ABSTRACT
We investigated the relationship between performance-related anxiety and the neural response to errors. Using the sport anxiety scale, we selected university athletes high in sports anxiety and low in sports anxiety. The two groups performed a spatial Stroop task while their performance was being evaluated by an experimenter and also during a control (i.e., no evaluation) condition. The error-related negativity was significantly larger during the evaluation than control condition among athletes who reported high performance-related anxiety. These results suggest that performance evaluation may make errors particularly aversive or salient for individuals who fail to perform well under pressure.
Introduction
Competitive athletes are commonly considered to be among the healthiest individuals, except for exposure to risks from potential injuries (Pontifex, O’Connor, Broglio, & Hillman, Citation2009). However, a common phenomenon is for some athletes to “choke” (i.e., perform poorly) under pressure due to anxiety that occurs preceding or during an important contest (Baumeister, Citation1984). For these individuals, performance is worse than expected in evaluative contexts (Beilock & Carr, Citation2001; DeCaro, Thomas, Albert, & Beilock, Citation2011). Choking under pressure can be empirically induced by pressure manipulations, including performance-contingent rewards, presence of audiences, and video-recording of the performer—all of which enhance the importance of good performance (Wang, Marchant, Morris, & Gibbs, Citation2004).
Two conceptual approaches have been proposed to explain choking under pressure. Distraction theories propose that attentional resources are prefrentially allocated to task-irrelevant information including concern about the situation and the outcomes of a high pressure situation (Wine, Citation1971). For instance, a performance-anxious athlete may attend more to thoughts regarding potential failure. Thus, distraction resulting from this dual-task situation is thought to be responsible for deterioration of performance. A similar concept, processing efficiency theory, was also proposed (Eysenck & Calvo, Citation1992) that asserts that performance decrements occur when auxiliary resources are not available for execution of the required task because attentional capacity is consumed through worry.
On the other hand, explicit monitoring theories claim that performance pressure raises self-consciousness and anxiety regarding correct performance, and this may disrupt the automaticity of well-learned skilled movements, resulting in poorer performance (Baumeister, Citation1984; Beilock & Carr, Citation2001). The explicit monitoring theory suggests that individuals who choke under pressure might increase error monitoring during performance evaluation.
Over the past two decades, a large body of research has examined neural correlates of error monitoring. Specifically, studies using event-related brain potentials (ERPs) have focused on the error-related negativity (ERN or Ne)—a negative deflection in the ERP over frontal-central recording sites that is maximal approximately 50 ms after error commission (Falkenstein, Hohnsbein, Hoormann, & Blanke, Citation1990; Gehring, Coles, Meyer, & Donchin, Citation1990). Hajcak and colleagues have proposed that variability in the size of the ERN reflects the degree to which errors are aversive or threatening (Proudfit, Inzlicht, & Mennin, Citation2013; Weinberg, Riesel, & Hajcak, Citation2012). For instance, errors associated with greater value elicit a larger (i.e., more negative) ERN (Hajcak, Moser, Yeung, & Simons, Citation2005). Along the same lines, punishing errors increases the amplitude of the ERN—and this effect is larger among more anxious individuals (Riesel, Weinberg, Endrass, Kathmann, & Hajcak, Citation2012). Moreover, a recent study found that performance evaluation increased the ERN, but only among individuals who reported high levels of social anxiety (Barker, Troller-Renfree, Pine, & Fox, Citation2015). Another study found that the difference between the ERN and the neural response on correct trials was increased in a numerical task as a function of both performance evaluation and test anxiety (Schillinger, De Smedt, & Grabner, Citation2015). Collectively, these data suggest that performance assessment may potentiate error-related brain activity among individuals who are more sensitive to evaluation.
In the current study, we examined whether individuals high in sports anxiety are characterized by an abnormal neural response to errors in evaluative compared to control conditions. Although aforementioned hypothetical models of choking have been proposed, no neurophysiological studies have tested attentional demands associated with performance monitoring in sports anxious athletes. Thus, we aimed to clarify differences in performance monitoring between high and low sports anxious individuals. To this end, we selected university athletes who exhibit either extremely high or low sports anxiety, using the sport anxiety scale (SAS-2; Smith, Smoll, Cumming, & Grossbard, Citation2006). Individuals scoring high or low in sports anxiety then performed a spatial Stroop task while their performance was being evaluated by an experimenter and during a control (i.e., no evaluation) condition, mimicking the procedure of Hajcak et al. (Citation2005).
In line with explicit monitoring theories of performance-related anxiety, we predicted that the performance evaluation manipulation would have a larger impact on the ERN among those individuals high in sports anxiety. Recent studies found that effects of performance evaluation on the ERN were obvious for high anxious individuals but not for low anxious individuals (Barker et al., Citation2015; Riesel et al., Citation2012). We presumed that individuals high in sports anxiety would demonstrate a disproportional effect of performance evaluation, resulting in a larger impact of evaluation on the ERN for those participants high in sports anxiety. In addition, we expected no difference in ERN amplitudes between high and low sports anxious individuals in the control condition, when performance was not evaluated.
Because other studies have found that “choking” occurs in relatively complex tasks, including putting (e.g., golf; Beilock & Carr, Citation2001; Masters, Citation1992), free throw shooting (e.g., basketball; Mesagno, Harvey, & Janelle, Citation2012; Wang et al., Citation2004), and a roll-up game performed bimanually (Baumeister, Citation1984), it is possible that the evaluation condition may also impact behavioral measures (i.e., longer reaction times and reduced accuracy) among individuals high in sports anxiety. On the other hand, previous work suggests that behavioral measures in this type of speeded response task remain unaffected even in the face of relatively severe anxiety inductions (Moser, Hajcak, & Simons, Citation2005). Thus, it is possible that the interaction of sports anxiety and performance evaluation might impact neural, but not behavioral, indices in the current study.
Method
Participants
For recruiting individuals high and low on performance anxiety, the sport anxiety scale (SAS-2) was administered to 216 undergraduate athletes of Faculty of Sport Sciences, Waseda University. Although they were competitive athletes, their sport disciplines and events were different. Individual participants belonged to different divisions depending on their sports events. The SAS-2 is a 15-item measure that separately assesses somatic anxiety, worry, and concentration disruption; it has excellent psychometric properties (Smith, Smoll, & Cumming, Citation2007; Smith et al., Citation2006).
We invited those students who scored above 35 (i.e., higher sport anxiety) and below 20 (i.e., lower sport anxiety) to participate in a laboratory visit. These criteria were determined on the basis of ±1 standard deviation (SD) from the mean total SAS-2 score (M: 27.1, SD: 7.1). Consequently, 14 higher sports anxious individuals (7 females, mean age ± SD = 20.5 ± 1.29; SAS-2 range: 35–52) and 14 lower sports anxious individuals (8 females, mean age ± SD = 21.4 ± 2.34; SAS-2 range: 15–20) participated in this study. A t-test conducted on SAS-2 scores for higher (M: 37.9, SEM: 1.2) and lower sport anxious individuals (M: 17.2, SEM: 0.4) confirmed differences between the two groups (t(26) = 16.13, p < .01, d = 6.10).
Participants had normal or corrected-to-normal vision and were paid 3,200 yen (about 26 U.S. dollars) for their participation. This study was approved by local Ethics Committee and informed consent was obtained from all participants.
Apparatus and procedure
Task
To obtain sufficient errors, we adopted a spatial Stroop task that would produce relatively large interference effects (Masaki, Murphy, Desjardins, & Segalowitz, Citation2012; Masaki & Segalowitz, Citation2004). Each trial began with a central 300 ms fixation cross (0.7° × 0.7°). Then, a white arrow (pointing up or down; 0.7° × 0.4°) was presented above or below the fixation with an eccentricity of 0.8° visual angle for 150 ms. This was followed by 1000 ms duration of blank screen after the stimulus offset until the next trial, which began with a fixation cross. The participants were instructed to respond to the pointing direction of the white arrow stimulus (i.e., up or down), but not to the location (i.e., above or below fixation), by quickly lifting their middle fingers. Congruent stimuli (i.e., the arrow pointing up presented above the fixation and the arrow pointing down below the fixation) and incongruent stimuli (i.e., the arrow pointing down presented above the fixation and the arrow pointing up below the fixation) were randomly presented with 50% probability. Responses were recorded with two microswitch keys placed on a flat board (445 × 910 × 25 mm) 150 mm apart from each other in the participant’s midsagittal plane. Hand placement was counter-balanced across upper (i.e., further) and lower (i.e., nearer) keys. RT was measured as the interval between the stimulus onset and the microswitch closure. Participants were requested to respond with both speed and accuracy. Participants also completed a time-estimation task following the spatial Stroop task, though these results will be reported elsewhere.
Performance evaluation manipulation
Each participant performed the spatial Stroop task in both a performance evaluation condition and a control condition. The performance evaluation manipulation was modeled on the study by Hajcak et al. (Citation2005). During the evaluation condition, participants were told that their performance was being evaluated online by a research assistant, and that the research assistant would compare the participant’s performance to other students who had performed the task; during the evaluation condition, the research assistant was seated on the right side behind the participant with a laptop computer. During the control condition, participants were left alone in room and performed the same task without any evaluation. The participants performed 4 blocks of 72 trials in each condition (i.e., evaluation/control). The order of condition was counter-balanced across participants using either ABBA or BAAB sequence.
EEG recording
he electroencephalogram (EEG) was recorded from 128 sites with Ag/AgCl electrodes embedded in a nylon mesh cap, according to the Biosemi electrode coordinates (Biosemi Inc.) that partly share the identical electrode sites with the international 10–10 system (Oostenveld & Praamstra, Citation2001). Horizontal electrooculograms (hEOG) were recorded from the left and right outer canthi, and vertical electrooculograms (vEOG) from above and below the left eye. These were recorded with a bandwidth of DC to 205 Hz, using a Biosemi Active Two system (Biosemi Inc.). The Biosemi system uses an active electrode system that amplifies EEG signals at the electrode site, thus drastically reducing 50 Hz interference that is typically introduced through electrode wires. The system requires minimal skin preparation to reduce impedance, and uses “offset” units to assure high quality data. Throughout the experiment, the EEG was digitized at a rate of 1024 Hz from 128 sites with Ag/AgCl electrodes and offsets were kept below 20, which is consistent with other studies using the Biosemi system.
Data analysis
Processing of EEG data was performed with Brain Vision Analyzer (Brain Products). The EEG was re-referenced to the average reference, band-pass filtered 0.1–30 Hz (roll-off 12 dB), and segmented for each trial beginning 800 ms before each response onset and continuing for 1600 ms (i.e., for 800 ms following the response). Ocular movement artifacts were corrected using the procedure described by Gratton, Coles, and Donchin (Citation1983). Response-locked ERPs were averaged separately for correct and incorrect trials; only incongruent trials were analyzed in this study. We excluded from ERP averaging those trials in which EEG voltages exceeded a threshold of 100 µV during the recording epoch (e.g., Bonnefond, Doignon-Camus, Hoeft, & Dufour, Citation2011; Larson, Gray, Clayson, Jones, & Kirwan, Citation2013; Masaki, Murphy, Kamijo, Yamazaki, & Sommer, Citation2012). Visual inspection of the data was then conducted to detect and reject any remaining artifacts.
The ERN was measured as the average of activity on error trials in a window spanning 20 ms to 100 ms after response onset at FCz (C23 of the Biosemi coordinates) relative to a pre-response baseline. The baseline was defined as mean voltage from 50 to 20 ms before the response in the averaged ERP waveform. We adopted an area measurement, as area measures of the ERN have been widely accepted in recent ERN studies (e.g., Hajcak et al., Citation2005).Footnote1
ERN amplitudes were subjected to a mixed model repeated measures ANOVA with group (high vs. low sports anxiety) as a between-subjects factor and condition (evaluation vs. control) as a within-subjects factor. To demonstrate specificity, we conducted an analogous analysis of the correct response negativity (CRN) that is observed after the correct response (Ford, Citation1999; Vidal, Hasbroucq, Grapperon, & Bonnet, Citation2000), scored as the average activity from 20 ms to 100 ms after correct responses at FCz. Along the same lines, RT and error rate were evaluated using a group (high vs. low sports anxiety), trial type (congruent vs. incongruent), and condition (evaluation vs. control) repeated measures mixed model ANOVA. All statistical analyses were conducted using SPSS (Version 20.0).
Results
Reaction time
(left panel) shows mean RT on correct trials. A three-way ANOVA revealed longer RT for the incongruent condition than the congruent condition (F(1, 26) = 417.53, p < .001, pη2 = .94). There were no significant effects for group (F(1, 26) = .04, p = .85, pη2 = .001) or evaluation (F(1, 26) = .02, p = .88, pη2 = .001). There were also no significant two-way or three-way interactions.
Error rate
(right panel) presents error rates. A three-way ANOVA revealed a higher error rate for incongruent than for congruent trials (F(1, 26) = 91.13, p < .001, pη2 = .78). Neither group (F(1, 26) = .003, p = .96, pη2 = .000) nor evaluation effect (F(1, 26) = .71, p = .41, pη2 = .026) was found. Neither three-way interaction nor two-way interactions reached significance.
Error-related negativity (ERN)
(top) depicts the grand-averaged ERPs at FCz relative to the response onset (vertical dotted line) for high (left) and low (right) sports anxious individuals; the scalp topographies of the ERN on error trials are also presented (bottom). In both groups, a frontal-centrally distributed ERN emerged, peaking ~50 ms after error responses. Average ERN amplitudes as a function of group and condition are presented in .
Figure 2. Response-locked grand averaged waveforms of the ERNs and correct response negativities (CRNs) on correct trials. The vertical bars represent the response onset. Scalp topographies of the delta ERN (i.e., error minus correct response) in each condition represent activities ranging from 20 to 102 ms following the button press.
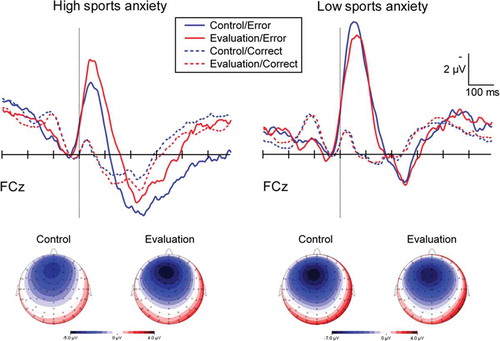
Figure 3. Mean ERN amplitudes both in the control and the evaluation conditions for athletes high and low in sport anxiety. Error bars represent mean of standard error (SEM).
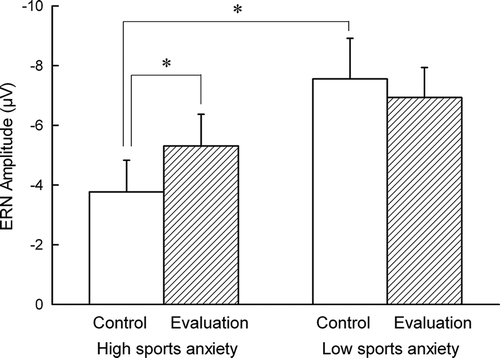
Consistent with the impression from and , the ERN did not vary overall by condition (F(1, 26) = .90, p = .35, pη2 = .03). Overall, high sports anxious individuals were characterized by a smaller (i.e., less negative) ERN compared to low sports anxious individuals, at a trend level (F(1, 26) = 3.16, p = .09, pη2 = .11). However, there was a significant interaction between evaluation condition and group (F(1, 26) = 4.96, p = .04, pη2 = .16). Post-hoc paired samples t-tests revealed that for the higher sports anxious group, the ERN was larger in the evaluation condition than in the control condition (t(13) = 2.75, p = .02, d = -.39). However, for the lower sport anxiety group, the ERN did not differ between conditions (t(13) = .79, p = .45, d = .14). In addition, the ERN was significantly larger for the lower sport anxiety group than for the higher sport anxiety group in the control condition (t(13) = 2.20, p = .04, d = .83). The ERN did not differ between groups in the evaluation condition (t(13) = 1.11, p = .28, d = .42). An identical analysis of the CRN revealed no main effect of condition, group, or interaction (all ps > .40).
Discussion
Among competitive university athletes, we investigated the relationship between sports anxiety and neural responses to errors both when performance was evaluated and in a control condition. In the spatial Stroop task, no behavioral differences between conditions were evident between groups, indicating that sports anxious individuals did not actually perform worse; they did not choke under pressure in this relatively simple speeded response task (Baumeister, Citation1984). On the other hand, the ERN was significantly larger in the evaluation condition than in the control condition for the higher sports anxiety group, but not for the lower sports anxiety group. In terms of the controversy regarding the explicit monitoring theory (Wine, Citation1971) and the distraction theory (Baumeister, Citation1984) of choking under pressure, our results do not support the distraction theory. If the distraction theory is correct (i.e., if athletes high in sports anxiety allocated more attention to worry regarding performance failure in the evaluation condition), the ERN should have been smaller in the evaluation condition than in the control condition, as previous studies have suggested that the ERN is smaller when less attention is allocated to the task (Gehring, Goss, Coles, Meyer, & Donchin, Citation1993; Tanaka, Mochizuki, Masaki, Takasawa, & Yamazaki, Citation2005). Indeed, the results were in the opposite direction: the enhanced ERN under evaluation condition suggests that inward attention to performance monitoring increased during evaluation among those who tend to choke under pressure. Thus, our results favor the explicit monitoring theory more than the distraction theory.
The evaluation effect on the ERN observed for athletes high in sports anxiety is consistent with previous findings (Hajcak et al., Citation2005). Hajcak et al. found that performance evaluation increased the ERN among an unselected group of college students. They attributed the larger ERN amplitude to the increased motivational significance of errors in the performance evaluation condition. Riesel and colleagues (Citation2012) found that punishing errors potentiated the ERN, and more so for individuals high in trait anxiety. In a more recent study, Barker and colleagues found that performance evaluation only potentiated the ERN among more socially anxious participants (Barker et al., Citation2015). Our results are quite similar to Barker and colleagues, and demonstrate that internal performance monitoring reflected in the ERN may be enhanced under performance evaluation for individuals who are especially anxious about failure (Barker et al., Citation2015). Thus, the ERN appears to reflect the interaction between trait and contextual variables that impact the neural response to errors (see reviews, Proudfit et al., Citation2013; Weinberg et al., Citation2012).
Recently, Meyer and colleagues (2014) found that children with more critical parents at age 3 were subsequently characterized by a larger ERN at age 6. Brooker and Buss (Citation2014) similarly found that harsh parenting related to an increased ERN in children. Collectively, these data suggest that punitive experiences surrounding error commission may potentiate the ERN and sensitize individuals to their mistakes. It would be interesting to examine whether performance anxious individuals are more likely to respond negativity to harsh or critical feedback and if this process explains their increased ERN under performance evaluation. It is important to note that high sports anxious individuals were actually characterized by a smaller (i.e., less negative) ERN than low sports anxious individuals in the control condition. One possibility is that highly sports anxious individuals—those who are most susceptible to performance evaluation—actually attend less to their errors when practicing and performing alone. That is, the current findings suggest that individuals high in sports anxiety may differ from individuals low in sports anxiety both in terms of the impact of performance evaluation on error monitoring, and in terms of the degree to which they monitor errors when alone. Larger studies are necessary to further examine whether sports anxious individuals are characterized by a reduced ERN when alone.
It is also important to note that high sports anxious individuals did not actually perform worse during evaluation (i.e., they did not “choke” under pressure, by definition). One likely reason for this is the relative simplicity of the task employed to elicit the ERN. Previous studies suggested that the relationship between performance decrements and self-consciousness underpinning choking might not be obtained when simple tasks are utilized (Masters, Polman, & Hammond, Citation1993; Wang et al., Citation2004). Indeed, even when performing this type of speeded response task in the presence of a phobic object, behavioral measures can be unaffected (Moser et al., Citation2005). In addition, recent studies have emphasized the importance of the quality of movement (i.e., kinematic variables) associated with choking rather than performance outcomes (Cooke, Kavussanu, McIntyre, & Ring, Citation2010; Tanaka & Sekiya, Citation2010). In a golf putting task, kinematics of swing (i.e., linear amplitude of the left elbow both in the back swing and the forward swing) were deteriorated during a pressure test even though putting scores did not decrease (Tanaka & Sekiya, Citation2010). These suggest that behavioral measures of performance might not be sufficient to fully evaluate processes related to choking.
Even in our simple task, however, neural correlates of error monitoring reflected differences in sports anxiety—but only in the evaluation condition. One avenue for future research is to relate evaluation-related increases in the ERN to actual choking behavior in real life contests. Another possibility would be to examine whether learning to reduce the ERN under evaluation conditions would lead to changes in performance-related anxiety.
It may be worthwhile to note limitations of this study. Firstly, the sample size was relatively small, and these findings require replication in larger samples. Secondly, as discussed earlier, because participants did not show deteriorated performance (i.e., no choking by definition) in our simple task, more complex and skill-oriented tasks should be used to observe choking in future studies. Third, we only utilized the SAS-2, and future studies may wish to adopt a battery with other sports-related questionnaires.
In sum, our results suggest that athletes who are prone to choke under pressure engage in excessive error monitoring during performance evaluation. These results are consistent with the view that performance anxiety involves abnormal attention to internal monitoring processes, especially in the presence of performance evaluation. These findings are consistent with previous studies linking the ERN to the motivational value and salience of errors (Proudfit et al., Citation2013; Riesel et al., Citation2012; Weinberg et al., Citation2012)—and suggest that errors may become exaggerated in value during performance evaluation among those who have a propensity to choke under pressure. In the control condition, we found smaller neural responses to errors among high sports anxious individuals, which may suggest that these individuals differ in terms of both their sensitivity to performance evaluation, and the degree to which they monitor their errors when alone. Future work might further examine the relationship between neural response to errors under performance evaluation and choking under pressure to determine the causal relationship between these phenomena.
Funding
Support for this research came from a grant from the Japanese Society for the Promotion of Science (JSPS Postdoctoral Fellowship Program for North American and European Researchers, 2010) to G. Hajcak, and from a Grant-in-Aid for challenging Exploratory Research 15K12657 from JSPS to H. Masaki, and a MEXT-Supported Program for the Strategic Research Foundation at Private Universities, 2015-2019 (S1511017).
Additional information
Funding
Notes
1. Our scoring procedure was not necessarily identical to previous studies in terms of the time window for both the pre-response baseline and the ERN amplitude; however, other area and baseline measures produced consistent results.
References
- Barker, T. V., Troller-Renfree, S., Pine, D. S., & Fox, N. A. (2015). Individual differences in social anxiety affect the salience of errors in social contexts. Cognitive, Affective, & Behavioral Neuroscience, 1–13. doi:10.3758/s13415-015-0360-9
- Baumeister, R. F. (1984). Choking under pressure: Self-consciousness and paradoxical effects of incentives on skillful performance. Journal of Personality and Social Psychology, 46(3), 610–620. doi:10.1037/0022-3514.46.3.610
- Beilock, S. L., & Carr, T. H. (2001). On the fragility of skilled performance: What governs choking under pressure? Journal of Experimental Psychology: General, 130(4), 701–725. doi:10.1037/0096-3445.130.4.701
- Bonnefond, A., Doignon-Camus, N., Hoeft, A., & Dufour, A. (2011). Impact of motivation on cognitive control in the context of vigilance lowering: An ERP study. Brain and Cognition, 77(3), 464–471. doi:10.1016/j.bandc.2011.08.010
- Brooker, R. J., & Buss, K. A. (2014). Harsh parenting and fearfulness in toddlerhood interact to predict amplitudes of preschool error-related negativity. Developmental Cognitive Neuroscience, 9, 148–159. doi:10.1016/j.dcn.2014.03.001
- Cooke, A., Kavussanu, M., McIntyre, D., & Ring, C. (2010). Psychological, muscular and kinematic factors mediate performance under pressure. Psychophysiology, 47(6), 1109–1118. doi:10.1111/j.1469-8986.2010.01021.x
- DeCaro, M. S., Thomas, R. D., Albert, N. B., & Beilock, S. L. (2011). Choking under pressure: Multiple routes to skill failure. Journal of Experimental Psychology: General, 140(3), 390–406. doi:10.1037/a0023466
- Eysenck, M. W., & Calvo, M. G. (1992). Anxiety and performance: The processing efficiency theory. Cognition & Emotion, 6(6), 409–434. doi:10.1080/02699939208409696
- Falkenstein, M., Hohnsbein, J., Hoormann, J., & Blanke, L. (1990). Effects of errors in choice reaction tasks on the ERP under focused and divided attention. In C. Brunia, A. Gaillard, & A. Kok (Eds.), Psychophysiological brain research (pp. 192–195). Tilburg, The Netherlands: Tilburg University Press.
- Ford, J. M. (1999). Schizophrenia: The broken P300 and beyond. Psychophysiology, 36(6), 667–682. doi:10.1111/psyp.1999.36.issue-6
- Gehring, W. J., Coles, M., Meyer, D., & Donchin, E. (1990). The error-related negativity: An event-related brain potential accompanying errors. Psychophysiology, 27, S34.
- Gehring, W. J., Goss, B., Coles, M. G., Meyer, D. E., & Donchin, E. (1993). A neural system for error detection and compensation. Psychological Science, 4(6), 385–390. doi:10.1111/j.1467-9280.1993.tb00586.x
- Gratton, G., Coles, M. G. H., & Donchin, E. (1983). A new method for off-line removal of ocular artifact. Electroencephalography and Clinical Neurophysiology, 55(4), 468–484. doi:10.1016/0013-4694(83)90135-9
- Hajcak, G., Moser, J. S., Yeung, N., & Simons, R. F. (2005). On the ERN and the significance of errors. Psychophysiology, 42, 151–160. doi:10.1111/j.1469-8986.2005.00270.x
- Larson, M. J., Gray, A. C., Clayson, P. E., Jones, R., & Kirwan, C. B. (2013). What are the influences of orthogonally-manipulated valence and arousal on performance monitoring processes? The effects of affective state. International Journal of Psychophysiology, 87(3), 327–339. doi:10.1016/j.ijpsycho.2013.01.005
- Masaki, H., Murphy, T. I., Desjardins, J. A., & Segalowitz, S. J. (2012). The error-related negativity associated with different strength of stimulus–response interference. Clinical Neurophysiology, 123(4), 689–699. doi:10.1016/j.clinph.2011.07.043
- Masaki, H., Murphy, T. I., Kamijo, K., Yamazaki, K., & Sommer, W. (2012). Foreshadowing of performance accuracy by event-related potentials: Evidence from a minimal-conflict task. Plos One, 7, e38006. doi:10.1371/journal.pone.0038006
- Masaki, H., & Segalowitz, S. (2004). Error negativity: A test of the response conflict versus error detection hypotheses. In M. Ullsperger, & M. Falkenstein (Eds.), Errors, conflicts, and the brain. Current opinions on performance monitoring (pp. 76–83). Leipzig, Germany: MPI of Cognitive Neuroscience.
- Masters, R. S. (1992). Knowledge, knerves and know-how: The role of explicit versus implicit knowledge in the breakdown of a complex motor skill under pressure. British Journal of Psychology, 83(3), 343–358. doi:10.1111/bjop.1992.83.issue-3
- Masters, R. S., Polman, R. C., & Hammond, N. (1993). “Reinvestment”: A dimension of personality implicated in skill breakdown under pressure. Personality and Individual Differences, 14(5), 655–666. doi:10.1016/0191-8869(93)90113-H
- Mesagno, C., Harvey, J. T., & Janelle, C. M. (2012). Choking under pressure: The role of fear of negative evaluation. Psychology of Sport and Exercise, 13(1), 60–68. doi:10.1016/j.psychsport.2011.07.007
- Meyer, A., Proudfit, G. H., Bufferd, S. J., Kujawa, A. J., Laptook, R. S., Torpey, D. C., & Klein, D. N. (2014). Self-reported and observed punitive parenting prospectively predicts increased error-related brain activity in six-year-old children. Journal of Abnormal Child Psychology, 1–9. doi:10.1007/s10802-014-9918-1
- Moser, J. S., Hajcak, G., & Simons, R. F. (2005). The effects of fear on performance monitoring and attentional allocation. Psychophysiology, 42(3), 261–268. doi:10.1111/j.1469-8986.2005.00290.x
- Oostenveld, R., & Praamstra, P. (2001). The five percent electrode system for high-resolution EEG and ERP measurements. Clinical Neurophysiology, 112(4), 713–719. doi:10.1016/S1388-2457(00)00527-7
- Pontifex, M. B., O’Connor, P. M., Broglio, S. P., & Hillman, C. H. (2009). The association between mild traumatic brain injury history and cognitive control. Neuropsychologia, 47(14), 3210–3216. doi:10.1016/j.neuropsychologia.2009.07.021
- Proudfit, G. H., Inzlicht, M., & Mennin, D. S. (2013). Anxiety and error monitoring: The importance of motivation and emotion. Frontiers in Human Neuroscience, 7. doi:10.3389/fnhum.2013.00636
- Riesel, A., Weinberg, A., Endrass, T., Kathmann, N., & Hajcak, G. (2012). Punishment has a lasting impact on error-related brain activity. Psychophysiology, 49, 239–247. doi:10.1111/j.1469-8986.2011.01298.x
- Schillinger, F. L., De Smedt, B., & Grabner, R. H. (2015). When errors count: An EEG study on numerical error monitoring under performance pressure. Zdm, 1–13. doi:10.1007/s11858-015-0746-8
- Smith, R. E., Smoll, F. L., & Cumming, S. P. (2007). Effects of a motivational climate iIntervention for coaches on young athletes’ sport performance anxiety. Journal of Sport & Exercise Psychology, 29, 39–59. doi:10.1123/jsep.29.1.39
- Smith, R. E., Smoll, F. L., Cumming, S. P., & Grossbard, J. R. (2006). Measurement of multidimensional sport performance anxiety in children and adults: The Sport Anxiety Scale-2. Journal of Sport & Exercise Psychology, 28, 479–501. doi:10.1123/jsep.28.4.479
- Tanaka, H., Mochizuki, Y., Masaki, H., Takasawa, N., & Yamazaki, K. (2005). A study of attentional resource of discrete and gradational allocation strategy using error-related negativity (ERN). Shinrigaku Kenkyu: The Japanese Journal of Psychology, 76(1), 43–50. doi:10.4992/jjpsy.76.43
- Tanaka, Y., & Sekiya, H. (2010). The influence of audience and monetary reward on the putting kinematics of expert and novice golfers. Research Quarterly for Exercise and Sport, 81(4), 416–424. doi:10.1080/02701367.2010.10599702
- Vidal, F., Hasbroucq, T., Grapperon, J., & Bonnet, M. (2000). Is the “error negativity” specific to errors? Biological Psychology, 51(2), 109–128. doi:10.1016/S0301-0511(99)00032-0
- Wang, J., Marchant, D., Morris, T., & Gibbs, P. (2004). Self-consciousness and trait anxiety as predictors of choking in sport. Journal of Science and Medicine in Sport, 7(2), 174–185. doi:10.1016/S1440-2440(04)80007-0
- Weinberg, A., Riesel, A., & Hajcak, G. (2012). Integrating multiple perspectives on error-related brain activity: The ERN as a neural indicator of trait defensive reactivity. Motivation and Emotion, 36(1), 84–100. doi:10.1007/s11031-011-9269-y
- Wine, J. (1971). Test anxiety and direction of attention. Psychological Bulletin, 76(2), 92–104. doi:10.1037/h0031332