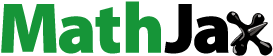
ABSTRACT
In this paper, the electronic, magnetic, and thermodynamic properties of NO, NO2, N2O adsorption by using C-doped boron nitride nanocage have been investigated using density functional theory. Based on nuclear quadrupole resonance (NQR) analysis, carbon-doped on B5N10 has shown the lowest fluctuation in electric potential and the highest negative atomic charge including 0.1190, 0.1844, and 0.1312 coulomb in NO→C–B4N10, NO2→C–B4N10, and N2O→C–B4N10, respectively. The C-doped complexes can be an appropriate option with the highest tendency for electron acceptance in the adsorption process. The reported results of nuclear magnetic resonance (NMR) spectroscopy have exhibited that atom doping of C on the B4N10 surface increases NO, NO2, N2O adsorption. The observed increase in the chemical shift anisotropy spans for N atoms, O(2) for NO/N2O adsorption and O(2)/O(18) for NO2 adsorption on the C–B4N10. Finally, Gibbs free energy alteration has shown that for a given number of nitrogen donor sites in NO, NO2, N2O, the stabilities of complexes owing to doping atom of C can be considered as: NO2→C–B4N10 > N2O→C–B4N10 > NO→C–B4N10. The procedure used in this research can be applied to the characterization of gaseous pollutants in any adsorption process.
1. Introduction
To address the most considerable environmental challenges, a probe for high-performance gas sensing materials is essential. Gas adsorption induces changes in the material’s work function, suggesting the potential application of these materials as catalysts. The development in micro and nano technologies enabled fabrication of structures whose function depends on adsorption-desorption processes. That dependence can be favourable like in affinity-based chemical and biochemicals sensors including graphene-based gas sensors, or undesirable like in microresonators whose adsorption-induced mass fluctuations provoke frequency instabilities. Although the process itself is not new, corresponding models are still under development. A requirement for models is that they need to be fast, reliable and flexible, suitable for stand-alone use or incorporation in multiscale models of complex systems [Citation1–6].
Furthermore, modelling of adsorption capacities and parameters for equations of adsorption kinetics and dynamics, the employment of artificial neural networks is used to explore the effect of surface structure on the adsorption process. In a combination of molecular simulation and machine learning was used to examine the role of various pore topological structures on the CO2 capture capabilities of metal organic frameworks [Citation7]. Boron nitride nanomaterials have been used owing to their unparalleled specifications of eco-friendly attributes for pollutant adsorption and semiconducting property [Citation8–11]. Boron nitride nanomaterials usually exhibit semi-leading behaviour, which is considered a proper alternative to carbon nanotubes. The properties of boron and nitrogen atoms which are the first neighbours of carbon in the periodic table, make boron nitride an interesting subject of numerous studies [Citation12–14]. In recent years, different investigations on the adsorption of chemical contaminants and applying various boron nitride nanostructures as adsorbents for water purification have been studied [Citation15–17].
Various physical shapes of boron nitride (BN)-based nano adsorbents such as nanoparticles, fullerenes, nanotubes, nanofibers, nanoribbons, nanosheets, nanomeshes, nanoflowers, and hollow spheres have been broadly considered possible adsorbents owing to their exceptional characteristics such as large surface area, structural variability, great chemical/mechanical strength, abundant structural defects, high reactive sites, and functional groups [Citation18,Citation19].
Adsorption involving charged adsorbates causes a change in the double layer and the potential at the outer Helmholtz plane, influencing the adsorption rates of both anodic and cathodic reactions. The first three modes are intimately with adsorption and the double layer involves interaction of the adsorbates and the intermediate products. These compounds have been formed during the partial electrochemical reactions and interaction of the adsorbed intermediates with organic molecules which can either inhibit or enhance electrode reaction rate.
Among all porous materials with potential applications for gas adsorption, boron nitride (BN), with its outstanding thermal and chemical stability, might be of great interest. Particularly, this research work aims to assess the influences of doping atom of C on the B4N10 for increasing gas detection through measurement of some parameters containing charge transfer, electric potential, electromagnetic and thermodynamic properties, functional group and examine the removal of selected toxic gas molecules including NO, NO2 and N2O during adsorption process.
2. Theoretical insights, applied material and method
2.1. Adsorption of gas molecules on C–B4N10
The aim of this study is to remove toxic gas molecules including NO, NO2, N2O from air through an eco-friendly approach by using C-doped B4N10 (). B5N10 nanosensor was modelled in the presence of doping atoms of carbon which can increase the gas sensing potential of the nanosurface. In our research, sample characterization was performed by CAM – B3LYP – D3/EPR–3, LANL2DZ level of theory.
Figure 1. Application of C–B4N10 towards adsorption of gas molecules of NO, NO2, N2O and formation of complexes: NO→C–B4N10, NO2→C–B4N10, and N2O→C–B4N10, complexes using CAM–B3LYP–D3/6–311+G (d,p), LANL2DZ calculation.
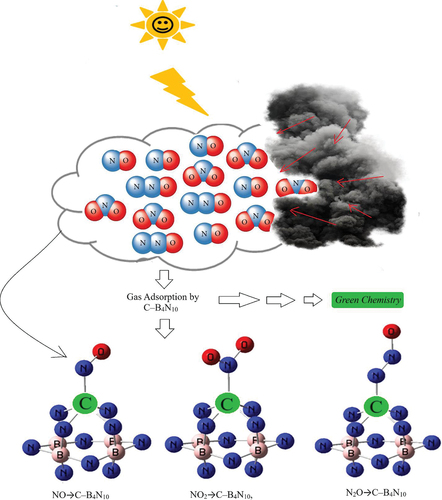
has shown the process adsorption of gas molecules of NO, NO2, N2O on the C – B4N10 surface which towards formation of complexes containing NO→C – B4N10, NO2→C – B4N10 and N2O→C – B4N10 by molecular modelling computations. The charge distribution of mentioned complexes is calculated due to the Bader charge analysis [Citation20].
The trapping of NO, NO2 and N2O molecules by C – B4N10 was successfully incorporated due to binding formation consisting of N→C (). Regardless of which gas molecule, the boron nitride nanocage expanded to accommodate the gas molecules by enhancing the selective sensitivity through different doping atoms of C ().
2.2. Application of density functional theory (DFT) approach
Hohenberg-Kohn (HK) functions have rigidly made the electronic density permissible as fundamental variable to electronic and structure computations. In other words, development of the applied DFT methodology only became notable after W. Kohn and L. J. Sham released their reputable series of equations which are introduced as Kohn-Sham (KS) equations [Citation21–26].
Considering the electronic density within the KS image directs us to a remarkable reduction of quantum computing. Thus, the KS methodology lightens the route for pursuing systems that cannot be discussed by conventional ab-initio methodologies. Kohn and Sham introduces the solution which brings up the mono-electronic orbitals to account the kinetic energy in a simple and relatively exact, by finding a residual modification that might be computed apart. So, one starts with a reference model of M with non-interacting electrons related to the external potential and with Hamiltonian [Citation25,Citation26]:
By representing the single particle orbitals all electronic densities physically acceptable for the system of ‘non-interacting’ electrons are written in the EquationEquation 2
(2)
(2) [Citation25,Citation26]:
Finally, the total energy could be measured by the KS method due to the EquationEquation 3(3)
(3) [Citation25,Citation26]:
Therefore, the precise exchange energy functional is described by the Kohn – Sham orbitals in lieu of the density which is cited as the indirect density functional. This research has employed the penetration of the hybrid functional of three-parameter basis set of B3LYP (Becke, Lee, Yang, Parr) within the conception of DFT upon theoretical computations [Citation27,Citation28]. The popular B3LYP (Becke, three-parameter, Lee – Yang – Parr) and exchange-correlation functional become based on EquationEquation 4(4)
(4) [Citation29–31]:
show a generalized gradient approximation: the Becke exchange functional [Citation32] and the correlation functional of Lee, Yang and Parr [Citation33] for B3LYP and
is the VWN local spin density approximation to the correlation functional [Citation34].
In this article, the rigid PES using DFT calculations has been computed applying Gaussian 16 revision C.01 software [Citation35]. The input Z-matrix for adsorption of NO, NO2 and N2O molecules in air by the C – B4N10 has been designed with GaussView 6.1 [Citation36] using 6–311+G (d,p), EPR–3, LANL2DZ basis set. In this study, the interaction between gas molecules and C – B4N10 was modelled and analysed. As revealed by DFT-based analysis, the potency of C – B4N10 for grabbing gas molecules was determined mainly by the electronegativity of the functional groups, as well as the interaction between the C – B4N10 and the gas molecules.
3. Results and discussion
Gas molecules that are dominant polluants in air are NO, NO2 and N2O. The data has evaluated the efficiency of boron nitride nanocage doped with carbon for gas detection. In fact, it can be remarked the chemisorptive nature of the bond among the gas molecules with boron and nitrogen elements through the equilibrium distribution of doping atom of C, B5N10, and a monolayer attribute.
3.1. PDOS & electronic evaluating
The electronic structures of gas adsorption (G) using C-doped B5N10 as the selective sensor for detecting and grabbing gas molecules in the air have been illustrated using CAM – B3LYP – D3/6–311+G (d,p), LANL2DZ level of theory.
shows the projected density of state (PDOS) of G→C – B4N10 through gas molecules adsorption. The appearance of the energy states (p-orbital) of C, N, O within the gap of C – B4N10 induces the reactivity of the system. It is clear from the figure that after trapping with gas molecules, there is a significant contribution of p-orbital in the unoccupied level. Therefore, the curve of partial PDOS has described that the p states of N atoms in gas molecules and C atom in C – B4N10 are overcoming due to the conduction band . A distinguished adsorption trait might be seen in G→C – B4N10 because of the potent interaction between the p states of nitrogen atom in gas molecules with p states of C in C – B4N10 complexes.
Figure 2. PDOS of gas molecules of NO, NO2, N2O adsorbed on C–B4N10 including a) NO→C–B4N10, b) NO2→C–B4N10, and c) N2O→C–B4N10 complexes by CAM–B3LYP–D3/6–311+G (d,p), LANL2DZ.
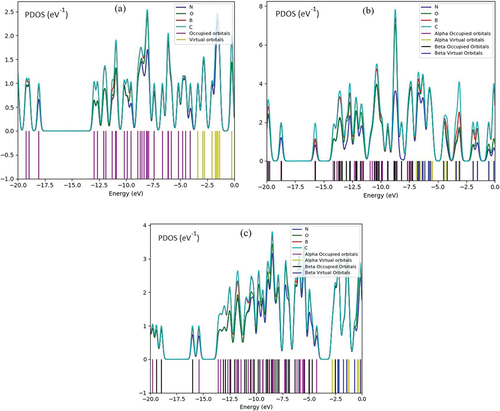
NO→C – B4N10 has indicated one sharp peak for C in around −7.5 eV. Furthermore, NO2→C – B4N10 has exhibited a strong peak around −9 eV through the graphs for C atom. Furthermore, the C graph with a sharp peak around −9 eV in N2O→C – B4N10 has been shown. Based on the PDOS of adsorbed gas molecules of NO, NO2 and N2O and the surface coverage, both in equilibrium, we can estimate the surface density of adsorption sites.
3.2. Insight to nuclear quadrupole resonance (NQR)
As the EFG at the citation of the nucleus in NO, NO2, N2O is allocated by the valence electrons twisted in the attachment with close nuclei of C – B4N10 through trapping of gas molecules, the NQR frequency at which transitions occur is particular for G→C – B4N10 complexes (G= NO, NO2, N2O) (). The NQR method is related to the multipole expansion in Cartesian coordinates as the Equationequation (5)(5)
(5) [Citation37,Citation38]:
Table 1. The electric potential (Ep/a.u.) and bader charge (Q/e) through NQR calculation for NO→C – B4N10, NO2→C – B4N10, and N2O→C – B4N10 complexes using CAM – B3LYP – D3/EPR–3, LANL2DZ calculation.
After that, a simplification on the equation (5), there are only the second derivatives related to the identical variable for the potential energy [Citation37,Citation38]:
There are two parameters which must be gotten from NQR experiments: the quadrupole coupling constant, , and asymmetry parameter of the EFG tensor
[Citation37,Citation38]:
where are ingredients of the EFG tensor at the quadrupole nucleus determined in the EFG principal axes system,
is the nuclear quadrupole moment,
is the proton charge and
is the Planck’s constant [Citation39,Citation40].
In this research work, the electric potential as the quantity of work energy through carrying over the electric charge from one position to another position in the essence of electric field has been evaluated for NO→C – B4N10, NO2→C – B4N10, and N2O→C – B4N10 complexes ().
In , the Bader charge and electronic potential properties of C – B, N in C – B4N10 and N, O of gas molecules trapped on doped-boron nitride nanocages have been investigated. The amounts indicate that with increasing the negative charge of different atoms, the electric potential resulted from NQR calculations increase. Moreover, the doping atom of C (15) on the B4N10 has shown the most potential for accepting the electron from electron donor of N (1) in NO, NO2, N2O adsorbed on the B5N10 ().
Furthermore, in , it has been sketched the electric potential of nuclear quadrupole resonance for some atoms of C/B, N in C – B4N10 and N, O of gas molecules trapped on doped-boron nitride nanocages which has been calculated by CAM – B3LYP – D3/EPR–3, LANL2DZ.
Figure 3. Electric potential (Ep/a.u.) versus bader charge (Q/e) through NQR calculation for a) NO→C–B4N10, b) NO2→C–B4N10, and c) N2O→C–B4N10 complexes by CAM–B3LYP–D3/EPR–3, LANL2DZ.
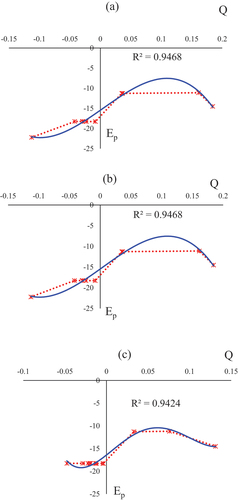
In , it was observed the behaviour of NO adsorption on the C – B4N10 with high sensitivity based on relation coefficient of R2 = 0.9468. Adsorption of NO2 on the C – B4N10 in has illustrated the same sensing as NO. In addition, has resulted that N2O→C – B4N10 has a good detecting for N2O removal from air through relation coefficient of R2 = 0.9424.
It’s vivid that the curve of C – B4N10 is waved by these gas molecules. The fluctuated peaks for electric potential have been shown around NO NO2≈N2O trapping on the C – B4N10 which demonstrate the electron accepting specifications of nitrogen and oxygen versus carbon doped on the B5N10 . Besides, it can be considered that silicon as a semiconductor element in the functionalized B5N10 might have more impressive sensitivity for accepting the electrons from NO , NO2 and N2O in the process of adsorption mechanism. However, carbon-doped on B5N10 has shown the lowest fluctuation between Bader charge versus electric potential extract from NQR parameters and the highest negative atomic charge including 0.1190 coulomb in NO→C – B4N10, 0.1844 coulomb in NO2→C – B4N10, and 0.1312 coulomb in N2O→C – B4N10 which can be an appropriate option with the highest tendency for electron accepting in the adsorption current (). In fact, the uptake of gas molecules has been known to be associated with C – B4N10, indicating that the adsorbed gas molecules in the C-doped nanocage can be internalized through a different pathway from pristine nanocage.
3.3. Analysis of NMR spectrums
Based on resulted amounts, nuclear magnetic resonance (NMR) spectrums of C – B4N10 as the potent sensor for adsorbing toxic gas molecules containing NO, NO2, N2O molecules can unravel the efficiency of C – B4N10 for detecting and removing of these hazardous gases in air as an ecofriendly approach.
From the DFT calculations, it has been attained the chemical shielding (CS) tensors in the principal axes system to estimate the isotropic chemical-shielding (CSI) and anisotropic chemical-shielding (CSA) [Citation41]:
The NMR data of isotropic (σiso) and anisotropic shielding tensors (σaniso) of gas molecules trapped in the C – B4N10 towards formation of NO→C – B4N10, NO2→C – B4N10, and N2O→C – B4N10 complexes have been computed by Gaussian 16 revision C.01 program package [Citation35] and been shown in .
Table 2. Data of NMR shielding tensors for selected atoms of NO→C – B4N10, NO2→C – B4N10, and N2O→C – B4N10 complexes using CAM – B3LYP – D3/6–311+G (d,p), LANL2DZ calculation.
In , NMR data has reported the notable amounts for NO, NO2, N2O which were adsorbed on the C – B4N10 as the selective sensor for detecting gas molecules in air. The observed increase in the chemical shift anisotropy spans for N atoms, O(2) for NO/N2O adsorption and O(2)/O(18) for NO2 adsorption on the C – B4N10. The observed weak signal intensity near the parallel edge of the nanocage pattern may be due to boron binding induced non-spherical distribution of these complexes.
It is remarkable that doping of C on B5N10 might promote the stability of nanocage that results in enhanced magnetic alignment of complexes. Interestingly, the reported results show that C element can be optimized to achieve optimal alignment of nanocage in the presence of an applied magnetic field.
In fact, the adsorption of NO, NO2, N2O can introduce spin polarization on the C – B4N10 which indicates that these surfaces might be applied as magnetic scavenging surface as a gas detector. Isotropic and anisotropic shielding fluctuate with the occupancy in the electron accepting gas molecules trapped in the atom-doped on the boron nitride nanocage.
exhibited the same tendency of shielding for boron and nitrogen; however, a considerable deviation exists from doping atom of C (15) through interaction with N (1) of NO, NO2, N2O during adsorbing on the B5N10.
Figure 4. The NMR spectrums for a) NO→C–B4N10, b) NO2→C–B4N10, and c) and N2O→C–B4N10 complexes using CAM–B3LYP–D3/6–311+G (d,p), LANL2DZ.
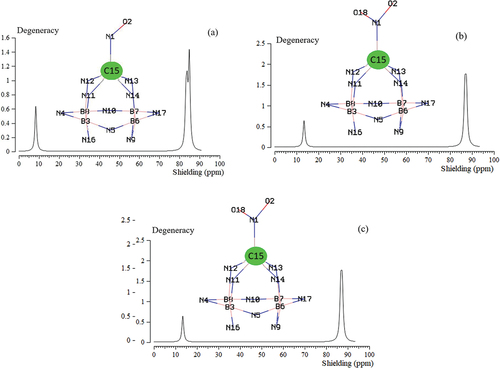
In , gas molecules of NO, NO2, N2O in the complexes of NO→C – B4N10 , NO2→C – B4N10 , and N2O→C – B4N10 denote the fluctuation in the chemical shielding during ion trapping. shows the gap chemical shielding between carbon doping of C – B4N10 nanocage and gas molecules.
In the NMR spectroscopy, it has been observed the remarkable peaks around interaction of NO, NO2, N2O molecules through adsorbing on the C – B4N10 during toxic gas detection and removal from air; however, there are some fluctuations in the chemical shielding behaviours of isotropic and anisotropy attributes. Therefore, the authors believe that the extracted results would be useful in the design of C – B4N10 based doped nanomaterials for increasing the adsorption of gas molecules in addition to the structural studies using solid-state and solution NMR techniques.
3.4. IR spectroscopy and thermodynamic factors
The IR calculations have been accomplished for adsorption of NO, NO2, N2O molecules by C – B4N10 during toxic gas sensing in air. Therefore, it has simulated the several clusters containing NO→C – B4N10 , NO2→C – B4N10 , and N2O→C – B4N10 ) complexes using CAM – B3LYP – D3/6–311+G (d,p), LANL2DZ.
Figure 5. The frequency (cm−1) changes through the IR spectrums for a) NO→C–B4N10, b) NO2→C–B4N10, and c) and N2O→C–B4N10 complexes using CAM–B3LYP–D3/6–311+G (d,p), LANL2DZ.
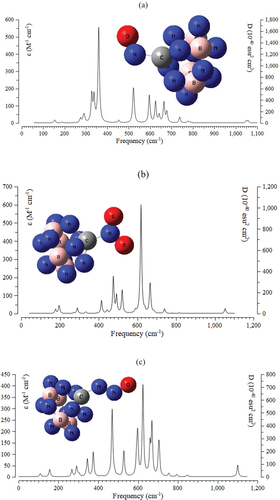
The graph of has been observed in the frequency range between 200–800 cm−1 for NO→C – B4N10 with several sharp peaks around 327.09, 337.61, 359.54, 595.74 and 664.20 cm−1.
The graph of has been observed in the frequency range between 200–1100 cm−1 for NO2→C – B4N10 with several sharp peaks around 414.80, 475.93, 492.51, 521.43, 618.93, 664.49, and 666.55 cm−1. Besides, has exhibited the frequency between 100–1200 for N2O →C – B4N10 with sharp peaks around 345.39, 374.87, 469.76, 528.65, 592.59, 597.92, 624.44, 659.94, 669.69, 702.84, and 706.06 cm−1.
The IR spectra of NO, NO2, N2O adsorption with C – B4N10 have demonstrated that the structure of the dominant complex correlates with the electron potency of doped C on the B5N10. As it has been seen the doped nanocage of C – B4N10 has the most fluctuations and the highest tendency for adsorption of NO, NO2 and N2O . Therefore, it can be found that IR spectroscopy of (G)-adsorbed C – B4N10 is now well-placed to address specific questions on the individual effect of charge carriers (gas molecule-nanosensor), as well as doping atoms on the overall structure . The analytical IR spectroscopy has described herein the selection of experimental parameters, and data processing. Atmospheric NOx is primarily a consequence of the combustion of fossil fuels at high temperatures. However, NOx is also readily produced by other means, including the burning of biomass material, soil emissions, and lightning strikes. The procedure used in this research can be applied to the characterization of gaseous pollutants in any adsorption process. through the thermodynamic specifications concluded that C – B4N10 due to adsorption of NO, NO2 and N2O might be more efficient nanosensors for detecting and removing the gas molecules from polluted air.
Table 3. The thermodynamic characters of NO→C – B4N10, NO2→C – B4N10, and N2O→C – B4N10 complexes using CAM – B3LYP – D3/6–311+G (d,p), LANL2DZ calculation.
Thermodynamic parameters of gas molecules adsorption with C – B4N10 have been determined using DFT theoretical technique. It has been shown that for a given number of nitrogen donor sites in NO, NO2, N2O, the stabilities of complexes owing to doping atom of C be considered as: NO2→C – B4N10> N2O→C – B4N10 > NO→C – B4N10 ().
The thermodynamic data in could detect the maximum efficiency of C atom doping of B5N10 for gas molecules adsorption through which depends on the covalent bond between NO, NO2, N2O molecules and C – B4N10 as a potent sensor for air pollution removal. The adsorption process of NO, NO2, N2O molecules on the C – B4N10 is affirmed by the
quantities:
G = NO, NO2, N2O. has shown that the key role of doped atom of C during interaction between the adsorbates of NO, NO2, N2O molecules as the electron donors and the adsorbent of C – B4N10 as electron acceptor. The interaction of carbon and gas molecules with solid surfaces is discussed within the framework of physisorption and chemisorption. Hereby also the description of chemisorption processes in terms of multidimensional potential hypersurfaces obtained from density functional theory (DFT) is briefly presented. BN-based material can be a noteworthy alternative to other porous materials for the removal of exhaust gas pollutants. However, many steps are needed to synthesize porous BN materials with a good composition, structure and porosity. Besides, it is suspected that defects within the BN structure or carbon-doping would be needed to ameliorate gas sorption. In this research, a porous carbon-doped BN is simulated in order to increase its gas adsorption efficiency.
4. Conclusion
Atmospheric NOx is primarily a consequence of the combustion of fossil fuels at high temperatures. However, NOx is also readily produced by other means, including the burning of biomass material, soil emissions, and lightning strikes. The procedure used in this research can be applied to the characterization of gaseous pollutants in any adsorption process. This research has investigated doping of C element on the boron nitride nanosensor (C – B4N10) for enhancing toxic gas sensing of these nanomaterials for air pollution removal. Therefore, NO, NO2, N2O molecules separation involving the C – B4N10 has been experimented based on electrostatic interactions between the gas molecules and C – B4N10. The electromagnetic and thermodynamic properties of C – B4N10 complex were computed using density functional theory. The results have illustrated that chosen gas molecules adsorbed on the C – B4N10 are rather stable with the most stable adsorption site being in the centre of C – B4N10 system. The doping atom of C (15) on the B4N10 has shown the most potential for accepting the electron from electron donor of N (1) in NO, NO2, N2O adsorbed on the B5N10. Moreover, Gibbs free energy alteration has shown that for a given number of nitrogen donor sites in NO, NO2, N2O, the stabilities of complexes owing to doping atom of C be considered as: NO2→C – B4N10> N2O→C – B4N10 > NO→C – B4N10. Indeed, it has been proven that carbon-doping alters the surface chemistry of boron nitrides and creates structural defects, which increases the number of active sites such as basic N groups suitable for NO, NO2, N2O adsorption. The above analysis shows that we can use the adsorbed phase as a small thermodynamic system. This allows the close relationship between the subdivision potential and the dependence of intensive properties on the internal structure of nanomaterials to be considered for gas adsorption.
Acknowledgements
In successfully completing this paper and its research, the authors are grateful to Kastamonu University.
Disclosure statement
No potential conflict of interest was reported by the author(s).
References
- Jakšić O, Jokić I, Jakšić Z, et al. The time response of plasmonic sensors due to binary adsorption: analytical versus numerical modeling. Appl Phys A. 2020;126(5):342. doi: 10.1007/s00339-020-03524-3
- Mollaamin F, Monajjemi M. The influence of Sc, V, Cr, Co, Cu, Zn as ferromagnetic semiconductors implanted on B5N10-nanocarrier for enhancing of NO sensing: an environmental eco-friendly investigation. Comput Theor Chem. 2024;1237(July):114666. doi: 10.1016/j.comptc.2024.114666
- Mollaamin F, Monajjemi M. Tailoring and functionalizing the graphitic-like GaN and GaP nanostructures as selective sensors for NO, NO2, and NH3 adsorbing: a DFT study. J Mol Model. 2023;29(6):170. doi: 10.1007/s00894-023-05567-8
- González-Hernández JL, Canedo MM, Encinar S. Application of a robust hybrid algorithm (neural networks-AGDC) for the determination of kinetic parameters and discrimination among reaction mechanisms. MATCH Commun Math Comput Chem. 2018;79:619–644.
- Mollaamin F, Monajjemi M. Transition metal (X = Mn, Fe, Co, Ni, Cu, Zn)-doped graphene as gas sensor for CO2 and NO2 detection: a molecular modeling framework by DFT perspective. J Mol Model. 2023;29(4):119. doi: 10.1007/s00894-023-05526-3
- Mollaamin F, Monajjemi M. In situ Ti-embedded SiC as chemiresistive nanosensor for safety monitoring of CO, CO2, NO, NO2: molecular modelling by conceptual density functional theory. Russ J Phys Chem B. 2024;18(1):49–66. doi: 10.1134/S1990793124010159
- Anderson R, Rodgers J, Argueta E et al. Role of pore chemistry and topology in the CO2 capture capabilities of MOFs: from molecular simulation to machine learning. Chem Mater. 2018;30(18):6325–6337. doi: 10.1021/acs.chemmater.8b02257
- Gonzalez-Ortiz D, Salameh C, Bechelany M et al. Nanostructured boron nitride-based materials: synthesis and applications. Mater Today Adv. 2020;8:100107. doi: 10.1016/j.mtadv.2020.100107
- Mishra NS, Saravanan P. A review on the synergistic features of hexagonal boron nitride (white graphene) as adsorbent-photo active nanomaterial. ChemistrySelect. 2018;3(28):8023–8034. doi: 10.1002/slct.201801524
- Weng QH, Wang XB, Wang X et al. Functionalized hexagonal boron nitride nanomaterials: emerging properties and applications. Chem Soc Rev. 2016;45(14):3989–4012. doi: 10.1039/C5CS00869G
- Delia Ocotitla Muñoz A, Escobedo-Morales A, Skakerzadeh E. Ernesto Chigo Anota, effect of homonuclear boron bonds in the adsorption of DNA nucleobases on boron nitride nanosheets. J Mol Liquids. 2021;322:114951. doi: 10.1016/j.molliq.2020.114951
- Shtansky DV, Matveev AT, Permyakova ES et al. Recent progress in fabrication and application of BN nanostructures and BN-Based nanohybrids. Nanomaterials. 2022;12(16):2810. doi: 10.3390/nano12162810
- Yang Y, Peng Y, Saleem MF et al. Hexagonal boron nitride on III–V compounds: a review of the synthesis and applications. Materials. 2022;15(13):4396. doi: 10.3390/ma15134396
- Davies A, Albar JD, Summerfield A, et al. Lattice-matched epitaxial graphene grown on boron nitride. Nano Lett. 2018;18(1):498–504. doi: 10.1021/acs.nanolett.7b04453
- Bangari RS, Yadav VK, Singh JK et al. Fe3O4-functionalized boron nitride nanosheets as novel adsorbents for removal of arsenic (III) from contaminated water. ACS Omega. 2020;5(18):10301–10314. doi: 10.1021/acsomega.9b04295
- Chao YH, Zhang J, Li HP, et al. Synthesis of boron nitride nanosheets with N-defects for efficient tetracycline antibiotics adsorptive removal. Chem Eng J. 2020;387:124138. doi: 10.1016/j.cej.2020.124138
- Khaleghian MZ, Mollaamin M, Monajjemi M. Investigation of solvent effects on armchair single-walled carbon nanotubes: A QM/MD study, fuller. Nanotub Carbon Nanostruct. 2011;19(4):2011)251–261. doi: 10.1080/15363831003721757
- Shahriari S, Soofi NS, Farzi F, et al. Interaction of nano-boron nitride/graphene sheets with anode lithium ion battery. J Comput Theor Nanosci. 2016;13(5):3070–3082. doi: 10.1166/jctn.2016.4959
- Guo Y, Wang RX, Wang PF et al. Developing a novel layered boron nitride–carbon nitride composite with high efficiency and selectivity to remove protonated dyes from water. ACS Sustainable Chem Eng. 2019;7(6):5727–5741. doi: 10.1021/acssuschemeng.8b05150
- Henkelman G, Arnaldsson A, Jónsson H. A fast and robust algorithm for bader decomposition of charge density. Comput Mater Sci. 2006;36(3):354–360. doi:10.1016/j.commatsci.2005.04.010
- Blöchl PE. Projector augmented-wave method. Phys Rev B. 1994;50(24):17953–17979. doi: 10.1103/PhysRevB.50.17953
- Perdew JP, Burke K, Ernzerhof M. Generalized gradient approximation made simple. Phys Rev Lett. 1996;77(18):3865. doi: 10.1103/PhysRevLett.77.3865
- Ziesche P, Kurth S, Perdew JP. Density functionals from LDA to GGA. Comput Mater Sci. 1998;11(2):122–127. doi: 10.1016/S0927-0256(97)00206-1
- Arrigoni M, Madsen GKH. Comparing the performance of LDA and GGA functionals in predicting the T lattice thermal conductivity of III-V semiconductor materials in the zincblende structure: the cases of AlAs and BAs. Comput Mater Sci. 2019;156:354–360. doi: 10.1016/j.commatsci.2018.10.005
- Hohenberg P, Kohn W. Inhomogeneous electron gas. Phys Rev B. 1964;136(3B):B864–B871. doi: 10.1103/PhysRev.136.B864
- Kohn W, Sham LJ. Self-consistent equations including exchange and correlation effects.Phys. Phys Rev. 1965;140(4A):A1133–A1138. doi: 10.1103/PhysRev.140.A1133
- Becke AD. Density-functional thermochemistry. III. The role of exact exchange. J Chem Phys. 1993;98(7):5648–5652. doi: 10.1063/1.464913
- Lee C, Yang W, Rg P. Development of the colle–salvetti correlation-energy formula into a functional of the electron density. Phys Rev B. 1988;37(2):785–789. doi: 10.1103/PhysRevB.37.785
- Kim K, Jordan KD. Comparison of density functional and MP2 calculations on the water monomer and dimer. J Phys Chem. 1994;98(40):10089–10094. doi: 10.1021/j100091a024
- Stephens PJ, Devlin FJ, Chabalowski CF et al. Ab initio calculation of vibrational absorption and circular dichroism spectra using density functional force fields. J Phys Chem. 1994;98(45):11623–11627. doi: 10.1021/j100096a001
- Cramer CJ. Essentials of computational chemistry: theories and Models. 2nd ed. Minneapolis, USA: Wiley; 2004.
- Mollaamin F, Monajjemi M. Corrosion inhibiting by some organic heterocyclic inhibitors through langmuir adsorption mechanism on the Al-X (X = mg/Ga/Si) alloy surface: a study of quantum three-layer method of CAM-DFT/ONIOM. J Bio Tribo Corros. 2023;9(33). doi: 10.1007/s40735-023-00751-y
- Mollaamin F, Monajjemi M. Molecular modelling framework of metal-organic clusters for conserving surfaces: langmuir sorption through the TD-DFT/ONIOM approach. Mol Simulat. 2023;49(4):365–376. doi: 10.1080/08927022.2022.2159996
- Vosko SH, Wilk L, Nusair M. Accurate spin-dependent electron liquid correlation energies for local spin density calculations: a critical analysis. Can J Phys. 1980;58(8):1200–1211. Bibcode:1980CaJPh.58.1200V. doi:10.1139/p80-159
- Frisch MJ, Trucks GW, Schlegel HB, et al. Gaussian 16. Wallingford (CT): Gaussian, Inc; 2016, Revision C.01.
- GaussView. Version 6.06.16, Dennington, Roy;Keith, Todd A.;Millam, John M. Semichem Inc. 2016, Shawnee Mission (KS).
- Mollaamin F, Monajjemi M. Adsorption ability of Ga5N10 nanomaterial for removing metal ions contamination from drinking water by DFT. Int J Quantum Chem. 2024;124(2):e27348. doi: 10.1002/qua.27348
- Mollaamin F, Monajjemi M. In silico-DFT investigation of nanocluster alloys of Al-(Mg, Ge, Sn) coated by nitrogen heterocyclic carbenes as corrosion inhibitor. J Clust Sci. 2023;34(6):2901–2918. doi: 10.1007/s10876-023-02436-5
- Mollaamin F, Shahriari S, Monajjemi M et al. Nanocluster of aluminum lattice via organic inhibitors coating: a study of freundlich adsorption. J Clust Sci. 2023;34(3):1547–1562. doi: 10.1007/s10876-022-02335-1
- Mollaamin F, Monajjemi M. Trapping of toxic heavy metals from water by GN–nanocage: application of nanomaterials for contaminant removal technique. J Mol Struct. 2024;1300:137214. doi: 10.1016/j.molstruc.2023.137214
- Sohail U, Ullah F, Binti Zainal Arfan NH et al. Transition metal sensing with nitrogenated holey graphene: a first-principles investigation. Molecules. 2023;28(10):4060. doi: 10.3390/molecules28104060