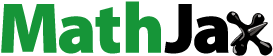
ABSTRACT
Tunnels with high-temperature rocks are built in seismically active areas, which may suffer heavy damages in earthquakes. This paper aims to explore the thermal insulation effect and seismic isolation effect layer of the thermal insulation layer in high-temperature tunnels. Firstly, taking Jiwoxiga tunnel in Tibet, China as the research background, the finite difference models are established to investigate temperature fields, seismic response, and structural safety of the tunnel. Then, the two most commonly used insulation layers are applied to the support structure to study the thermal insulation effect and seismic isolation effect of the layers. Besides, the effect of factors including the thickness, elastic modulus, surrounding rock, and boundary temperature on the application of the layer are investigated in detail. The result shows that the application of the thermal insulation layer has isolated the geothermal and seismic motion propagating from the rock to linings, thus improving the structural safety of the high-temperature tunnel when encountering strong earthquakes. The thermal insulation effect of the layer in the high-temperature tunnel enhances with the increasing thickness of the layer, and its seismic isolation behaviour enhances with the increasing of the layer thickness and elastic modulus.
1. Introduction
While people enjoy the convenience brought by traffic tunnels, we cannot ignore the tunnel damage brought by high-temperature rocks. A tunnel with a surrounding rock temperature higher than 30°C can be considered as a high rock temperature tunnel. Typical high rock temperature tunnels have been built in some countries, including Anfang tunnel in Japan, Jiuzhai railway tunnel, and Jiwoxiga tunnel in China, with the highest rock temperature of 75°C, 52°C, and 58°C respectively. Tunnels with high rock temperature often appear in tunnels buried more than 1000 m deep, tunnels passing through geothermal active areas, or tunnels passing through magmatic areas(Zhou et al., Citation2021). Previous studies have shown that the temperature of surrounding rock increases with the increase of tunnel depth, especially when the tunnel depth exceeds 1500 m, the temperature will increase rapidly with the increase of tunnel depth. For the tunnel with high rock temperature, too high temperature during construction will slow down the construction speed, affect the normal construction period, and even cause heatstroke of workers(Liu et al., Citation2019).
The main factors leading to the earthquake damage of tunnels in strong earthquake areas are seismic inertia force and forced displacement (Cui & Ma, Citation2021a). In the tunnel with high rock temperature, when the lining is subjected to high temperature, it will produce additional temperature stress, which will lead to pores, micro-cracks, and macro cracks in the construction of tunnel lining concrete(Haido et al., Citation2021; Thomas et al., Citation2021). On this basis, under the action of a strong earthquake load, micro-cracks will continue to gather and connect, resulting in new macro cracks. Macro cracks will gradually expand along the weakest path and finally lead to concrete damage(Abdel-Motaal et al., Citation2014; Wang et al., Citation2019). Therefore, the tunnel structure is affected by high rock temperature, brittle degradation, plastic enhancement, coupled with seismic action, the stability of surrounding rock is greatly affected, and the risk of instability increases sharply. The research of high rock temperature tunnels mainly includes heat damage classification, ventilation and cooling, support structure strengthening, material characteristics of high temperature-resistant materials, and so on(Liu et al., Citation2019; Wang et al., Citation2019). However, the literature on the seismic mitigation technology of high rock temperature tunnels under strong earthquakes is very rare. Progress has been made in the last few years in understanding the lining–soil interaction mechanism, and the earthquake motion transfer from ground to lining structure during the earthquake, while the dynamic response of tunnel under the action of high rock temperature and strong earthquake is rarely reported in literatures.
In theory, two approaches can be used to reduce the damage of high-temperature tunnels subjected to the earthquake (Tsinidis et al., Citation2020; Zhao et al., Citation2018). One is the design of thermal insulation and shock absorption, which is to reduce the energy when an earthquake occurs through the thermal insulation layer, and prevent the high temperature of surrounding rock from transferring to the secondary lining structure (Kang et al., Citation2020; Suzuki et al.). The second is the reinforcement of structure and surrounding rock, which is to reduce the tunnel damage of thermal seismic coupling by appropriately improving the stiffness, toughness, and crack resistance of lining and surrounding rock (Cui & Ma, Citation2021b; Jamshidi Avanaki, Citation2019). At present, there are few researches on the control technology of tunnel failure under thermal seismic interaction and mainly focuses on the structure reinforcement and the design of thermal insulation efficiency under a single factor of temperature or earthquake(Bilotta, Citation2018; Corigliano et al., Citation2011). This paper provides results of 3D thermal–seismic interaction models, conducted in FLAC 5.0 (Inc, Citation2015), for high-temperature tunnels subjected to the strong seismic motion, produced by the seismic waves of the 2008 Wenchuan earthquake. Firstly, taking Jiwoxiga tunnel in Tibet, China as the research background, the finite difference models are established to investigate temperature fields, seismic response, and structural safety of the tunnel. Then, the two most commonly used insulation layers are applied to the support structure to study the thermal insulation effect and seismic isolation effect of the layers. Besides, the effect of factors including the thickness, elastic modulus, surrounding rock, and boundary temperature on the application of the layer are investigated in detail. The result may provide good guidance for the same engineering.
2. Materials and methods
2.1. Geological condition
The Jiwoxiga tunnel of the Lasa–Rikaze Railway is located on the left bank of Yarlung Zangbo River in Nimu County, Tibet Autonomous Region. The geological conditions of the Jiwoxiga tunnel are severely complex, and there are many dangerous geological areas, including the debris flow, rockfall, rock heap, geothermal, and so on. Referring to the geological data, the lithology of the tunnel body is grade V and Ⅳ rocks(China RTSotPsRo, Citation2017). The support structure of the Jiwoxiga tunnel in the high rock temperature section adopts composite lining (see ) with a span of 12.40 m and a height of 9.28 m. C25 shotcrete with a thickness of 25 cm is used for primary support, and C25 plain concrete with a thickness of 45 cm is used for secondary lining(MOHURD, Citation2011). In addition, geothermal is the most typical geological condition of the tunnel. It is found that the ground temperature at the tunnel entrance is 65°C, and the highest ground temperature in the actual tunnel construction is about 55°C. The geological structure-activity is intense, and the geothermal is relatively developed. Moreover, the Jiwoxiga tunnel is located in the Yarlung Zangbo gorge Valley, which is located in the Himalayan seismic belt. The tunnel site has high seismic intensity, so the probability of an earthquake is high.
2.2. Thermal insulation layer
In this paper, by conducting the numerical analysis, the thermal isolation effect and seismic response of the thermal insulation layer are studied under the coupling effect of the seismic motion and the high geothermal. Besides, the numerous influence factors on the seismic response and the insulation effect were conducted, including the layer materials, elastic modulus, rock lithologies, boundary conditions.
2.3. Basic assumption
The following assumptions are adopted to explore the application of thermal insulation layer in high-temperature tunnels:
(1) The materials of the surrounding rock are homogenous and isotropic, and its thermal conductivity is constant, and the influence of temperature on the thermal conductivity is not considered.
(2) The thermal insulation layer and tunnel lining are homogeneous and isotropic materials, and their interface can transfer force and heat by specifying the thermal and stress parameters.
2.4. Numerical model
Taking the Jiwoxiga tunnel as the research background, the finite difference calculation models are set up to study the seismic and thermal behaviours of the high-temperature tunnel, as is shown in . The buried depth and the longitudinal depth of the tunnel are 85 m and 35 m, respectively. The width of both sides of the tunnel is 5 times that of the tunnel, about 50 m, to minimise the influence of the boundary on the calculation results. Due to the limitation of FLAC software in modelling, ANSYS APDL was used to establish the finite element model, and then the model was imported into FLAC 5.0 for dynamic and thermal calculation.
The numerical simulation including two steps: (1) the geostatic calculation and (2) the dynamic analysis. In the geostatic calculation, the normal displacements of the four sides and the bottom of the constrained model, the boundary temperatures are applied to the model. The temperatures of the upper surface, lower surface, and lining surface are set as 30°C, 80°C, and 28°C, respectively. Before the seismic simulation, the displacement data in the model is cleared to simulate the actual response of the original tunnel to ground stress. Then, the dynamic motion was input to study the isolation effect and thermal insulation of the layers. In the present study, the time history of acceleration recorded by Wolong station in the 2008 Wenchuan earthquake is chosen as the input motion, since this particular load could reflect the real seismic response characteristics of the Jiwoxiga tunnel site area. Considering the calculation time and accuracy of numerical simulation, the original seismic wave is filtered and the baseline is corrected. The duration of the modified seismic acceleration wave is 30 s, the acquisition interval is 0.01 s, and it has 95% of the energy of the original wave, as is shown in . In the dynamic calculation, the free-field boundary in FLAC software is used, which can effectively reduce the reflection of seismic waves at the model boundary. The east-west, north-south and vertical directions of the waves are corresponding to the longitudinal, transverse, and vertical direction of the tunnel in the numerical model. The mechanical damping used in the dynamic calculation is local damping, with a coefficient of 0.1571. The thermal, stress, and internal force data of the model are collected by using the history command of the software. In this study, totally eight measuring points (see ) are set on the circumferential direction of lining, namely the vault, left spandrel, left wall, left-wall foot, right spandrel, right wall, right-wall foot and invert.
2.5. Test cases
Based on the previous investigation of the layer, the isolation is set between the surrounding rock and primary support, with a thickness of 10 cm (Cui & Ma, Citation2021a). Firstly, the silicate composite coil and rigid polyurethane board are chosen as the materials of the insulation layer to find the optimum layer materials (see ). The silicate insulation composite felt has good compression performance and can be used at a high temperature of 600°C, but its thermal conductivity is higher than that of general insulation materials; however, rigid polyurethane board has the lower thermal conductivity but poor compression performance, and can only be used below 120°C. Then, referring to the related Chinese Code, the surrounding rocks with the grade of V, IV, III, and the boundary temperature of 60°C, 70°C, 80°C, 90°C were adopted to study the influence of the rock parameters. Finally, the layer with the thickness of 10 cm, 20 cm, 30 cm, and with the elastic modulus of 60 MPa, 120 MPa, 240 MPa are used to study the influence of the parameters of the layer. In this paper, the mechanical and thermal parameters are measured by laboratory tests, and see and for details.
Table 1. Physical parameters
Table 2. Thermal parameters
3. Results and analysis
3.1. Heat insulation effect
The thermal data of tunnel linings are collected by the history command, and the peak temperature is extracted in .
In general, the temperature distribution of the secondary lining under 13 cases is similar, and there are maximum temperatures at the left and right arch feet. The application of isolation layers could decrease the temperature, and the insulation effect of rigid polyurethane board is better than that of silicate insulation composite roll felt. In the present study, the thermal insulation effect of the insulation layer is calculated by EquationEquation (1)(1)
(1) .
Where represents the insulation capacity of the isolation capacity,
represents the lining’s temperature of tunnel with isolation layer,
represents the lining’s temperature of the original tunnels.
In , the insulation capacity of the silicate composite roll felt is 4.129%–4.669%, and that of rigid polyurethane board is 5.037%–6.282%. The thermal isolation effect of rigid polyurethane board is better than the silicate insulation composite roll felt. plots the temperature data of the rigid polyurethane board with different surrounding rock grades. The insulation effect of the layer in the grade of V, IV, III is 5.04%–6.08%, 5.36%–6.65%, and 5.74%–7.30%, respectively. Referring to the Chinese Code, the higher the grade of surrounding rock is, the worse its lithology is. Thus, the thermal insulation effect of the layer in weak rocks is better than that in hard rocks.
In theory, the insulation effect of the rigid polyurethane board enhances as its thickness and boundary temperature increase, the results of and have well corresponded to this law. It can be observed that the thermal insulation layer with a thickness of 30 mm has the best insulation effect, followed by the 20 mm-thick layer and the 20 mm-thick layer. With respect to the boundary, the lining’s temperature increases as the boundary temperature increases, the insulation effect of the rigid polyurethane board under the boundary temperature of 90°C and 60°C is 2.69%–3.56% and 6.72%–7.83%, respectively.
3.2. Seismic isolation effect
3.2.1. Principal stress response
The time history of the maximum principal stress of tunnel vault is shown in , taking the tunnel without any layers as an example for illustrating. The peak value of the maximum principal stress of tunnel vault is depicted in . Overall, the peak stress of tunnel lining is almost similar, and it all reaches the maximum at the inverted arch, the peak stresses of the tunnel lining with insulation layers have been decreased with respect to the original tunnel. This shows that the application of the thermal insulation layer can prevent the seismic force from spreading to the lining structure and reduce the stress of the lining structure. As depicted in , the stress isolation effect of silicate composite roll felt is slightly better than that of rigid polyurethane board.
The peak value of the maximum principal stress of the insulation layer with different thicknesses is plotted in . The maximum principal stress of the tunnel lining decreases by 6.41%–12.67%, 9.38%–21.66%, and 10.66%–22.43% with the layer thickness of 10 cm, 20 cm, and 30 cm, respectively. It can be observed that the thicker the insulation, the better the barrier effect. This law has well corresponded to the shock absorption theory. However, the thicker layer not increases the difficulty of construction but costs much money. In the actual tunnel, the thickness of the thermal insulation layer should be determined after considering the construction technology, effectiveness of layers, and construction funds.
4. Axis force and bending moment response
depicts the time history of the axis force and the bending moment of the tunnel vault (taking the original tunnel as an example for illustrating). It can be seen from Fig X that the axial force of the original tunnel presents a compressive stress state, and the maximum value appears at the left spandrel of the tunnel, with a value of – 3758kN.
The axial force distribution of section in cases with the thermal insulation layer is similar (), the axial force of vault and invert shows tensile stress state, and the other measuring points show compressive stress state; the maximum value appears at the left spandrel of tunnel, in which the value of case 2 is – 3520kN, and the value of test case 3 is – 3554kN. To sum up, the axial force of the tunnel structure after the construction of the insulation layer shows a downward trend.
From , the bending moment of the section in case 1 is under lateral tension, and the maximum value appears at the left and right spandrels of the tunnel, with a value of 30.8kN•m. While the bending moment of the section in cases 2 and 3 is under lateral tension except for the invert; the maximum bending moment of case 2 appears at the right spandrel of the tunnel, with a value of 11.0kN•m, and the maximum bending moment of case 3 appears at the vault, with a value of 9.2kN•m.
4.0.2. Safety factor response
The safety factor in the Chinese code(China RTSotPsRo, Citation2017) is used to measure the safety of tunnel structure, which is calculated by EquationEquation (2)(2)
(2) -(Equation3
(3)
(3) ). The time history of the safety factor is shown in , taking the vault point of the original tunnel as an example for illustrating. Fig X-Y presents the maximum safety factor over the measuring points. In general, the minimum safety factor of test cases all appears at the left spandrel of the tunnel, the minimum value of the original tunnel is the smallest. From , it can be concluded that the safety of the original tunnel without measures is the lowest, that of silicate composite roll felt is the best, and that of rigid polyurethane plate is between them.
In this paper, the seismic isolation effect of thermal insulation layers could be calculated by EquationEquation (4)(4)
(4) , that is, the growth percentage of the safety factor of the tunnel with the thermal insulation layer compared with that of the original tunnel.
Where is safety factor;
is longitudinal-bending coefficient;
is influence coefficient of axial force eccentricity;
is ultimate compressive strength of concrete; b is width of tunnel section; h is thickness of section;
is ultimate tensile strength of concrete;
is eccentricity of section.
Where is the isolation effect;
is the safety factor of original and
is the safety factor of tunnel with thermal insulation layer.
The seismic isolation effects of the tunnel with the silicate composite roll felt and the tunnel with rigid polyurethane board are 3.56%–111.42% and 2.51%–101.35%. The results show that the isolation effect of vault and invert is obvious, while that of other measuring points is limited. The isolation effect of silicate composite roll felt is better than that of the rigid polyurethane board.
plot the minimum safety of the tunnel with the rigid polyurethane board under different surrounding rock grades, layer thickness, and elastic modulus. The safety factor decreases with the increasing rock grades. In , the seismic isolation effect of the 10 cm-, 20 cm-, and 30 cm-thick rigid polyurethane all increases, and it enhances with the thickness of the layer. Besides, the safety factor increases as the elastic modulus of the layer increases, the layer with the elastic modulus of 240 MPa has the highest safety factor.
To sum up, the seismic isolation effect of the thermal insulation increases with the increasing of the layer thickness and the layer’s elastic modulus. It should be noticed that the thicker layer and high elastic modulus materials may extremely enhance the construction difficulty and increase engineering cost. In the actual engineering, the application of the thermal insulation layer should not only consider its effectiveness but also the construction technology, as well as the engineering cost.
5. Discussion and conclusion
In view of the fact that tunnels with high-temperature rock are widely constructed in strong earthquake areas, taking Jiwoxiga tunnel in Tibet, China as the research background, the finite difference models are established to investigate temperature fields, seismic response, and structural safety of the tunnel. Then, the two most commonly used insulation layers are applied to the support structure to study the thermal insulation effect and seismic isolation effect of the layers. Besides, the effect of factors including the thickness, elastic modulus, surrounding rock, and boundary temperature on the application of the layer are investigated in detail. Some important conclusions are obtained as follows:
(1) The application of the thermal insulation layer has isolated the geothermal and seismic motion propagating from the rock to the tunnel lining, thus the structural safety of the high-temperature tunnel improves when encountering strong earthquakes.
(2) The thermal insulation effect of the layer in the high-temperature tunnel enhances with the increasing thickness of the layer, and its seismic isolation behaviour enhances with the increasing of the layer thickness and elastic modulus.
(3) The safety factor of the high-temperature tunnels increases after the thermal insulation layer is adopted in the support structure, and the seismic safety performance of the tunnel structure has been effectively improved.
Acknowledgments
We are very grateful to the experts of the same research group who put forward valuable opinions in the research process of this paper, and the graduate students of the same research group who helped us proofread the article in the process of writing the article.
Data Availability Statement
The authors confirm that the data supporting the findings of this study are available within the article and its supplementary materials.
Disclosure statement
The authors declared that they have no conflicts of interest to this work.
Additional information
Funding
References
- Abdel-Motaal, M. A., El-Nahhas, F. M., & Khiry, A. T. (2014). Mutual seismic interaction between tunnels and the surrounding granular soil. HBRC Journal, 10, 265–278. https://doi.org/https://doi.org/10.1016/j.hbrcj.2013.12.006
- Bilotta, E. (2018). Modelling tunnel behaviour under seismic actions: An integrated approach (pp. 3–20). CRC Press. https://doi.org/https://doi.org/10.1201/9780429438660-1
- China RTSotPsRo. (2017). Code for design of railway tunnel: TB 10003—2016. China Railway Publishing House.
- Corigliano, M., Scandella, L., Lai, C. G., & Paolucci, R. (2011). Seismic analysis of deep tunnels in near fault conditions: A case study in. Southern Italy Bulletin of Earthquake Engineering, 9(4), 975–995. https://doi.org/https://doi.org/10.1007/s10518-011-9249-3
- Cui, G., & Ma, J. (2021a). Combination of lining strengthening and buffer layers for soft and hard rock tunnels junction subjected to seismic waves. Geomatics, Natural Hazards and Risk, 12, 522–539. https://doi.org/https://doi.org/10.1080/19475705.2021.1886184
- Cui, G., & Ma, J. (2021b). Structure Strengthening Method for Enhancing Seismic Behavior of Soft Tunnel Portal Section. Mathematical Problems in Engineering, 1–12. 2021. https://doi.org/https://doi.org/10.1155/2021/6624963
- Haido, J. H., Tayeh, B. A., Majeed, S. S., & Karpuzcu, M. (2021). Effect of high temperature on the mechanical properties of basalt fibre self-compacting concrete as an overlay material. Construction and Building Materials, 268, 121725. https://doi.org/https://doi.org/10.1016/j.conbuildmat.2020.121725
- Inc, I. C. G. (2015). FLAC3D-Fast Lagrangian Analysis of Continua in 3 Dimension. Ver. 5.0 User’s Manual.
- Jamshidi Avanaki, M. (2019). Response modification factors for seismic design of steel Fiber Reinforced Concrete segmental tunnels. Construction and Building Materials, 211, 1042–1049. https://doi.org/https://doi.org/10.1016/j.conbuildmat.2019.03.275
- Kang, F., Li, Y., & Tang, C. (2020). Numerical study on airflow temperature field in a high-temperature tunnel with insulation layer. Applied Thermal Engineering, 179, 115654. https://doi.org/https://doi.org/10.1016/j.applthermaleng.2020.115654
- Liu, P., Cui, S., Li, Z., Xu, X., & Guo, C. (2019). Influence of surrounding rock temperature on mechanical property and pore structure of concrete for shotcrete use in a hot-dry environment of high-temperature geothermal tunnel. Construction and Building Materials, 207, 329–337. https://doi.org/https://doi.org/10.1016/j.conbuildmat.2019.02.125
- MOHURD. (2011). Code for design of concrete structures. China Construction Industry Press.
- Thomas, C., Tamayo, P., Setién, J., Ferreño, D., Polanco, J. A., & Rico, J. (2021). Effect of high temperature and accelerated aging in high density micro-concrete. Construction and Building Materials, 272, 121920. https://doi.org/https://doi.org/10.1016/j.conbuildmat.2020.121920
- Tsinidis, Grigorios, Filomena de Silva, Ioannis Anastasopoulos, Emilio Bilotta, Antonio Bobet, Youssef M. A. Hashash, Chuan He, Georgios Kampas, Jonathan Knappett, Gopal Madabhushi, Nikolaos Nikitas, Kyriazis Pitilakis, Francesco Silvestri, Giulia Viggiani, and Raul Fuentes. (2020) Seismic behaviour of tunnels: From experiments to analysis. Tunnelling and Underground Space Technology, 99:103334 https://doi.org/https://doi.org/10.1016/j.tust.2020.103334
- Wang, M., Hu, Y., Wang, Q., Tian, H., & Liu, D. (2019). A study on strength characteristics of concrete under variable temperature curing conditions in ultra-high geothermal tunnels. Construction and Building Materials, 229, 116989. https://doi.org/https://doi.org/10.1016/j.conbuildmat.2019.116989
- Zhao, W., Chen, W., & Yang, D. (2018). Interaction between strengthening and isolation layers for tunnels in rock subjected to SH waves. Tunnelling and Underground Space Technology, 79, 121–133. https://doi.org/https://doi.org/10.1016/j.tust.2018.05.012
- Zhou, X., Ren, X., Ye, X., Tao, L., Zeng, Y., & Liu, X. (2021). Temperature field and anti-freezing system for cold-region tunnels through rock with high geotemperatures. Tunnelling and Underground Space Technology, 111, 103843. https://doi.org/https://doi.org/10.1016/j.tust.2021.103843