCP concentration detected in the edible tissue of C. bellicosus from NAV. Different letters mean significant differences among periods (p < 0.05).
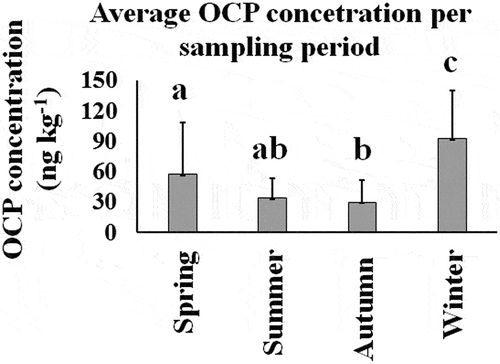
The mean of individual OCP analytes across all samples ranged from 3.2 ×10−7 to 1.9 ×10−5 mg kg−1.
ANOVA test showed significant differences among average OCP families concentrations (p < 0.01). Endosulfan sulfate presented the highest median concentration (17.6 %), followed by endrin ketone (12.2 %), endosulfan II (9.1 %), metoxychlor (8.6 %) and 4-4’-DDT (7.7 %); the rest of OCPs were detected below 5 % across samples, and no significant differences (post-hoc HSD p > 0.05) were determined among mean OCP concentrations across samples (Figure ).
Figure 4. Total OCP isomer families (A) and average OCP (B) detected concentrations in C. bellicosus from NAV. Different letters mean significant differences (p < 0.05).
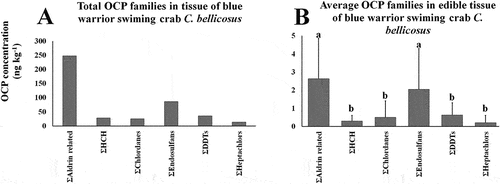
Compared to previous studies ion crabs (Chen et al., Citation2014; Khairy et al., Citation2019; Kilercioglu et al., Citation2018; Saber et al., Citation2018), the OCP concentrations in C. bellicosus from Navachsite complex were lower, and the endrin aldehyde in the present report were higher than those reports (Table ).
Table 2. Average OCP estimated exposure (mg kg−1 day−1) to the population for consumption of seafood in the world
Similar to previous studies in Navachiste complex have reported HCH´s isomers and aldrin, as the most frequent OCPs in edible tissues of other species (Granados-Galván et al., Citation2015; Montes et al., Citation2012; Reyes-Montiel et al., Citation2013). HCHs persist in the soil environment for several years, and β-HCH and δ-HCH were detected in the present study as the most frequent. The higher presence of the degraded forms of OCPs indicates an historic use (Garcia Hernandez et al., Citation2015). In the present study, some isomer concentrations were higher than the parent metabolite such as HCH, chlordane, endrin and endosulfan (Figure ).
Figure 5. Estimated average OCP exposure (50 and 95 percentile). (a) OCP carcinogenic; (b) non–carcinogenic; (c) risk for the consumption of edible tissues of C. bellicosus from NAV.
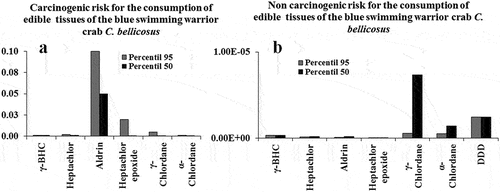
The proportions of the technical compounds are: for constitutive technical HCH (t-HCH) α- (60–70%), β- (5–12%), γ- (10–12%), δ- (6–10%), ε- (3–4%; Nayyar et al., Citation2014); for commercial DDT are 4-4’-DDT (65–80 %), 4-4’-DDE (15–21 %), and 4-4’-DDD (up to 4 %; Faroon & Harris, Citation2002); for technical chlordane are α- and, γ-chlordane, heptachlor, heptachlor epoxide (with 71.7, 23.1, 0.3, and 1.95 %, respectively), more two nanochlor isomers (USEPA, Citation1997); and for endosulfan consists of two stereoisomers, α- and β-endosulfan, in an approximate ratio of 70:30 (Ghadiri and Rose, 2001). It is known that the ratio of the parent/metabolite can serve as an indication of the degradation degree of OCP (Table ), in this sense was observed recent use of β-HCH, α-, y- and heptachlor, α-endosulfan and aldrin.
Table 3. Ranges of parent/metabolite (or degradation products) ratios indicative of recent use (>1) and legacy use (<1) of OCPs
t- indicate technical compounds (parents). Ratio ≤ 1 indicates aged uses, while ratios > 1 implicates recent use (Kafaei et al., Citation2020; Leung et al., Citation2020).
The elevated ratio of α-heptachlor maybe of recent use, because the time of soil half-life range from 37 to 3500 days of chlordane (USEPA, Guidance for Assessing Chemical Contaminant Data for Use,). In the case of the rate of α-endosulfan, could be recently used because is known of during the first 20 days of use this analyte is degraded to endosulfan sulfate (Ghadiri, Citation2001), which could imply that endosulfan was used within the last six months before sampling, and proportions could be related on the bioaccumulation and age of crabs (Kim, Citation2020). On the other hand, the ratio of aldrin could indicate its recent use because the volatilization and degradation of this analyte derive to dieldrin in 1 or 2 weeks (ATSDR, Citation2002). endrin degrades to form endrin aldehyde and endrin ketone (with the degradation of the endrin epoxide group, aldehyde or ketone is produced), the process lasts between 15 and 19 days (Taylor et al., Citation1994). Drines´ family concentrations were higher compared to previous reports in crabs and could be related to bioaccumulation, bio-transformation, genus, and age of each species (Dias et al., Citation2013). Although pollution by OCP residues in the NAV complex has been reported and attributed to the drains from the Guasave Valley (Montes et al., Citation2012), studies are necessary to determine if the OCP accumulation in the edible tissue crabs is caused by persistence or intensive use of pesticides.
Even most of the detected OCPs in the present study are used in intensive agriculture activities and are banned in Mexico, the higher OCP concentration in the Spring could be caused by the discharge of maize culture intensive irrigation that is carried from October to March (Leyva Morales et al., Citation2014), which residues are constantly discharged to the NAV (Carrasquilla-Henao et al., Citation2013). In the Spring (April, 2014) were identified in higher concentration the dieldrin (13.22 mg kg−1), heptachlor epoxide (7.85 mg kg−1), and α-HCH (1.48 mg kg−1); while in the Summer (July, 2014), the higher concentration was detected by heptachlor epoxide (7.78 mg kg−1). In the Autumn (November 2014), the heptachlor (3.96 mg kg−1) was significantly higher. Different concentrations of OCP metabolites in the edible tissue of the blue crabs could be attributed to the intensive irrigation season and the crop phase when the OCP is applied to combat the pest. In the present study, the highest concentrations of OCP’s are not linked to the seasons of the year but after the more abundant rains and after the intensive irrigation months of the crops. It is difficult to establish periods of consumption since this species follows an annual life cycle and adult phase is reached in the months after irrigation or rainy season (August to November). Regarding the significant statistical differences observed between the correlations of the physicochemical parameters of the water with the OCP by seasons of the year, they were observed between salinity and OCP in Summer and Winter; the pH with OCP in Spring; conductivity with OCP in Summer; and the temperature (in °C) with the OCP in Autumn and Winter (Table ).
Table 4. Correlation (r2) among water physicochemical parameters and mean OCP concentrations (mg kg−1 d−1) detected in edible tissues of C. bellicosus, from the NAV, Mexico
Different letters mean differences among parameters. Significance: p < 0.05 and number are in cursive bold format.
Probably the variation of the physicochemical parameters given by the annual seasons promotes the confinement of the OCP in the area, making them bioavailable for various non-target species such as crabs. According to Lakhlalki et al., (Citation2020) the pH, salinity, and dissolved O2 might influence the accumulation of OCPs in water, and temperature is associated with a negative correlation because of a decrease of salinity and conversely. In the present study, the highest concentrations of OCP’s are not linked to the seasons of the year but after of the more abundant rains and after the intensive irrigation months of the crops.
A calculated exposure risk below 1 means the absence of carcinogenic and non-carcinogenic risks (USEPA, Guidance for Assessing Chemical Contaminant Data for Use,). At the consumption of 0.204 kg d−1 of contaminated edible tissue of C. bellicosus, the quotients calculated to be below 1 reveal no risk. The highest average OCPs concentration estimated exposure risk (mg kg−1 d−1) for consumption in the 95 percentile corresponded to endosulfan sulphate (49.11), endrin ketone (46.57), endosulfan II (35.02) and metoxichlor (31.71). In the 50 percentile, the highest estimated exposure risk (mg kg−1 d−1) was endosulfan sulphate (13.93) and endrin ketone (9.28). A consumption calculated for one month of an edible portion of crab (higher than 0.27–0.54 mg kg−1) with a concentration of 0.31 mg kg−1 for ∑HCH or 0.51 mg kg−1 for ∑chlordane residues is equivalent to carcinogenic risk concentrations. The ∑DDT showed carcinogenic risk concentrations (0.64 mg kg−1) for a monthly 0.5 mg kg−1 consumption of crab edible portion (>0.47–0.94 mg kg−1). Cancer Benchmark Concentrations were not found for related compounds of ∑aldrin (2.64 mg kg−1) and ∑endosulfan (2.05 mg kg−1) if one portion of crab edible is consumed per month, but there could be a risk when consuming 16 monthly crab edible portions (>1.8–3.5 mg kg−1). Carcinogenic concentrations of ∑heptachlors (0.21 mg kg−1) were calculated consuming crab edible portions higher than 0.021 mg kg−1 month−1. The consumption of edible tissue of crabs above these OPC non-carcinogenic limits constitute a potential human health risk according to the MRLs for Fish and Fishery Products Hazards and Controls Guidance (FDA, Citation2011). Based on our results, on a meal consuming of 8 oz (0.227 kg), as the worst scenario and under average OCPs exposure between 1 × 10−7 and 0.0000248 mg kg−1 d−1, the recommended estimated daily intake (EDI) should be below 0.204 g person−1 day−1. The role played by the blue C. bellicosus in the bioavailability of OCPs has to be studied more precisely and the trophic biomagnification of these pollutants should be analyzed in the aquatic food web in the NAV complex.
Research involving animals
Non vertebrate animals, threatened or endangered according with the International Union for Conservation of Nature (IUCN) policies research involving species at risk of extinction were used in the present experimental studies.
Disclosure statement
All authors have stated that the content of this paper has not been published or submitted for publication elsewhere except as a brief abstract in the proceedings of a scientific meeting or symposium. In addition, all authors contributed equally and significantly, all authors agreed with the content of the manuscript, and declare no financial support or relationships that may pose conflict of interest. Tables and figures have not been reproduced from any other source. No studies involving human participants, human data or human tissue as well as vertebrate animals were carried in this research. All authors have consent for publication and do not contain data from any individual person.
Data availability statement
The data that support the findings of this study are available from the corresponding author, [HAGO], upon reasonable request.
Additional information
Funding
References
- Adeleye, A. O., Sosan, M. B., & Oyekunle, J. A. O. (2019). Dietary exposure assessment of organochlorine pesticides in two commonly grown leafy vegetables in South-western Nigeria. Heliyon, 5(6), e01895. https://doi.org/10.1016/j.heliyon.2019.e01895
- Aguilar-Gonzalez, M. E., LUNA-GONZÁLEZ, A., Aguirre, A., ZAVALA-NORZAGARAY, A. A., MUNDO-OCAMPO, M., & GONZÁLEZ-OCAMPO, H. A. (2014). Perceptions of fishers to sea turtle bycatch, illegal capture and consumption in the san ignacio-navachiste-macapule lagoon complex, Gulf of California, Mexico. Integrative Zoology, 9(1), 70–9. https://doi.org/10.1111/1749-4877.12024
- Arellano-Aguilar, O., Betancourt-Lozano, M., Aguilar-Zárate, G., & Ponce de Leon-Hill, C. (2017). Agrochemical loading in drains and rivers and its connection with pollution in coastal lagoons of the Mexican Pacific. Environmental Monitoring and Assessment, 189(6). https://doi.org/10.1007/s10661-017-5981-8
- ATSDR. (2002). Toxicological profile for aldrin/dieldrin. Agency for toxic substances and disease registry (ATSDR)-U.S. Department of Health and Human Services.
- Barakat, A. O., Khairy, M., & Aukaily, I. (2017). Organochlorine pesticides and polychlorinated biphenyls in sewage sludge from Egypt. Journal of Environmental Science and Health - Part A Toxic/Hazardous Substances and Environmental Engineering, 52, 750–756. https://doi.org/10.1080/02772248.2016.1156683
- Carrasquilla-Henao, M., González Ocampo, H. A., Luna González, A., & Rodríguez Quiroz, G. (2013). Mangrove forest and artisanal fishery in the southern part of the Gulf of California, Mexico. Ocean & Coastal Management, 83, 75–80. https://doi.org/10.1016/j.ocecoaman.2013.02.019
- Chen, J., Chen, L., Liu, D., & Zhang, G. (2014). Organochlorine pesticide contamination in marine organisms of Yantai coast, northern Yellow Sea of China. Environmental Monitoring and Assessment, 186(3), 1561–1568. https://doi.org/10.1007/s10661-013-3473-z
- Dias, P. S., Cipro, C. V. Z., Taniguchi, S., & Montone, R. C. (2013). Persistent organic pollutants in marine biota of São Pedro and São Paulo Archipelago, Brazil. Marine Pollution Bulletin, 74(1), 435–440. https://doi.org/10.1016/j.marpolbul.2013.06.025
- EC., maximum residue levels of pesticides in or on food and feed of plant and animal origin and amending Council Directive 91/414/EEC. In: European, (Ed.), Vol. 396/2005. Official Journal of the European Union 2005, pp. 70.
- Faroon, O., & Harris, M. O. (2002). Toxicological profile for DDT, DDE, and DDD (pp. 497). U.S. Department of Health and Human Services.
- FDA. (2011). Fish and Fishery Products Hazards and Controls Guidance (pp. 468). U.S. Food and Drug Administration.
- G20, The G20 countries. 2017, pp. The G20 is the central forum for international cooperation on financial and economic issues. The G20 countries account for more than four-fifths of gross world product and three-quarters of global trade, and are home to almost two-thirds of the world’s population.
- Garcia Hernandez, J, Espinosa Romero, M. J., Cisneros Mata, M. A., Leyva Garcia, G., Aguilera Marquez, D., & Torre Cosio, J. (2015). Concentration of mercury and organochlorine pesticides (POC) in edible tissue of jaiba coffee Callinectes bellicosus off the coast of Sonora and Sinaloa Mexico [Concentración de mercurio y plaguicidas organoclorados (POC) en tejido comestible de jaiba cafe Callinectes bellicosus de las costas de Sonora y Sinaloa México]. http://dspace.uan.mx:8080/jspui/handle/123456789/2234
- Ghadiri, H. (2001). Degradation of endosulfan in a clay soil from cotton farms of western Queensland. Journal of Environmental Management, 62(2), 155–169. https://doi.org/10.1006/jema.2001.0428
- Granados-Galván, I. A., Rodríguez-Meza, D. G., Luna-González, A., & González-Ocampo, H. A. (2015). Human health risk assessment of pesticide residues in snappers (Lutjanus) fish from the Navachiste Lagoon complex, Mexico. Marine Pollution Bulletin, 97(1–2), 178–187. https://doi.org/10.1016/j.marpolbul.2015.06.018
- Kachi, N., Kachi, S., & Bousnoubra, H. (2016). Effects of irrigated agriculture on water and soil quality (case perimeter Guelma, Algeria). Soil and Water Research, 11(No. 2), 97–104. https://doi.org/10.17221/81/2015-SWR
- Kafaei, R., Arfaeinia, H., Savari, A., Mahmoodi, M., Rezaei, M., Rayani, M., Sorial, G. A., Fattahi, N., & Ramavandi, B. (2020). Organochlorine pesticides contamination in agricultural soils of southern Iran. Chemosphere, 240, 124983. https://doi.org/10.1016/j.chemosphere.2019.124983
- Kannan, K., Tanabe, S., Quynh, H. T., Hue, N. D., & Tatsukawa, R. (1992). Residue pattern and dietary intake of persistent organochlorine compounds in foodstuffs from Vietnam. Archives of Environmental Contamination and Toxicology, 22(4), 367–374. https://doi.org/10.1007/BF00212555
- Kannan, K., Tanabe, S., Williams, R. J., & Tatsukawa, R. (1994). Persistant organochlorine residues in foodstuffs from Australia, Papua New Guinea and the Solomon Islands: Contamination levels and human dietary exposure. Science of the Total Environment, 153(1–2), 29–49. https://doi.org/10.1016/0048-9697(94)90099-X
- Khairy, M. A., Noonan, G. O., & Lohmann, R. (2019). Uptake of hydrophobic organic compounds, including organochlorine pesticides, polybrominated diphenyl ethers, and perfluoroalkyl acids in fish and blue crabs of the lower Passaic River. Environmental toxicology and chemistry. 38, 872–882. https://doi.org/10.1002/etc.4354
- Kilercioglu, B. G, Cengizler, I., Daglioglu, N., & Kilercioglu, S. (2018). Organochlorine pesticides and polychlorinated biphenyls in blue crabs callinectes sapidus (Rathbun, 1896) from Akyatan Lagoon in the Eastern Mediterranean region of Turkey. Mediterranean Marine Science, 19, 376–382. https://doi.org/10.12681/mms.15516
- Kim, S.-K. (2020). Trophic transfer of organochlorine pesticides through food-chain in coastal marine ecosystem. Environmental Engineering Research, 25(1), 43–51. https://doi.org/10.4491/eer.2019.003
- Lakhlalki, H., Jayed, M., Benbrahim, S., Rharbi, N., Benhra, A., Moutaki, B., & Maanan, M. (2020). Assessment of contamination by organochlorine pesticides and polychlorinated biphenyl’s from Oualidia lagoon water (Morocco). Arabian Journal of Geosciences, 13(16), 821. https://doi.org/10.1007/s12517-020-05761-5
- Lee, H.-S., Jeon, H.-J., Lee, H.-S., & Lee, S.-E. (2015). Pesticide-originated persistent organic pollutants in agricultural waterways in chungcheong province, Korea. Journal of Applied Biological Chemistry, 58(4), 291–294. https://doi.org/10.3839/jabc.2015.046
- Leung, M., Nøst, T. H., Wania, F., Papp, E., Herzke, D., Mahmud, A. A., & Roth, D. E. (2020). Maternal-Child Exposures to Persistent Organic Pollutants in Dhaka, Bangladesh. Exposure and Health, 12(1), 79–87. https://doi.org/10.1007/s12403-018-0286-x
- Leyva Morales, J. B, García de la Parra, L. M., Bastidas Bastidas, P. de J., Astorga Rodríguez, J. E., Bejarano Trujillo, J., Cruz Hernández, A., Martínez Rodríguez, I. E., & Betancourt Lozano, M. (2014). Pesticide use in a technified agricultural valley in northwestern Mexico [Uso de plaguicidas en un valle agrícola tecnificado en el noroeste de México]. Revista internacional de contaminación ambiental, 30, 247–261. https://www.scielo.org.mx/scielo.php?script=sci_arttext&pid=S0188-49992014000300002&nrm=iso.
- Li, A., Tanabe, S., Jiang, G., Giesy, J. P, & Lam, P. SK. (2011). Persistent organic pollutants in Asia: Sources, distributions, transport and fate. Elsevier. ISBN: 008055113.
- Lupi, L., Bedmar, F., Wunderlin, D. A., & Miglioranza, K. S. B. (2016). Organochlorine pesticides in agricultural soils and associated biota. Environmental Earth Sciences, 75(6). https://doi.org/10.1007/s12665-015-5140-x
- Magalhães, C. A., Taniguchi, S., Cascaes, M. J., & Montone, R. C. (2012). PCBs, PBDEs and organochlorine pesticides in crabs Hepatus pudibundus and Callinectes danae from Santos Bay, State of São Paulo, Brazil. Marine Pollution Bulletin, 64(3), 662–667. https://doi.org/10.1016/j.marpolbul.2011.12.020
- Montes, A., González-Farias, F., & Botello, A. (2012). Pollution by organochlorine pesticides in Navachiste-Macapule, Sinaloa, Mexico. Environmental Monitoring and Assessment, 1–11. https://doi.org/10.1007/s10661-011-2046-2
- Moon, H.-B., Kim, H.-S., Choi, M., Yu, J., & Choi, H.-G. (2009). Human health risk of polychlorinated biphenyls and organochlorine pesticides resulting from seafood consumption in South Korea, 2005–2007. Food and Chemical Toxicology: an International Journal Published for the British Industrial Biological Research Association, 47(8), 1819–1825. https://doi.org/10.1016/j.fct.2009.04.028
- Nayyar, N., Sangwan, N., Kohli, P., Verma, H., Kumar, R., Negi, V., Oldach, P., Mahato, N. K., Gupta, V., & Lal, R. (2014). Hexachlorocyclohexane: Persistence, toxicity and decontamination. Reviews on Environmental Health, 29(1–2), 49–52. https://doi.org/10.1515/reveh-2014-0015
- Polder, A., Müller, M. B., Lyche, J. L., Mdegela, R. H., Nonga, H. E., Mabiki, F. P., Mbise, T. J., Skaare, J. U., Sandvik, M., Skjerve, E., & Lie, E. (2014). Levels and patterns of persistent organic pollutants (POPs) in tilapia (Oreochromis sp.) from four different lakes in Tanzania: Geographical differences and implications for human health. Science of the Total Environment, 488-489, 252–260. https://doi.org/10.1016/j.scitotenv.2014.04.085
- Reyes-Montiel, N. J., Santamaría-Miranda, A., Rodríguez-Meza, G. D., Galindo-Reyes, J. G., & González-Ocampo, H. A. (2013). Concentrations of organochlorine pesticides in fish (Mugil cephalus) from a coastal ecosystem in the southwestern Gulf of California. Biology & environment proceedings of the royal Irish Academy. 113B, 1–11. https://doi.org/10.3318/BIOE.2013.25
- Saber, T. M., Khedr, M. H. E., & Darwish, W. S. (2018). Residual levels of organochlorine pesticides and heavy metals in shellfish from Egypt with assessment of health risks. Slovenian Veterinary Research, 55(2), 101–113. https://doi.org/10.26873/SVR-453-2017
- SIAP. Date of planting and harvesting in Mexico [Fecha de Siembras y Cosechas en México]. Vol. 2019. SIAP-SAGARPA 2018. https://www.gob.mx/siap/articulos/fecha-de-siembras-y-cosechas-en-mexico?idiom=es
- Silveira-Gramont, M. I., Aldana-Madrid, M. L., Piri-Santana, J., Valenzuela-Quintanar, A. I., Jasa-Silveira, G., & Rodríguez-Olibarria, G. (2018). PLAGUICIDAS AGRICOLAS: UN MARCO DE REFERENCIA PARA EVALUAR RIESGOS A LA SALUD EN COMUNIDADES RURALES EN EL ESTADO DE SONORA, MÉXICO. Revista internacional de contaminación ambiental, 34(1), 7–21. https://doi.org/10.20937/RICA.2018.34.01.01
- Taylor, J., Kedderis, L. B. (1994). United States. Dept. of Health Human Services United States. Public Health Service United States. Agency for Toxic Substances Disease Registry Research Triangle Institute. Toxicological Profile for Endrin and dendrin aldehyde. US Departament of Health & Human Services. https://books.google.com.mx/books?id=FnX6nlnl6mcC
- Tekin, S., & Pazi, I. (2017). POP levels in blue crab (Callinectes sapidus) and edible fish from the eastern Mediterranean coast. Environmental Science and Pollution Research, 24(1), 509–518. https://doi.org/10.1007/s11356-016-7661-6
- Toan, V. D. (2015). Residue of select organochlorine pesticides (OCPs) in sediment from Vietnam’s CauBay river and their impact on agricultural soil and human health [Article]. Polish Journal of Environmental Studies, 24(1), 301–306. https://doi.org/10.15244/pjoes/28119
- USEPA. (1997). Toxicological Review of Chlordane (Technical) (pp. 73). U.S. Environmental Agency.
- USEPA. (2000).Guidance for Assessing Chemical Contaminant Data for Use in Fish Advisories: Risk Assessment and Fish Consumption Limit. In 2. Monitoring Strategy (Vol. 2) (pp. 383). ISBN: EPA 823-B-94-004. Unites States Environmental Agency. https://nepis.epa.gov/Exe/ZyPURL.cgi?Dockey=20003OMP.txt
- USEPA. (2007). Method 8081B, Revision 2: Organochlorine Pesticides by Gas Chromatography, part of Test Methods for Evaluating Solid Waste, Physical/Chemical Methods. United States Environmental Protecction Agency. https://www.epa.gov/sites/production/files/2015-12/documents/8081b.pdf
- USEPA. (2018). Regional Screening Levels (RSLs)–Generic Tables. USA Environmental Protection Agency. https://www.epa.gov/risk/regional-screening-levels-rsls
- USEPA, Integrated Risk Information System (IRIS). (2013). Full list of IRIS Chemicals. U.S.A. Environnmental Protection Agency.
- Vasavada, N. (2014a). One-way ANOVA (Analysis of variance) with post-hoc Tukey HSD (Honestly Significant Difference). Test calculator for comparing multiple treatments. https://astatsa.com/OneWay_Anova_with_TukeyHSD/
- Vasavada, N., & Spearman, P. R. (2014b). Kendall’s tau correlations (for paired sample data). https://astatsa.com/CorrelationTest/