ABSTRACT
This study presents the bio-extraction and mineralization properties of 5 different Magnetotactic bacteria (MTB) to extract valuable metals from spent Li-ion and Ni-Cd batteries. The powdered spent batteries were incorporated for particle size, FTIR, XRD, and XRF analysis. Further, MTB (1 × 107CFUs/ml) was treated individually with the powdered battery sample, and the bioabsorption results showcased the successful removal of lead (98%), nickel (90%), cadmium (97%), zinc (100%), and lead/cadmium (33%) from MSR1, RJS2, RJS5, RJS6, and RJS7, respectively. In addition, the MAG1 consortium treatment effectively removed cadmium (98.5%) and lead (60%). Also, the MAG2 consortium treatment removed cadmium (100%), followed by copper (67%). Similarly, MTB treated with Ni-Cd battery showed the strains RJS5 and MSR1 removed 60% and 66% of cadmium; furthermore, RJS2 and RJS6 removed 72% and 71.4% nickel, respectively. Finally, the RJS7 strain removed 100% lead and 75% cadmium. However, the Ni-Cd battery treated with MAG1 showed effective removal of 67.07% copper and 65% cadmium. Similarly, the MAG2 consortium removed 87% lead and 71% cadmium. The final SEM-EDX and XRF analysis on the Li-ion batteries showed that the RJS2 and RJS5 strains removed zinc and lead effectively. Also, the RJS5 and RJS7 strains treated with the Ni-Cd battery showed better removal of lead and manganese, respectively. This is the first report on the Magnetotactic bacteria to be used for the bio-extraction of valuable metals from spent battery waste.
Introduction
Electronic gadgets such as mobile phones, televisions, laptops/computers, gaming consoles, analytical instruments, and entertainment units have become unavoidable components in our lives. Which aids and assists in better communication, reduction of human efforts, safety, and entertainment applications (Heelan et al., Citation2016; Jhu et al., Citation2011; Kuzuhara et al., Citation2018; Winslow et al., Citation2018; Zubi et al., Citation2018). In addition, the demand for electronic devices is rising in economies such as Europe, China, India, Brazil, and South Africa, which contributes to enormous waste of electrical and electronic equipment (WEEE) (S. K. Ghosh et al., Citation2016). Switching electronic gadgets due to the end of their life or due to technological upgrades has become more common, which generates vast quantities of electronic waste (Bertuol et al., Citation2006; Liang et al., Citation2019). Such e-waste that is in its end-of-life period or no longer fit for use is destined for recovery, recycling, or disposal (Agnihotri, Citation2011). The e-waste contains several main components like printed circuit board (PCB), plastic outer casing, metal parts, and batteries. The batteries are the major components and power source of any electronic device, without which it cannot function. In addition, these batteries are present in a wide range of sizes, shapes, and voltages depending on which they operate, starting from button cells (CR2032 and LR41), A27, AAA, and AA to huge automobile batteries. Effective waste recycling strategies have been implemented for recycling plastics, metals, and PCBs, but technological advancements have not been effectively advanced in recycling batteries. Commonly known and available battery chemistry are lead—acid, lithium—ion, lithium—titanate (Li2TiO3), lithium—manganese oxide (LiMn2O4), nickel—cadmium (NiCd), and nickel—metal hydride (NiMH) batteries. Many prominent physical and chemical methods, such as pyrometallurgy, hydrometallurgy, and thermal treatment of spent batteries, have been employed to reduce, recycle and reuse the metals (mercury, arsenic, cadmium, selenium, cobalt, lithium, copper, nickel, and manganese) present in the spent batteries (Assefi et al., Citation2018; Chen et al., Citation2018; Dang et al., Citation2020; Martinez-Laserna et al., Citation2018; Meshram et al., Citation2015). But, these techniques are expensive and require intense energy, skilled labour, and higher running costs. Improper disposal of the spent batteries has a higher risk of explosion and leaching of metals or electrolytes (LiPF6) into soil and groundwater, causing severe environmental pollution (E-Waste Fact Sheet, Citation2009; B. Ghosh et al., Citation2015; Nshizirungu et al., Citation2020; Roy et al., Citation2021). Furthermore, exposure to such metals through food and water beyond their permissible limit may cause several health issues, such as cancer, acute kidney failure, and respiratory diseases (Needhidasan et al., Citation2014; Pant et al., Citation2012). Therefore, an eco-friendly and economical metal removal recycling and recovery approach is needed to extract valuable metals from spent batteries.
Bio extraction studies on the recovery of metals using bacteria and fungal species have provided promising results. They can become a promising, cost-effective technique without using harsh chemicals and higher temperatures. However, very few microbes have been reported to have such functional properties, and their yield is significantly lower. The Magnetotactic bacteria (MTB) are Gram-negative, motile bacteria that can align themselves perfectly on the oxic—anoxic gradient using the earth’s geomagnetic field using nanoparticles such as magnetite (Fe3O4) and greigite (Fe3S4) (Schüler & Frankel, Citation1999; Zhou et al., Citation2012). Despite their nanoparticle potential, they have been widely used in therapeutics and bioremediation prospects (Ali et al., Citation2018; Bazylinski & Schubbe, Citation2007; Kopp & Kirschvink, Citation2008; X. Wang et al., Citation2020). Bahaj et al (Citation1991, Citation1994, Citation1998). studied the ability of MTB to remove metals from wastewater, and similar studies focusing on the adsorption of heavy metals were also investigated (Arakaki et al., Citation2002; Boateng et al., Citation2019; Diaz-Alarcón et al., Citation2019; Keim & Farina, Citation2005; Song et al., Citation2008; Tajer-Mohammad Ghazvini et al., Citation2016; Wang et al., Citation2011). This research study focuses on the extraction of valuable metals from the spent lithium-ion and nickel-cadmium batteries using the individual and consortium of Magnetotactic bacteria. So far, this is the first report from India focusing on bioremediation and recovery of valuable metals using the MTB.
Materials and methods
Source, collection, and analysis of spent battery sample
Spent Li-ion (Nokia BL-5C, 3.7 V) and Ni-Cd battery (Eveready AA Battery) were collected from the local market in Vellore district, Tamilnadu. Plastic parts were carefully removed from the batteries. Metal parts were subjected to size reduction using a hammer mill and finely powdered. Particle size was analyzed using a particle size analyzer (HORIBA SCIENTIFIC SZ-100, Japan). The powder from the Li-ion and Ni-Cd battery was for metal composition using FTIR (Schimadzu, Japan), XRD (BRUKER, Germany), and X-ray 95 fluorescence (XRF, XGT-5200, HORIBA, Japan). Further to 1 g of homogenized powder (Li-ion and Ni-Cd) a few drops of HNO3 were added, and the volume was made to 100 ml with double distilled water. Finally, the sample was filtered (>100 µm) and subjected to metal analysis using AAS (VARIAN SPECTRAA240, Australia) (Adhapure et al., Citation2013).
Microorganisms, consortium, and culture condition
The strain Magnetospirillum gryphiswaldense (MSR-1) was purchased from Deutsche Sammlung von Mikroorganismen und Zellkulturen (DSMZ) Germany: other strains, Magnetospirillum sp. RJS2 (KJ570852), Magnetospirillumsp. RJS5 (KM289194), Magnetospirillum sp. RJS6 (KT266803) and Magnetospirillum sp. RJS7 (KT693285) was isolated and maintained in the laboratory (Jacob et al., Citation2016). The strains were cultured in Magnetospirillum growth medium (MSGM) till they reached 1 × 108 CFUs/ml (Blakemore et al., Citation1979) and oxygen sulphide gradient medium (OSGM) (Schuler et al., Citation1999) using the Hungate anaerobic technique (Hungate, Citation1950). Two sets of consortia, MAG1 and MAG2, were also formed (Sannigrahi & Suthindhiran, Citation2019). The MAG1 consortium contains RJS2, RJS5, and MSR-1 with a final cell concentration of 1.5 × 107 CFUs/ml, and the MAG2 consortium contains RJS6 and RJS7 with a final cell concentration of 1.5 × 107 CFUs/ml. Based on the growth requirements, the strains were maintained in MSGM or OSGM. All the chemicals were obtained from Himedia Laboratories, India, until and unless specified.
The bio removal of metal for MTBs and its consortia
The biodegradation batch experiment using MTBs and their consortia was performed using a modified protocol followed by Sannigrahi and Suthindhiran (Citation2019). MSGM media (pH 6.7) and oxygen sulphide gradient medium (pH 7) were transferred in separate sets of serum bottles. The digested samples (Li-ion and Ni-Cd battery) were added separately in a 1:10 ratio to each set of the medium. The pH of the respective medium was again adjusted after adding the digested samples. Nitrogen gas was sparged into bottles and sealed with aluminium caps to maintain microaerophilic conditions. Sterile vitamin solution and the ferric quinate solution were added to the sterilized setup of MSGM. Sterile vitamin solution, ferric citrate solution, and cysteine HCl solution were added to the sterilized setup of OSGM. Culture (1 mL − 1 × 107 CFUs/ml) was inoculated to each bottle except for the specified control. Two sets of control were kept along with the experimental setup for comparison. The first control was maintained without the Li-ion/Ni-Cd battery (media + culture), while the other set had no bacterial culture inoculated (media + E-waste). The inoculated bottles were incubated in an orbital shaker at 28 ± 1°C with 120 rpm for 12 days, and the growth of the culture was regularly monitored.
Further, the metal recovery from the Li-ion and Ni-Cd battery using consortia was also performed. Finally, the treated Li-ion and Ni-Cd battery samples were centrifuged (6708g for 10 min) to separate the MTB cells from unutilized batteries. The supernatant was separated, and AAS was used to analyze the initial and final metal content. The MTB cells were fixed on the cover slide using 0.25% glutaraldehyde and analyzed through SEM-EDX (Tescan VEGA 3SBH, BrukerEasy EDS, Czech Republic) Sannigrahi and Suthindhiran (Citation2019). The metal removal in each MTB strain was analyzed through XRF analysis from the protocol followed in the previous study (Sannigrahi & Suthindhiran, Citation2019).
Results
Particle size and metal content analysis in Li-ion battery
The particle size analysis of the prepared Li-ion battery suggested to have a size of 3017.5 diameters (nm) (). Also, the FTIR spectrum from 500 cm−1 to 4000 cm−1 of the powdered Li-ion battery recorded the presence of cadmium (Cd(II)) [3479.58 cm−1], lithium (LiOCH3) [2931.80 cm−1], zinc (Zn(O2CCH3)2) [1629.85 cm−1], copper (Cu2+) [1402.25 cm−1], zinc (ZnO) [1043.49 cm−1] and lead (Pb(NO3))2 [867.97 cm−1] (). In addition, the XRD analysis of powdered Li-ion battery confirmed the presence of lithium (KLiO5Si2O2), chromium (CrHgO6Pb2), lead (Pb2SO5), silica (SiO2), and copper (CuTe2O5) (). Also, the XRF analysis confirmed the presence of manganese, copper, zinc, lead, cadmium, and nickel (). Finally the AAS analysis of Li-ion battery revealed the presence of a higher concentration of copper and zinc followed by, in contrast the cadmium and lead were found to be low.
Figure 1b. FTIR analysis of spent Li-ion battery shows the presence of different metals in the form of compounds. Metals such as cadmium as cadmium (II) form, at 3479.58 cm−1, lithium as lithium methoxide form, at 2931.80 cm−1, zinc as zinc acetate at 1629.85 cm−1 and zinc oxide at 1043.49 cm−1, copper as Cu2- form, at 1402.25 cm−1, lead as lead nitrate at 867.97 cm−1 has been reported in spent Li-ion battery.
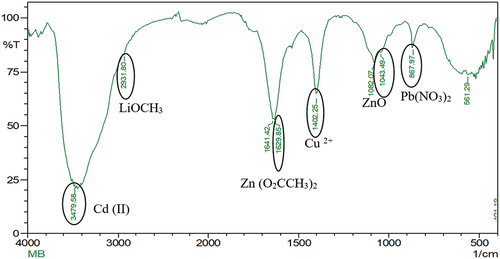
Particle size and metal analysis in Ni-Cd battery
Similarly the particle size analysis of the powdered spent Ni-Cd battery was estimated to be 5185.3 diameters (nm) (). In contrast, the FTIR spectrum of Ni-Cd batteries showed the presence of cadmium (Cd (II)) [3421.72 cm−1], lithium (LiOCH3) [2924.09 cm−1], zinc (Zn(O2CCH3)2) [1631.78 cm−1], copper (Cu2+) [1400.32 cm−1], zinc (ZnO) [1039.63 cm−1], nickel (NiO) [617.22 cm−1] and lead (2PbCO3 Pb (OH)2) [565.14 cm−1] (). Further, XRD analysis of spent Ni-Cd battery () showed the presence of lithium, silicon, and aluminium in LiSi2AlO7H2 form; rubidium, and zinc in O14P4Rb2Zn3 form; lead, titanium arsenic, and uranium in PbTiOAsO4 and U4PbU19H10 form; and various other metals present in CsLaO12P4 form. In continuation, the XRF analysis showed the presence of lead, copper, zinc, nickel, cadmium, and manganese in the Ni-Cd battery and finally, the AAS analysis verified the presence of cadmium, copper, lead, and zinc ( and Supplementary S1).
Figure 2b. FTIR analysis of spent Ni-cd battery shows the presence of different metals in the form of compounds. Metals such as cadmium as cadmium (II) form at 3421.72 cm−1, lithium as lithium methoxide form at 2924.09 cm−1, zinc as zinc acetate at 1631.78 cm−1 and zinc oxide at 1039.63 cm−1, copper as Cu2+ form at 1400.32 cm−1, nickel as nickel oxide at 617.22 cm−1, lead as lead white at 565.14 cm−1 has been reported in Ni-cd battery.
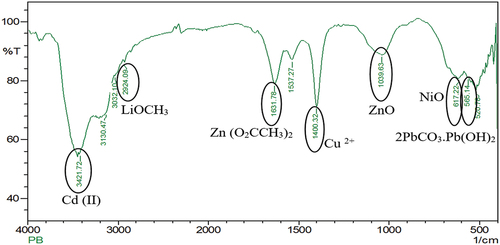
Treatment of Li-ion battery with individual strains
The bio extraction of metals from the spent Li-ion battery using the MTB was identified using the AAS, and the results are presented in . Results showcased that the strain MSR-1 was able to remove 98% of lead, 42% of copper, and 22% of zinc. Similarly, the RJS2 strain recovered 90% of nickel, 67% of lead, 44% of copper, and 15% of zinc. The RJS5 strain removed cadmium (97%), nickel (73%), copper (24%), and lead (2%), except zinc. The RJS6 strain removed zinc (100%), lead (40%), copper (19%), cadmium (6%), and nickel (2%). Finally, the RJS7 strain removed 33% of lead and cadmium, 13.3% of nickel, 3.5% of zinc, and 2.4% of copper. From these results, we were able to interpret that among the five metals, lead and nickel were extracted the most using the MTB from the spent Li-ion battery.
Table 1. Metal removal in different strains of MTB from spent Li-ion battery
The bio-recovery studies suggest that all the strains were able to extract copper and lead in various concentrations from the spent Li-ion battery. The RJS2 and MSR1 strains effectively removed copper (44% and 42%) and lead (67% and 98%), respectively. Similarly, effective removal of cadmium, nickel, and zinc was observed from the strains RJS5 (97%), RJS2 (90%), and RJS6 (100%), respectively. Finally, the strains MSR1 showed negligible or no absorption of cadmium and nickel. A similar result with no absorption of zinc was also recorded from the RJS5 strain.
Treatment of Li-ion battery using consortia
The MAG1 consortium formulated using the RJS2 (1 × 108 CFUs/ml), RJS5 (1 × 108 CFUs/ml), and MSR1 (1 × 108 CFUs/ml) strains with the final consortium concentration of 1.5 × 107 CFUs/ml showed significant removal of metals from the spent Li-ion battery (Table ). At the same time, the MAG1 consortium showed higher removal of cadmium (98.5%), followed by lead (60%), nickel (31%), zinc (16%), and copper (15%). Similarly, the second set of consortiums formulated using RJS6 (1 × 108 CFUs/ml) and RJS7 (1 × 108 CFUs/ml) strains with the final consortium concentration (1.5 × 107 CFUs/ml) showed effective removal of lead (100%), followed by copper (67%), zinc (27%), cadmium (21%), and nickel (14%).
Table 2. Metal removal in MAG1 and MAG2 consortium from spent Li-ion battery
Treatment of Ni-Cd battery with individual strains
Similarly, the bio-extraction studies from the spent Ni-Cd battery using the MTB strain analyzed using the AAS studies are mentioned in Table . The RJS2 strain removed the highest concentration of nickel (72%), followed by zinc (46%) and copper (34%). Meanwhile, the MSR1 strain showed lesser removal of nickel (5.2%). In addition, higher cadmium removal was observed in RJS7 (75%), MSR-1 (66%), and RJS5 (60%) strains. On the contrary, the least cadmium was absorbed by RJS6 (2.7%) and RJS2 (0%). Among the selected MTB strains, the RJS7 strain effectively removed all the metals lead (100%), cadmium (75%), nickel (22%), zinc (11.26%), and copper (9.6%). Followed by the RJS5 strain removed cadmium (60%), copper (55%), nickel (50%), zinc (36%), and lead (5%). In addition, the MSR1 strain removed cadmium (66%), effectively followed by copper (50.3%), lead (40%), zinc (15%), and nickel (5.2%). Finally, the RJS6 strain effectively removed nickel (71.4%), followed by copper (20.2%), lead (9%), cadmium (3.4%), and zinc (2.7%).
Table 3. Metal removal in different strains of MTB from spent Ni-cd battery
Treatment of Ni-Cd battery using consortia
Furthermore, bio-extraction of metal from the spent nickel-cadmium powder using the MAG1 consortium (1.5 × 107 CFUs/ml) showed significant removal of copper (67%), nickel (51%), and lead (20%) (Table ). However, it showed inefficient removal of cadmium and low removal of zinc (8.7%). In comparison with individual strains (RJS2, RJS5, and MSR-1), the removal of copper, nickel, and lead was higher in the MAG1 consortium. However, cadmium and zinc removal was reduced in the MAG1 consortium. The removal of copper was maximum in the MAG1 consortium, but the production of magnetosomes was suppressed. The MAG2 consortium (1.5 × 107 CFUs/ml) treatment showed enhanced removal of copper (50.4%) and nickel (57.14%) compared to the individual strains (RJS6 and RJS7). Removal was elevated at approximately 35% for copper and 11% for nickel. The consortium showed low removal of cadmium, lead, and zinc (Table ). The individual strains showed better removal of cadmium, lead, and zinc compared to the microbial consortium treatment.
Table 4. Metal removal in MAG1 and MAG2 consortium from spent Ni-Cd battery
SEM-EDX and XRF analysis
Based on the AAS study results of the spent Li-ion batteries and MTB treatment, RJS2 and RJS5 strains were selected for further SEM-EDX studies (Figure ). The removal of zinc was maximum in the RJS2 strain, followed by manganese, lead, iron, nickel, and copper. In RJS5, the removal of lead was maximum, followed by zinc, manganese, iron, cadmium, copper, and nickel.
Figure 3. SEM-EDX analysis of (a) RJS2 from Li-ion battery, (b) RJS5 from Li-ion battery, (c) RJS5 from Ni-Cd battery, (d) RJS7 from Ni-Cd battery.
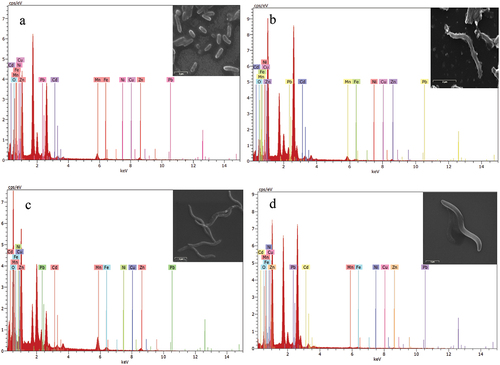
In the case of the Ni-Cd battery, RJS5 and RJS7 strains were selected for the SEM-EDX based on AAS analysis (Figure ). The strain RJS5 showed good removal of manganese, zinc, lead, iron, cadmium, and copper. In the RJS7 strain, the removal of lead was maximum, followed by zinc, iron, cadmium, manganese, and copper. Removal of nickel was negligible in both strains.
The removal of metals in individual MTB strains from Li-ion battery and Ni-Cd battery was also verified in XRF analysis (Table ). In the case of Li-ion batteries, metals like copper, manganese, and zinc were removed in good quantity. Moreover, the strains RJS2, RJS5, and RJS6 were capable of removing most of the metals. Next, in the case of the Ni-Cd battery, zinc and copper were successfully removed. Comparatively, other metals like lead, nickel, and copper showed low removal. The MTB strains, RJS2, RJS7, and MSR-1 removed the maximum quantity of metals. Among all the strains, only the RJS7 strain was capable of removing cadmium from the Ni-Cd battery.
Table 5. XRF analysis of Li-ion and Ni-Cd battery treated with MTB strains
Discussion
Metal removal studies from Li-ion batteries using conventional microorganisms such as sulfur-oxidizing and iron-oxidizing bacteria have been widely explored (Biswal et al., Citation2018; Wu et al., Citation2019). However, most of these studies are limited to the removal of cobalt and lithium from spent batteries. The Ni-Cd battery is known to have an abundance of cadmium present in it (Hazotte et al., Citation2016), but the removal of cadmium has always been a tedious process.
The current study presents a simple strategy for the bio removal of cadmium along with other metals- copper, nickel, lead, and zinc from Li-ion batteries and Ni-Cd batteries. This is the first report wherein magnetotactic bacteria is used for the bio removal of metals from Li-ion and Ni-Cd batteries. The preliminary studies has verified the presence of lithium, cadmium, zinc, nickel, lead, mercury, and copper in spent Li-ion batteries and Ni-Cd batteries using particle size, FTIR, XRD, and XRF analysis (Recknagel et al., Citation2014; Rudnik & Knapczyk-Korczak, Citation2019; Tanong et al., Citation2017; Weshahy et al., Citation2022). Nickel is one of the most expensive metals due to its extensive use in the stainless steel industry (Watling, Citation2008). However, frequent recycling or reuse of nickel is not mainly achieved as per its demand (Elshkaki et al., Citation2017; Sakunai et al., Citation2021). The MTB strains RJS2 and RJS5 used in our study exhibited 70–90% bio removal of nickel from the spent Li-ion battery. In contrast the RJS2 and RJS6 strains showed > 70% bio removal of nickel from the Ni-Cd battery. RJS2 is the prominent strain that removes 90% of nickel from the Li-ion battery. In contrast, previous studies showed low (38%, 23%) or negligible bioleaching of nickel from Li-ion and Ni-Cd batteries (Chen et al., Citation2015; Horeh et al., Citation2016; Randhawa et al., Citation2016; Shin et al., Citation2005).
On the contrary, in nickel bio removal, specific reports retain 84.3% removal of cadmium from spent batteries (Rahangdale & Kumar, Citation2018; Paul et al., Citation2020). However, it is time-consuming, expensive, and produces hazardous chemicals. The MAG1 consortium and strain RJS5, on the other hand, exhibit 98.5% removal of cadmium using a simple, eco-friendly, cost-effective biological process. Similarly, a good amount of cadmium is also removed from the Ni-Cd battery when treated with strains MSR-1, RJS5, and RJS7. Lead is one of the toxic metals that poses a real threat during disposal of the batteries (Ara & Usmani, Citation2015; Fujimori et al., Citation2016; Rahman & Singh, Citation2019). It is noteworthy to point out that the bio removal of lead from spent batteries is left unexplored. In our study, we highlighted the strains MSR-1 and MAG2 consortium, which displayed 100% removal of lead from the Li-ion battery. Also, the strain RJS7 exhibited 100% removal of lead from the Ni-Cd battery.
The Aspergillus niger and Aspergillus tubingensis were able to remove 90% of zinc from spent batteries, but the strain RJS6 exhibited 100% removal of the metal (Kim et al., Citation2016). Copper removal by conventional microorganisms such as A. ferrooxidans, A. thiooxidans, and A. ferrivorans is standard (Hong & Valix, Citation2014), while the mixture of both A. thiooxidans and A. ferrivorans formed could remove 98.4% of copper (Işıldar et al., Citation2016).
Likewise, a comparative biosorption study with individual MTB strains and their consortium (MAG1 and MAG 2) depicted higher copper bio-removal efficiency of the latter from spent batteries. The removal of copper from the Ni-Cd battery was also influential in two individual strains, MSR-1 and RJS5. The evident functioning of both consortia was also observed for other metals, namely cadmium and lead from Li-ion battery and nickel from Ni-Cd battery. However, the magnetic moment in the consortium was visibly low. It could be speculated for the adaption of MTB to a new environment. Xin et al. (Citation2012) reported a reduced leaching rate of Alicyclobacillus sp. and Sulfobacillus sp. when exposed to high pulp-density electronic waste with a toxic and acidic nature. Lately, specific reports have proposed that increased leaching of E-waste could be achieved by various pre-adaptation processes for microbes, adjustment of media pH, and removal of non-metallic components (Baniasadi et al., Citation2019; Hansda et al., Citation2016; Teimouri et al., Citation2023).
The SEM-EDX and XRF analysis showed the maximum removal of cadmium, copper, manganese, and zinc metals by the MTBs. The uptake of manganese and zinc in MTBs could be the result of nutrient imbalance and low exposure to iron (Berny et al., Citation2020; Deveci et al., Citation2004; Prozorov et al., Citation2014). High copper uptake could be due to the presence of ChpA (copper handling proteins) in MTBs (Dubbels et al., Citation2004). Compared to the previous reports of bioleaching from spent batteries, our study shows Magnetospirillum sp. as a promising candidate for lead removal from Li-ion batteries and nickel as well as cadmium removal from Ni-Cd batteries. The individual strains, as well as the consortium, present a huge removal of lead and nickel from Li-ion batteries and Ni-Cd batteries, respectively. On average, among the five individual strains, strain RJS2 presents the maximum metal removal from the Li-ion battery, and strain RJS7 exhibits the maximum removal of metals from the Ni-Cd battery. Moreover, the application of both consortias reveals better removal of metals- copper, cadmium, and lead from Li-ion batteries, metals-coppers, and nickel from Ni-Cd batteries. Among the two groups of consortia, the MAG2 consortium presents better metal removal from the Li-ion battery, whereas the MAG1 consortium presents better metal removal from the Ni-Cd battery.
This study provides a necessary solution to the ongoing economic and ecological problems. These MTBs could be the solution to the ongoing problem of metal generation and reduce the harmful disposition of these metals in the environment (Richa et al., Citation2017). Essential metals like nickel, copper, and zinc could be necessarily recycled from spent batteries by MTBs for use in industries that might result in lesser production of these metals (Richa et al., Citation2017; Salgado et al., Citation2003). Hazardous metals like lead and cadmium could be essentially removed from the waste landfills by implementing these MTBs, thereby restricting these metals from sip in groundwater and soil. From a future perspective, these MTB strains, along with the consortia, can be further applied in the industrial sector to recover precious metals like gold and silver for ecological and economic benefits.
Conclusion
This work attempts to provide an eco-friendly methodology for the removal of metals from the spent Li-ion battery and Ni-Cd battery. The metal content from both the spent batteries is significantly removed after the treatment with the test organisms (RJS2, RJS5, RJS6, RJS7, MSR-1) and their consortia MAG1 and MAG2. The individual strains, along with their consortium, demonstrated effective removal of lead, nickel, copper, zinc, manganese, and cadmium. Further research on determining the influence of various conditions and parameters on the activity of strains, in situ biodegradation, and bioreactor design is underway. The optimization of important parameters in the leaching process would significantly increase the removal of metal percentages. The effective reuse and recycling of these precious metals are necessary elements to achieve a sustainable technology-based society. Economic and ecological benefits, in addition to bio removal, can lead to a wide range of applications in industries. Moreover, the waste generated in the environment via these batteries could be substantially reduced by the proper implementation of MTBs.
Author Contribution:
All authors contributed to the study conception and design. Validation, Investigation and Writing- Reviewing and Editing the final draft were performed by Sumana Sannigrahi, Gargi Sarkar and Dr. K. Suthindhiran. Methodology and Formal analysis were performed by Sumana Sannigrahi and Gargi Sarkar. Writing the Original draft and Data curation were done by Sumana Sannigrahi. Resources, Supervision, Project Administration and Funding Acquisition were done by Dr. K. Suthindhiran.
supplementary Fig.docx
Download MS Word (247.9 KB)Acknowledgements
The authors thank the management of Vellore Institute of Technology for providing FTIR, XRD, particle size analyser, and Technology Business Incubator-VIT to facilitate AAS. We also thank CSIR- Central Electrochemical Research Institute, Karaikudi for carrying out XRF and SEM analysis.
Disclosure statement
No potential conflict of interest was reported by the author(s).
Data availability statement
Data will be made available from the corresponding author on reasonable request.
Supplementary material
Supplemental data for this article can be accessed online at https://doi.org/10.1080/27658511.2024.2345431
Additional information
Funding
References
- Adhapure, N. N., Waghmare, S. S., Hamde, V. S., & Deshmukh, A. M. (2013). Metal solubilization from powdered printed circuit boards by microbial consortium from bauxite and pyrite ores. Applied Biochemistry and Microbiology, 49(3), 256–13. https://doi.org/10.1134/S0003683813030034
- Agnihotri, K. V. (2011). E-Waste in India, research unit, Rajya Sabha Secretariat. Retrieved May 11, 2022, from. https://rajyasabha.nic.in/rsnew/publication_electronic/E-Waste_in_india.pdf
- Ali, I., Peng, C., Khan, Z. M., Naz, I., & Sultan, M. (2018). An overview of heavy metal removal from wastewater using magnetotactic bacteria. Journal of Chemical Technology & Biotechnology, 93(10), 2817–2832. https://doi.org/10.1002/jctb.5648
- Arakaki, A., Takeyama, H., Tanaka, T., & Matsunaga, T. (2002). Cadmium recovery by a sulfate-reducing magnetotactic bacterium, desulfovibrio magneticus RS-1, using magnetic separation. Biotech Fuel Chem, 833–840. https://doi.org/10.1007/978-1-4612-0119-9_67
- Ara, A., & Usmani, J. A. (2015). Lead toxicity: A review. Interdisciplinary Toxicology, 8(2), 55–64. https://doi.org/10.1515/intox-2015-0009
- Assefi, M., Maroufi, S., Mayyas, M., & Sahajwalla, V. (2018). Recycling of Ni-cd batteries by selective isolation and hydrothermal synthesis of porous NiO nanocuboid. Journal of Environmental Chemical Engineering, 6(4), 4671–4675. https://doi.org/10.1016/j.jece.2018.07.021
- Bahaj, A. S., Croudace, I. W., & James, P. A. B. (1994). Treatment of heavy metal contaminants using magnetotactic bacteria. IEEE transactions on magnetics, 30(6), 4707–4709. https://doi.org/10.1109/20.334196
- Bahaj, A. S., Croudace, I. W., James, P. A. B., Moeschler, F. D., & Warwick, P. E. (1998). Continuous radionuclide recovery from wastewater using magnetotactic bacteria. Journal of Magnetism and Magnetic Materials, 184(2), 241–244. https://doi.org/10.1016/S0304-8853(97)01130-X
- Bahaj, A. S., Ellwood, D. C., & Watson, J. H. P. (1991). Extraction of heavy metals using microorganisms and high gradient magnetic separation. IEEE transactions on magnetics, 27(6), 5371–5374. https://doi.org/10.1109/20.278842
- Baniasadi, M., Vakilchap, F., Bahaloo-Horeh, N., Mousavi, S. M., & Farnaud, S. (2019). Advances in bioleaching as a sustainable method for metal recovery from e-waste: A review. Journal of Industrial & Engineering Chemistry, 76, 75–90. https://doi.org/10.1016/j.jiec.2019.03.047
- Bazylinski, D. A., & Schubbe, S. (2007). Controlled biomineralization by and applications of magnetotactic bacteria. Advances in Applied Microbiology, 62, 21–62. https://doi.org/10.1016/S0065-2164(07)62002-4
- Berny, C., Fèvre R, L., Guyot, F., Blondeau, K., Guizonne, C., Rousseau, E., Bayan, N., & Alphandéry, E. (2020). A method for producing highly pure magnetosomes in large quantity for medical applications using Magnetospirillum gryphiswaldense MSR-1 magnetotactic bacteria amplified in minimal growth media. Frontiers in Bioengineering and Biotechnology, 8, 16. https://doi.org/10.3389/fbioe.2020.00016
- Bertuol, D. A., Bernardes, A. M., & Tenorio, J. A. S. (2006). Spent NiMH batteries: Characterization and metal recovery through mechanical processing. Journal of Power Sources, 160(2), 1465–1470. https://doi.org/10.1016/j.jpowsour.2006.02.091
- Biswal, B. K., Jadhav, U. U., Madhaiyan, M., Ji, L., Yang, E. H., & Cao, B. (2018). Biological leaching and chemical precipitation methods for recovery of Co and Li from spent lithium-ion batteries. ACS Sustainable Chemistry & Engineering, 6(9), 12343–12352. https://doi.org/10.1021/acssuschemeng.8b02810
- Blakemore, R. P., Maratea, D., & Wolfe, R. S. (1979). Isolation and pure culture of a freshwater magnetic spirillum in chemically defined medium. Journal of Bacteriology, 140(2), 720–729. https://doi.org/10.1128/jb.140.2.720-729.1979
- Boateng, T. K., Opoku, F., & Akoto, O. (2019). Heavy metal contamination assessment of groundwater quality: A case study of oti landfill site, Kumasi. Applied Water Science, 9(2), 33. https://doi.org/10.1007/s13201-019-0915-y
- Chen, Y., Liu, N., Hu, F., Ye, L., Xi, Y., & Yang, S. (2018). Thermal treatment and ammoniacal leaching for the recovery of valuable metals from spent lithium-ion batteries. Waste Management, 75, 469–476. https://doi.org/10.1016/j.wasman.2018.02.024
- Chen, X., Xu, B., Zhou, T., Liu, D., Hu, H., & Fan, S. (2015). Separation and recovery of metal values from leaching liquor of mixed-type of spent lithium-ion batteries. Sep. Separation and Purification Technology, 144, 197–205. https://doi.org/10.1016/j.seppur.2015.02.006
- Dang, H., Li, N., Chang, Z., Wang, B., Zhan, Y., Wu, X., Liu, W., Ali, S., Li, H., Guo, J., Li, W., Zhou, H., & Sun, C. (2020). Lithium leaching via calcium chloride roasting from simulated pyrometallurgical slag of spent lithium ion battery. Separation and Purification Technology, 233, 116025. https://doi.org/10.1016/j.seppur.2019.116025
- Deveci, H., Akcil, A., & Alp, I. (2004). Bioleaching of complex zinc sulphides using mesophilic and thermophilic bacteria: Comparative importance of pH and iron. Hydrometallurgy, 73(3–4), 293–303. https://doi.org/10.1016/j.hydromet.2003.12.001
- Diaz-Alarcón, J. A., Alfonso-Pérez, M. P., Vergara-Gómez, I., Díaz-Lagos, M., & Martínez-Ovalle, S. A. (2019). Removal of iron and manganese in groundwater through magnetotactic bacteria. Journal of Environmental Management, 249, 109381. https://doi.org/10.1016/j.jenvman.2019.109381
- Dubbels, B. L., DiSpirito, A. A., Morton, J. D., Semrau, J. D., Neto, J. N. E., & Bazylinski, D. A. (2004). Evidence for a copper-dependent iron transport system in the marine, magnetotactic bacterium strain MV-1. Microbiology (Reading, England), 150(9), 2931–2945. https://doi.org/10.1099/mic.0.27233-0
- Elshkaki, A., Reck, B. K., & Graedel, T. E. (2017). Anthropogenic nickel supply, demand, and associated energy and water use. Resources, Conservation and Recycling, 125, 300–307. https://doi.org/10.1016/j.resconrec.2017.07.002
- E-Waste Fact Sheet. (2009). Clean up. Retrieved November 21, 2021, from. https://issuu.com/clean_up/docs/electronic_waste_factsheet
- Fujimori, T., Eguchi, A., Agusa, T., Tue, N. M., Suzuki, G., Takahashi, S., Viet, P. H., Tanabe, S., & Takigami, H. (2016). Lead contamination in surface soil on roads from used lead–acid battery recycling in Dong Mai, Northern Vietnam. Journal of Material Cycles & Waste Management, 18(4), 599–607. https://doi.org/10.1007/s10163-016-0527-7
- Ghosh, S. K., Debnath, B., Baidya, R., De, D., Li, J., Ghosh, S. K., Zheng, L., Awasthi, A. K., Liubarskaia, M. A., Ogola, J. S., & Tavares, A. N. (2016). Waste electrical and electronic equipment management and basel convention compliance in Brazil, Russia, India, China and South Africa (BRICS) nations. Waste Management & Research: The Journal of the International Solid Wastes & Public Cleansing Association, ISWA, 34(8), 693–707. https://doi.org/10.1177/0734242X16652956
- Ghosh, B., Ghosh, M. K., Parhi, P., Mukherjee, P. S., & Mishra, B. K. (2015). Waste printed circuit boards recycling: An extensive assessment of current status. Journal of Cleaner Production, 94, 5–19. https://doi.org/10.1016/j.jclepro.2015.02.024
- Hansda, A., Kumar, V., & Anshumali. (2016). A comparative review towards potential of microbial cells for heavy metal removal with emphasis on biosorption and bioaccumulation. World Journal of Microbiology & Biotechnology, 32(10), 1–14. https://doi.org/10.1007/s11274-016-2117-1
- Hazotte, C., Leclerc, N., Meux, E., & Lapicque, F. (2016). Direct recovery of cadmium and nickel from Ni-Cd spent batteries by electroassisted leaching and electrodeposition in a single-cell process. Hydrometallurgy, 162, 94–103. https://doi.org/10.1016/j.hydromet.2016.02.019
- Heelan, J., Gratz, E., Zheng, Z., Wang, Q., Chen, M., Apelian, D., & Wang, Y. (2016). Current and prospective Li-ion battery recycling and recovery processes. Jom, 68(10), 2632–2638. https://doi.org/10.1007/s11837-016-1994-y
- Hong, Y., & Valix, M. (2014). Bioleaching of electronic waste using acidophilic sulfur oxidising bacteria. Journal of Cleaner Production, 65, 465–472. https://doi.org/10.1016/j.jclepro.2013.08.043
- Horeh, N. B., Mousavi, S. M., & Shojaosadati, S. A. (2016). Bioleaching of valuable metals from spent lithium-ion mobile phone batteries using aspergillus niger. Journal of Power Sources, 320, 257–266. https://doi.org/10.1016/j.jpowsour.2016.04.104
- Hungate, R. E. (1950). The anaerobic mesophilic cellulolytic bacteria. Bacteriological Reviews, 14(1), 1. https://doi.org/10.1128/br.14.1.1-49.1950
- Işıldar, A., van de Vossenberg, J., Rene, E. R., van Hullebusch, E. D., & Lens, P. N. (2016). Two-step bioleaching of copper and gold from discarded printed circuit boards (PCB). Waste Management, 57, 149–157. https://doi.org/10.1016/j.wasman.2015.11.033
- Jacob, J. J., Revathy, T., Jayasri, M. A., & Suthindhiran, K. (2016). Diversity of magnetospirillum sp. From the Southern Coast of India. Current Science, 00113891) 111, 1. https://doi.org/10.18520/cs/v111/i1/177-183 (1)
- Jhu, C. Y., Wang, Y. W., Shu, C. M., Chang, J. C., & Wu, H. C. (2011). Thermal explosion hazards on 18650 lithium-ion batteries with a VSP2 adiabatic calorimeter. Journal of Hazardous Materials, 192(1), 99–107. https://doi.org/10.1016/j.jhazmat.2011.04.097
- Keim, C. N., & Farina, M. (2005). Gold and silver trapping by uncultured magnetotactic cocci. Geomicrobiology Journal, 22(1–2), 55–63. https://doi.org/10.1080/01490450590922550
- Kim, M. J., Seo, J. Y., Choi, Y. S., & Kim, G. H. (2016). Bioleaching of spent Zn–mn or Ni–cd batteries by aspergillus species. Waste Manag, 51, 168–173. https://doi.org/10.1016/j.wasman.2015.11.001
- Kopp, R. E., & Kirschvink, J. L. (2008). The identification and biogeochemical interpretation of fossil magnetotactic bacteria. Earth Science Reviews, 86(1–4), 42–61. https://doi.org/10.1016/j.earscirev.2007.08.001
- Kuzuhara, S., Akimoto, Y., Shibata, K., Oguchi, M., & Terazono, A. (2018). Evaluation by year of the valuable/hazardous material content of lithium-ion secondary battery cells and other components of notebook computer battery packs. Jouranl of Material Cycles Waste Management, 20(1), 431–438. https://doi.org/10.1007/s10163-017-0600-x
- Liang, Y., Zhao, C. Z., Yuan, H., Chen, Y., Zhang, W., Huang, J. Q., Yu, D., Liu, Y., Titirici, M. M., Chueh, Y. L., Yu, H., & Zhang, Q. (2019). A review of rechargeable batteries for portable electronic devices. InfoMat, 1(1), 6–32. https://doi.org/10.1002/inf2.12000
- Martinez-Laserna, E., Gandiaga, I., Sarasketa-Zabala, E., Badeda, J., Stroe, D. I., Swierczynski, M., & Goikoetxea, A. (2018). Battery second life: Hype, hope or reality? A critical review of the state of the art. Renewable & Sustainable Energy Reviews, 93, 701–718. https://doi.org/10.1016/j.rser.2018.04.035
- Meshram, P., Pandey, B. D., & Mankhand, T. R. (2015). Hydrometallurgical processing of spent lithium ion batteries (LIBs) in the presence of a reducing agent with emphasis on kinetics of leaching. Chemical Engineering Journal, 281, 418–427. https://doi.org/10.1016/j.cej.2015.06.071
- Needhidasan, S., Samuel, M., & Chidambaram, R. (2014). Electronic waste–an emerging threat to the environment of urban India. Journal of Environmental Health Science and Engineering, 12(1), 36. https://doi.org/10.1186/2052-336X-12-36
- Nshizirungu, T., Agarwal, A., Jo, Y. T., Rana, M., Shin, D., & Park, J. H. (2020). Chlorinated polyvinyl chloride (CPVC) assisted leaching of lithium and cobalt from spent lithium-ion battery in subcritical water. Journal of Hazardous Materials, 393, 122367. https://doi.org/10.1016/j.jhazmat.2020.122367
- Pant, D., Joshi, D., Upreti, M. K., & Kotnala, R. K. (2012). Chemical and biological extraction of metals present in E waste: A hybrid technology. Waste Management, 32(5), 979–990. https://doi.org/10.1016/j.wasman.2011.12.002
- Paul, S., Shakya, A. K., & Ghosh, P. K. (2020). Bacterially-assisted recovery of cadmium and nickel as their metal sulfide nanoparticles from spent Ni–cd battery via hydrometallurgical route. Journal of Environmental Management, 261, 110113. https://doi.org/10.1016/j.jenvman.2020.110113
- Prozorov, T., Perez-Gonzalez, T., Valverde Tercedor, C., Jimenez-Lopez, C., Yebra-Rodriguez, A., Körnig, A., Faivre, D., Mallapragada, S. K., Howse, P. A., Bazylinski, D. A., & Prozorov, R. (2014). Manganese incorporation into the magnetosome magnetite: magnetic signature of doping. European Journal of Mineralogy, 26(4), 457–471. https://doi.org/10.1127/0935-1221/2014/0026-2388
- Rahangdale, D., & Kumar, A. (2018). Acrylamide grafted chitosan based ion imprinted polymer for the recovery of cadmium from nickel-cadmium battery waste. Journal of Environmental Chemical Engineering, 6(2), 1828–1839. https://doi.org/10.1016/j.jece.2018.02.027
- Rahman, Z., & Singh, V. P. (2019). The relative impact of toxic heavy metals (THMs)(arsenic (as), cadmium (cd), chromium (Cr)(vi), mercury (hg), and lead (pb)) on the total environment: An overview. Environment Monitoring Assess, 191(7), 419. https://doi.org/10.1007/s10661-019-7528-7
- Randhawa, N. S., Gharami, K., & Kumar, M. (2016). Leaching kinetics of spent nickel–cadmium battery in sulphuric acid. Hydrometallurgy, 165, 191–198. https://doi.org/10.1016/j.hydromet.2015.09.011
- Recknagel, S., Radant, H., & Kohlmeyer, R. (2014). Survey of mercury, cadmium and lead content of household batteries. Waste Management, 34(1), 156–161. https://doi.org/10.1016/j.wasman.2013.09.024
- Richa, K., Babbitt, C. W., & Gaustad, G. (2017). Eco‐efficiency analysis of a lithium‐ion battery waste hierarchy inspired by circular economy. Journal of Industrial Ecology, 21(3), 715–730. https://doi.org/10.1111/jiec.12607
- Roy, J. J., Madhavi, S., & Cao, B. (2021). Metal extraction from spent lithium-ion batteries (LIBs) at high pulp density by environmentally friendly bioleaching process. Journal of Cleaner Production, 280, 124242. https://doi.org/10.1016/j.jclepro.2020.124242
- Rudnik, E., & Knapczyk-Korczak, J. (2019). Preliminary investigations on hydrometallurgical treatment of spent Li-ion batteries. Metallurgical Research and Technology, 116(6), 603. https://doi.org/10.1051/metal/2019008
- Sakunai, T., Ito, L., & Tokai, A. (2021). Environmental impact assessment on production and material supply stages of lithium-ion batteries with increasing demands for electric vehicles. Journal of Material Cycles & Waste Management, 23(2), 470–479. https://doi.org/10.1007/s10163-020-01166-4
- Salgado, A. L., Veloso, A. M., Pereira, D. D., Gontijo, G. S., Salum, A., & Mansur, M. B. (2003). Recovery of zinc and manganese from spent alkaline batteries by liquid–liquid extraction with cyanex 272. Journal of Power Sources, 115(2), 367–373. https://doi.org/10.1016/S0378-7753(03)00025-9
- Sannigrahi, S., & Suthindhiran, K. (2019). Metal recovery from printed circuit boards by magnetotactic bacteria. Hydrometallurgy, 187, 113–124. https://doi.org/10.1016/j.hydromet.2019.05.007
- Schüler, D., & Frankel, R. B. (1999). Bacterial magnetosomes: Microbiology, biomineralization and biotechnological applications. Applied Microbiology and Biotechnology, 52(4), 464–473. https://doi.org/10.1007/s002530051547
- Schuler, D., Spring, S., & Bazylinski, D. A. (1999). Improved technique for the isolation of Magnetotactic Spirilla from a freshwater sediment and their phylogenetic characterization. Systematic and Applied Microbiology, 22(3), 466–471. https://doi.org/10.1016/S0723-2020(99)80056-3
- Shin, S. M., Kim, N. H., Sohn, J. S., Yang, D. H., & Kim, Y. H. (2005). Development of a metal recovery process from Li-ion battery wastes. Hydrometallurgy, 79(3–4), 172–181. https://doi.org/10.1016/j.hydromet.2005.06.004
- Song, H. P., Li, X. G., Sun, J. S., Xu, S. M., & Han, X. (2008). Application of a magnetotactic bacterium, Stenotrophomonas sp. to the removal of Au (III) from contaminated wastewater with a magnetic separator. Chemosphere, 72(4), 616–621. https://doi.org/10.1016/j.chemosphere.2008.02.064
- Tajer-Mohammad Ghazvini, P., Kasra-Kermanshahi, R., Nozad Golikand, A., Sadeghizadeh, M., Ghorbanzadeh-Mashkani, S., & Dabbagh, R. (2016). Cobalt separation by Alphaproteobacterium MTB-KTN90: Magnetotactic bacteria in bioremediation. Bioprocess and Biosystems Engineering, 39(12), 1899–1911. https://doi.org/10.1007/s00449-016-1664-z
- Tanong, K., Tran, L. H., Mercier, G., & Blais, J. F. (2017). Recovery of Zn (II), Mn (II), Cd (II) and Ni (II) from the unsorted spent batteries using solvent extraction, electrodeposition and precipitation methods. Journal of Cleaner Production, 148, 233–244. https://doi.org/10.1016/j.jclepro.2017.01.158
- Teimouri, F., Mokhtari, M., Nasiri, T., & Abouee, E. (2023). Introducing heterotrophic iron ore bacteria as new candidates in promoting the recovery of e-waste strategic metals. World Journal of Microbiology & Biotechnology, 39(5), 137. https://doi.org/10.1007/s11274-023-03589-1
- Wang, Y., Gao, H., Sun, J., Li, J., Su, Y., Ji, Y., & Gong, C. (2011). Selective reinforced competitive biosorption of Ag (I) and Cu (II) on magnetospirillum gryphiswaldense. Desalination, 270(1–3), 258–263. https://doi.org/10.1016/j.desal.2010.11.053
- Wang, X., Li, Y., Zhao, J., Yao, H., Chu, S., Song, Z., He, Z., & Zhang, W. (2020). Magnetotactic bacteria: Characteristics and environmental applications. Frontiers of Environmental Science & Engineering, 14(4), 1–14. https://doi.org/10.1007/s11783-020-1235-z
- Watling, H. R. (2008). The bioleaching of nickel-copper sulfides. Hydrometallurgy, 91(1–4), 70–88. https://doi.org/10.1016/j.hydromet.2007.11.012
- Weshahy, A. R., Sakr, A. K., Gouda, A. A., Atia, B. M., Somaily, H. H., Hanfi, M. Y., Sayyed, M. I., El Sheikh, R., El-Sheikh, E. M., Radwan, H. A., & Cheira, M. F. (2022). Selective recovery of cadmium, cobalt, and nickel from spent Ni–cd batteries using Adogen® 464 and mesoporous silica derivatives. International Journal of Molecular Sciences, 23(15), 8677. https://doi.org/10.3390/ijms23158677
- Winslow, K. M., Laux, S. J., & Townsend, T. G. (2018). A review on the growing concern and potential management strategies of waste lithium-ion batteries. Resources, Conservation and Recycling, 129, 263–277. https://doi.org/10.1016/j.resconrec.2017.11.001
- Wu, W., Liu, X., Zhang, X., Li, X., Qiu, Y., Zhu, M., & Tan, W. (2019). Mechanism underlying the bioleaching process of LiCoO2 by sulfur-oxidizing and iron-oxidizing bacteria. Journal of Bioscience and Bioengineering, 128(3), 344–354. https://doi.org/10.1016/j.jbiosc.2019.03.007
- Xin, B., Jiang, W., Li, X., Zhang, K., Liu, C., Wang, R., & Wang, Y. (2012). Analysis of reasons for decline of bioleaching efficiency of spent Zn–mn batteries at high pulp densities and exploration measure for improving performance. Bioresource Technology, 112, 186–192. https://doi.org/10.1016/j.biortech.2012.02.133
- Zhou, W., Zhang, Y., Ding, X., Liu, Y., Shen, F., Zhang, X., Deng, S., Xiao, H., Yang, G., & Peng, H. (2012). Magnetotactic bacteria: Promising biosorbents for heavy metals. Applied Microbiology and Biotechnology, 95(5), 1097–1104. https://doi.org/10.1007/s00253-012-4245-3
- Zubi, G., Dufo-López, R., Carvalho, M., & Pasaoglu, G. (2018). The lithium-ion battery: State of the art and future perspectives. Renewable and Sustainable Energy Reviews, 89, 292–308. https://doi.org/10.1016/j.rser.2018.03.002