ABSTRACT
The current study was designed to examine whether 1-year-old infants relate the perception of others’ being touched to their own sensorimotor system, and whether they distinguish between animate and inanimate targets. During electroencephalography (EEG) assessment, infants watched video sequences in which either a human or a nonhuman target was touched/not touched by another object. Comparisons of sensorimotor alpha activation (7–9 Hz) on centro-parietal electrodes revealed differential cortical reactivity to the touch versus nontouch situations for the human versus the nonhuman target. Our findings provide preliminary evidence for the claim that infants might relate others’ sensory experiences to their own sensorimotor system.
An essential developmental achievement for infants and fundamental prerequisite for successful interaction with the environment concerns the differentiation between animate and inanimate beings (e.g., Caramazza & Shelton, Citation1998; Rakison & Poulin-Dubois, Citation2001). Indeed, developmental research has shown that already in their first year of life young children treat animate and inanimate agents differently and have different expectations concerning their reactivity and future actions (e.g., Falck-Ytter, Gredebäck, & Von Hofsten, Citation2006; Johnson, Slaughter, & Carey, Citation1998; Kuhlmeier, Bloom, & Wynn, Citation2004; Woodward, Citation1998). For example, recent work demonstrated that 1-year-old infants expected that a human agent (i.e., a human hand) keeps grasping the same object even when the object’s location changed. A mechanical claw however, was expected to follow the exact same movement path independent of the object placed at the end location (Cannon & Woodward, Citation2012; Woodward, Citation1998).
Recent neuroimaging research has provided ample evidence that the perception of behavior by human agents leads to activation in the observer’s cortical sensorimotor system. This is true not only for human adults (e.g., Caetano, Jousmäki, & Hari, Citation2007; Decety, Citation2007; Pineda, Citation2005) but also for infants (e.g., Nyström, Ljunghammar, Rosander, & Von Hofsten, Citation2011; Paulus, Hunnius, & Bekkering, Citation2013; Reid, Striano, & Iacoboni, Citation2011; Saby, Marshall, & Meltzoff, Citation2012; Turati et al., Citation2013; for review see Saby & Marshall, Citation2012). These activations of the sensorimotor system through the observation of others’ actions have been shown to be affected by the observer’s own first-hand experience with the respective action (e.g., Buccino et al., Citation2004) and to be effector-specific (Saby, Metzoff, & Marshall, Citation2013). The findings have led to an intense discussion in developmental psychology (e.g., Cuevas & Paulus, Citation2016) and have given rise to theoretical approaches proposing that mirroring others’ actions within one’s own sensorimotor system plays an important role in understanding others, and learning from them (e.g., Casartelli & Molteni, Citation2014; Paulus, Citation2014; Quadrelli & Turati, Citation2015; Rizzolatti & Craighero, Citation2004).
Interestingly, recent studies with adults have shown that people not only resonate with observed actions, but they also seem to simulate others’ sensory experiences. Neuroimaging studies using functional magnetic resonance imaging (fMRI) in adults suggest that the somatosensory cortices are activated during the observation of others’ pain (e.g., Singer et al., Citation2004) and of another person being touched (e.g., Ebisch et al., Citation2008, Citation2011; Keysers, Kaas, & Gazzola, Citation2010; Kuehn, Müller, Turner, & Schütz-Bosback, Citation2014; Schaefer, Flor, Heinze, & Rotte, Citation2006; Schaefer, Xu, Flor, & Cohen, Citation2009), a phenomenon that is referred to as mirror touch. This work is complemented by investigations using event-related potentials (ERPs) demonstrating that the time-course of the activation of somatosensory processing is comparable during human and nonhuman touch observation at centro-parietal sites (Streltsova & McCleery, Citation2014). Based on these findings, it has been suggested that body representations are shared between self and other (e.g., De Vignemont, Citation2014), and that it might be the case that these phenomena play an important role for empathic reactions (Bolognini, Rossetti, Fusaro, Vallar, & Miniussi, Citation2014).
Thus, even though there are theoretical reasons to believe that the sharing of sensory experiences might have an influence on social behavior, little is known about its ontogenetic origins. Do infants process the perception of others being touched in their own sensorimotor system? There are theoretical reasons to believe that this might be the case. Influential theoretical accounts have argued that infants experience others’ sensations and emotions, creating thus a space of immediate and pre-reflective intersubjectivity (e.g., Reddy, Citation2008; Stern, Citation1985; Trevarthen, Citation1979; Trevarthen & Aitken, Citation2003). This form of intersubjectivity and interpersonal relatedness has been proposed to be the central basis of the development of social understanding and social relations, probably due to immediate sensation or affect sharing (e.g., Hobson, Citation1993; Markova & Legerstee, Citation2006).
A related question is, whether this processing is different from the perception of an inanimate object being touched. Answering these questions would deepen our knowledge on the neurocognitive basis of the animate-inanimate distinction early in ontogeny (e.g., Rakison & Poulin-Dubois, Citation2001). Furthermore, it would also demonstrate that infants not only mirror others’ actions, but also relate to others’ sensory experiences that is a central prerequisite for the development of empathy and social understanding that takes place in the first years of life (e.g., Brownell, Citation2013; Davidov, Zahn-Waxler, Roth-Hanania, & Knafo, Citation2013; Eisenberg, Citation2000; Zahn-Waxler, Radke-Yarrow, Wagner, & Chapman, Citation1992). Behavioural studies already support the view that infants can distinguish between animate and inanimate agents (e.g., Woodward, Citation1998). Yet, the psychological basis is theoretically debated (e.g., Legerstee, Citation1992; Rakison & Poulin-Dubois, Citation2001). The present study contributes to this debate by further clarifying the neurocognitive processes underlying this distinction. In particular, the current study examined whether the sensorimotor system reacts differently to animate vs. inanimate targets being touched, thereby investigating an embodied account of early social cognitive development.
It has been repeatedly shown that a decrease in spectral power in the sensorimotor alpha frequency band above central sites provides a direct way of assessing activation in the sensorimotor system (e.g., Caetano et al., Citation2007). Consequently, frequency band analysis has been successfully employed to examine cortical motor and sensory activation, also in infants (e.g., Saby & Marshall, Citation2012). The current study was thus designed to examine whether already infants relate the perception of others’ being touched to their own sensorimotor system. To this end, we presented 1-year-old infants with video sequences showing a human hand being touched versus nontouched by an object. During the presentation, desynchronisation in sensorimotor alpha (7–9 Hz) above centro-parietal sites was assessed. We selected 1-year-old infants for two reasons: firstly, previous work suggested that at least by the end of their first year of life, infants seem to possess a somatotopically organised sensory system (Saby, Metzoff, & Marshall, Citation2015). Secondly, 1-year-olds are clearly able to recognise other humans, and differently process the actions of a human hand (i.e., an animate being) and a claw (i.e., an inanimate being; Cannon & Woodward, Citation2012). As a comparison (cf. Streltsova & McCleery, Citation2014), we included video sequences in which a nonhuman entity (a cylinder) was touched versus not touched. This allowed us to examine in greater detail the extent to which the activation of the sensorimotor system might be specific for humans being touched versus objects being touched. Based on findings that infants have different action expectations concerning humans and nonliving objects (e.g., Rakison & Poulin-Dubois, Citation2001; Woodward, Citation1998), we expected a similar differentiation in their sensorimotor system between a human hand versus an object being touched/not-touched.
Methods
Participants
Thirty-two children (N = 17 female) with a mean age of 365 days (range = 11 months, 10 days–13 months, 0 days) were tested. The participants were healthy full-term infants without any pre- or perinatal complications and were recruited from local birth records by means of an invitation letter to join the study. Participants came from the lower to upper middle class families in an urban area of Munich/Germany. Parents gave written consent after being informed about the procedure. The final sample of the present study consisted of 21 children (N = 10 female) with a mean age of 368 days (range = 11 months, 10 days–13 months, 0 days), and did not differ on sex or age from the children excluded from the study (all p’s > .05).
Materials and procedure
The EEG recording sessions were situated in an electrically shielded, sound-attenuated chamber with a 19-inch computer monitor placed 100 cm in front of the participants. During EEG recording, infants sat quietly on their mother’s lap, and mothers were instructed not to talk to infants during the EEG recording.
Infants were presented with video clips in .avi format with a total length of 2700 ms each. Adapting previous work with adults, in the human condition, a right (respectively left) palm and forearm of a female actor could be seen from an egocentric point of view (cf. Streltsova & McCleery, Citation2014). In the nonhuman condition, a cylinder from either a right or left side orientation was presented. In the touch condition, an object (i.e., a peacock feather, a brush, or a ruler) entered from the top of the screen after 300 ms. It approached either the arm or the cylinder, and touched it after approximately 1000 ms for approximately 1300 ms. In the nontouch condition, the video clips involved the same objects (i.e., feather, brush, ruler) approaching the arm of the actor or the cylinder, but without touching it. The three same objects were used in all four conditions (human-touch, human nontouch, nonhuman touch, nonhuman nontouch). We included three objects to guarantee that possible sensorimotor alpha effects are not object-specific (cf. Streltsova & McCleery, Citation2014). The order of the four video types and the objects approaching the target was randomised across subjects. Each video was repeated six times for each object; three times with the arm (respectively cylinder) oriented from the right, and three times from the left, resulting in 72 trials in total. The experimenter started a trial by pressing a button the moment the infant looked at the screen. In case infants lost interest in the videos, brightly colored short video clips were presented as attention grabbers. This is an established procedure that allows to keep the infant’s visual attention and to yield minimal eye and motor movements (e.g., Mundy et al., Citation2000; Paulus et al., Citation2014). Infants were videotaped during this procedure to be able to control for attentional distraction and movements. Videos were synchronized with the incoming EEG data, which enabled us to review the recordings frame by frame while leafing through the acquired data.
Electrophysiological recordings
EEG was registered with Cz reference via BrainAmp amplifier (Brain Products, Gilching, Germany) and active Ag/AgCl electrodes (ActiCap, Brain Products, Germany). The band-pass filter settings were 0.016–100 Hz and the sampling rate was 500 Hz. Impedance of all electrodes were kept below 10 kΩ (see also Coll, Bird, Catmur, & Press, Citation2015; Paulus, Kühn-Popp, Licata, Sodian, & Meinhardt, Citation2013; Saby et al., Citation2015). Thirty-two sites (Fp1, Fp2, Fpz, F3, F4, F7, F8, F9, F10, Fz, FC1, FC2, FC5, FC6, C3, C4, T7, T8, TP9, TP10, CP1, CP2, CP5, CP6, P3, P4, P7, P8, Pz, O1, O2, Oz) were placed according to the International 10/20 System at locations commonly used in infants EEG research (see Mundy et al., Citation2000). Fp1 and Fp2 were used to obtain eye blinks and vertical eye-movements; F9 and F10 allowed to detect horizontal eye-movements.
EEG data analysis
EEG data were examined and analysed using Brain Vision Analyzer (Brain Products, Germany). All channels were re-referenced offline to the linked mastoids (Tp9/Tp10). A digital band pass filter of 1–35 Hz/−24 dB/oct was applied. To obtain a reliable time window of EEG activity in which the mere movement of the approaching object was visible subsequent analysis focused on intervals between 1000 ms until 2250 ms after stimulus onset. During this time window, infants perceived how the object approached the arm (or cylinder), either touched or did not touch them, and moved towards the left or right side of the screen where it disappeared. These stimuli related intervals were segmented into 0.5 s epochs (with 50% overlap) in order to provide the data basis for the subsequent Fast Fourier Transform (FFT). For artefact detection segments were automatically scanned and excluded if EEG amplitude of any channel exceeded ±100 μV, or if the difference of values exceeded 150 μV (interval length: 200 ms). In addition, the segments were visually inspected, for eye-movements, blinks, and (motor) artifacts that were previously undetected. Furthermore, segments in which the infant was not looking at the screen or moved were removed from analyses. Finally, artifact-free EEG segments were extracted through a Hanning window and power spectra were calculated via FFT and expressed as mean square microvolts (µV2).
Visual inspection revealed that all participants had a dominant alpha peak around 8 Hz, so that the frequency range of 7–9 Hz was used for analysis. This is in line with previous work with 12-month-old infants (Stapel, Hunnius, Van Elk, & Bekkering, Citation2010). Infants’ EEG data were included if at least 9 trials per condition met the following criteria: a) attention to the stimuli, b) no limb movements, and c) no EEG artefacts (e.g., eye blinks, electrode drifts). Eleven further participants were tested; ten were excluded after artefact detection, due to insufficient trial number (see data analysis section), one because of alpha power being 3 SD above the group mean. The average number of useable segments after artefact rejection were as follows: in the human touch condition M = 31.14 (SD = 13.86, SE = 3.03), the human nontouch condition M = 24.09 (SD = 13.86, SE = 3.03), the nonhuman touch condition M = 24.86 (SD = 12.39, SE = 2.70), and the nonhuman nontouch condition M = 26.48 (SD = 12.35, SE = 2.70). The human touch condition differed significantly from the human nontouch and the nonhuman touch condition (p’s < .001), and from the nonhuman nontouch condition (p < .05). All other comparisons were nonsignificant (p’s > .618).
Given that the purpose of the study was to investigate sensorimotor alpha during infants’ perception of touch and nontouch, we computed clusters and calculated the mean power of the sensorimotor alpha frequency for centro-parietal electrodes (C3/CP5 respectively C4/CP6). Hemisphere was included as a factor to control for possible differences as found in previous studies (e.g., Marshall, Saby, & Meltzoff, Citation2013). All factors were subjected to a repeated-measures analysis of variance (ANOVA) and, where appropriate, Greenhouse–Geisser corrections for nonsphericity were applied. The corrected p-values and the original degrees of freedom are reported. Significant results are reported only when the factors agent or touch were included and were followed by post-hoc tests if appropriate.
Results
It was the aim of the study to investigate whether the power of the EEG signal in the sensorimotor alpha frequency range differed for the perception of human/nonhuman nontouch versus human/nonhuman touch. The topography can be seen in Data were entered into a 2 (hemisphere: left vs. right) x 2 (target: human vs. nonhuman) x 2 (touch: touch vs. no-touch) repeated-measures ANOVA, with the power of the sensorimotor alpha frequency as dependent variable. A significant two-way interaction between agent and touch was found, F(1,20) = 5.48, p = .030, η2ρ = .215 (see ). Step-down comparisons between the human condition and nonhuman condition separately revealed nonsignificant differences for the nonhuman condition (p = .91), while in the human condition the comparison between touch and nontouch did not reach significance, p = .059, η2ρ = .167. No other main or interaction effects were significant (all p’s > .17).
Figure 1. Topographic voltage maps of mean amplitude differences (touch minus nontouch), as a function of target (human vs. nonhuman).
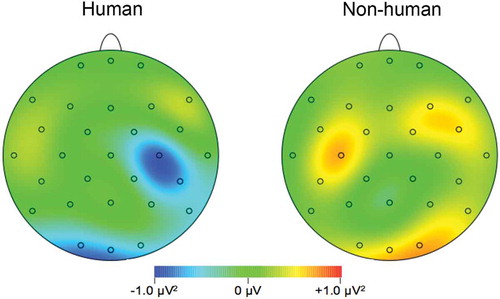
Figure 2. The results of the grand average analysis: on the y-axis, sensorimotor alpha frequency is depicted. The grand averaged EEG power over the sensorimotor alpha power (7–9 Hz) for (a) the touch and (b) the nontouch condition, as a function of target (human vs. nonhuman). Error bars represent standard errors.
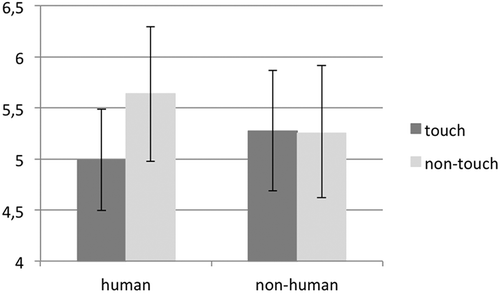
To further explore the differences between sensorimotor alpha frequency, difference scores were calculated by subtracting the nontouch alpha power from the touch alpha power for both the human and nonhuman condition separately. Lower scores indicate lower sensorimotor alpha power for the touch condition. A 2 (agent: human vs. nonhuman) repeated-measures ANOVA with the difference score as dependent variable revealed a significant main effect, F(1,20) = 4.51, p = .046, η2ρ = .184, indicating that a differentiation was made in the sensorimotor system for a human hand versus an object being touched/not-touched.
Discussion
The current study was designed to examine whether already infants relate the perception of others’ being touched to their own sensorimotor system and whether they distinguish between animate and inanimate targets. To this end, we presented 1-year-old infants with video sequences showing a human or nonhuman target being touched versus nontouched by an object. Simultaneously, we assessed desynchronization in sensorimotor alpha (7–9 Hz) above centro-parietal sites. Our results indicate differential cortical reactivity to the touch versus nontouch situations for the human compared to the nonhuman target, suggesting that infants’ sensorimotor system might be involved in the processing of others being or not being touched.
That is, no differences between touch and nontouch could be found in the nonhuman condition. Interestingly, earlier research demonstrated that infants have different action expectations concerning humans and nonliving objects (e.g., Cannon & Woodward, Citation2012; Falck-Ytter et al., Citation2006; Johnson et al., Citation1998; Rakison & Poulin-Dubois, Citation2001; Woodward, Citation1998). Thus, our study extends this line of work to the area of how infants process the sensory experiences of others. Notably, our hypothesis that already 1-year-old infants differentiate between a human hand versus an object being touched/not-touched in their sensorimotor system could be supported: when it comes to the simulation of others’ sensory experiences, observed touch seems to be restricted to other humans. From a developmental point this aspect is noteworthy. Our finding suggests that already 1-year-old infants show differential cortical reactivity to the touch versus nontouch situations for other humans, but not inanimate objects.
In contrast to the inanimate stimuli, we found a tendency for differential reactivity to touch versus nontouch events for the human target stimuli, which, despite the significant interaction effect, did not reach significance. Research with adults reported stronger sensorimotor alpha suppression during the human touch condition (e.g., Ebisch et al., Citation2008, Citation2011; Keysers et al., Citation2010; Kuehn et al., Citation2014; Schaefer et al., Citation2006, Citation2009), and present findings suggest that already from an early age on, infants might be able to relate the perception of others’ being touched to their own sensorimotor system. The weak effects could partially be explained by the use of video stimuli: infants were presented with movie clips instead of real-life events, as this setup guaranteed a controlled timeline. Although this methodological decision allowed us to control for movement variability and other experimenter effects, it could be problematic given that research has shown decreased performance when presented with video clips compared to live performance (e.g., Wellman, Cross, & Watson, Citation2001). For example, 6-months-olds produce different motor activity when viewing reaching actions live compared to viewing the same movements on video (Shimada & Hiraki, Citation2006). Given that we found a significant interaction in sensorimotor alpha even with video stimuli, one could speculate that evidence for an involvement of the sensorimotor system in the processing of others being touched or not touched might be stronger when using live events.
It is possible that already infants share body representations between self and other (e.g., De Vignemont, Citation2014), which are suggested to play an important role for emotional and social understanding (Bolognini et al., Citation2014). These similarities are especially interesting given the increased understanding of others’ subjective feelings (Davidov et al., Citation2013): although the understanding of others’ feelings has yet to be fully developed (Wellman et al., Citation2001), the supposed underlying mechanisms could already start to develop in the course of the second year of life. These findings contribute to recent work that highlights the neurocognitive basis of early social understanding and the early ontogeny of the social brain (e.g., Cowell & Decety, Citation2015; Grossmann, Citation2015). Notwithstanding this strength, further research is necessary to confirm our findings before drawing strong theoretical conclusions.
The current work also relates to research on the processing of touch in infants. Work has shown that the sensitivity for pleasant touch emerges early in human development (e.g., Bremner, Mareschal, Lloyd-Fox, & Spence, Citation2008; Fairhurst, Löken, & Grossman, Citation2014). Our study extends this line of work by demonstrating that infants also process the touch of others. Combining these two lines of investigation, it would be interesting to see when in development children start to understand that also others might perceive touch as being something pleasant. Additionally, it would be interesting for future research to explore the developmental aspects of viewing someone being touched, and viewing someone touching a target. Embodied theories would predict some similarity in the activation patterns. Yet, one would also hypothesize subtle differences. Indeed, research in adults demonstrated that while observing someone being touched mostly activates the primary somatosensory cortex (e.g., Pihko, Nangini, Jousmäki, & Hari, Citation2010), observing another person performing an action, such as touching someone or something, mainly activates the premotor cortex (e.g., Rizzolatti, Fadiga, Gallese, & Fogassi, Citation1996). It would be worthwhile to explore commonalities and differences of these two systems in greater detail.
With the present design, we followed earlier research conducted with adults (e.g., Bufalari, Aprile, Avenanti, Di Russo, Aglioti, Citation2007; Streltsova & McCleery, Citation2014), which found evidence for embodied simulation of touch by comparing different observation conditions. Yet, differences between conditions do not necessarily preclude activation simply due to representation of an arm in general being touched, and not necessarily another person’s arm. However, similar paradigms have been used in earlier research (e.g., Cannon & Woodward, Citation2012; Woodward, Citation1998), and as the arm was an adult arm, the clothing was different from the infant, and the infant did not feel any touch in the touch-conditions (i.e., when the arm in the video was touched), we have good reasons to believe that infants did not think that it was their arm being touched. Nevertheless, it would be interesting to examine in greater detail whether the activation of the sensorimotor areas represents a rather unspecific embodied activation or whether it represents a specific mirroring of the observed experiences of the other. To clarify this issue, future studies could compare the activation of observing someone being touched with the activation that follows from being touched oneself. We leave it to future research to address this issue in greater detail. Moreover, a limitation of the current study setup concerns the fact that infants sat on their mother’s lab during the experiment. This was done to keep infants in an attentive state and calm enough to participate in the experiment. Yet, one could argue that additional artefacts for electrophysiological recordings could be introduced with such a setup. Thus, future research could, for example, place infants in an appropriate chair to avoid direct bodily contact with the mother.
In sum, we showed that by 12 months, infants show different cortical reactivity to human compared to nonhuman targets being touched versus nontouched by an object. Our study is, to the best of our knowledge, one of the first to explore the ontogenetic origins of shared sensory experiences. Therefore, the present findings are a first step to expand our knowledge on the neurocognitive basis of both the animate-inanimate distinction in early infancy, and the development of early intersubjectivity.
Acknowledgments
We would like to thank Ruth Hofrichter, Sara Murphy, and the entire developmental psychology EEG lab for their help with this study, as well as Nike R. H. Tsalas for her helpful comments on earlier versions of the manuscript. Furthermore, we thank all infants and their parents for their participation in this project.
References
- Bolognini, N., Rossetti, A., Fusaro, M., Vallar, G., & Miniussi, C. (2014). Sharing social touch in the primary somatosensory cortex. Current Biology, 24, 1513–1517. doi:10.1016/j.cub.2014.05.025
- Bremner, A. J., Mareschal, D., Lloyd-Fox, S., & Spence, C. (2008). Spatial localization of touch in the first year of life: Early influence of a visual spatial code and the development of remapping across changes in limb position. Journal of Experimental Psychology: General, 137, 149–162. doi:10.1037/0096-3445.137.1.149
- Brownell, C. (2013). Early development of prosocial behavior: Current perspectives. Infancy, 18, 1–9. doi:10.1111/infa.12004
- Buccino, G., Lui, F., Canessa, N., Patteri, I., Lagravinese, G., Benuzzi, F., … Rizzolatti, G. (2004). Neural circuits involved in the recognition of actions performed by non-conspecifics: An fMRI study. Journal of Cognitive Neuroscience, 16, 114–126. doi:10.1162/089892904322755601
- Bufalari, I., Aprile, T., Avenanti, A., Di Russo, F., & Aglioti, S. M. (2007). Empathy for pain and touch in the human somatosensory cortex. Cerebral Cortex, 17, 2553–2561. doi:10.1093/cercor/bhl161
- Caetano, G., Jousmäki, V., & Hari, R. (2007). Actor’s and observer’s primary motor cortices stabilize similarly after seen or heard motor actions. Proceedings of the National Academy of Sciences, 104, 9058–9062. doi:10.1073/pnas.0702453104
- Cannon, E. N., & Woodward, A. L. (2012). Infants generate goal-based action predictions. Developmental Science, 15, 292–298. doi:10.1111/j.1467-7687.2011.01127.x
- Caramazza, A., & Shelton, J. R. (1998). Domain-specific knowledge systems in the brain: The animate-inanimate distinction. Journal of Cognitive Neuroscience, 10, 1–34.
- Casartelli, L., & Molteni, M. (2014). Where there is a goal, there is a way: What, why and how pareto-frontal mirror network can mediate imitative behaviours. Neuroscience and Biobehavioral Review, 47, 177–193. doi:10.1016/j.neubiorev.2014.08.004
- Coll, M. P., Bird, G., Catmur, C., & Press, C. (2015). Cross-modal repetition effects in the mu rhythm indicate tactile mirroring during action observation. Cortex, 63, 121–131. doi:10.1016/j.cortex.2014.08.024
- Cowell, J., & Decety, J. (2015). Precursors to morality in development as a complex interplay between neural, socio-environmental, and behavioral facets. Proceedings of the National Academy of Sciences of the USA, 112, 12657–12662. doi:10.1073/pnas.1508832112
- Cuevas, K., & Paulus, M. (2016). Development of action mirroring. British Journal of Developmental Psychology, 34, 1–5. doi:10.1098/rstb.2013.0181
- Davidov, M., Zahn-Waxler, C., Roth-Hanania, R., & Knafo, A. (2013). Concern for others in the first year of life: Theory, evidence, and avenues for research. Child Development Perspectives, 7, 126–131. doi:10.1111/cdep.12028
- De Vignemont, F. (2014). Shared body representations and the “Whose” system. Neuropsychologia, 55, 128–136. doi:10.1016/j.neuropsychologia.2013.08.013
- Decety, J. (2007). A social cognitive neuroscience model of human empathy. In E. Harmon-Jones, & P. Winkielman (Eds.), Social neuroscience: Integrating biological and psychological explanations of social behavior (pp. 246–270). New York, NY: Guilford.
- Ebisch, S. J. H., Ferri, F., Salone, A., Perrucci, M. G., D’Amico, L., Ferro, F. M., … Press, C. (2011). Differential involvement of somatosensory and interoceptive cortices during the observation of affective touch. Journal of Cognitive Neuroscience, 23, 1808–1822. doi:10.1162/jocn.2010.21551
- Ebisch, S. J. H., Perrucci, M. G., Ferretti, A., Del Gratta, C., Romani, G. L., & Gallese, V. (2008). The sense of touch: Embodied simulation in a visuotactile mirroring mechanism for observed animate or inanimate touch. Journal of Cognitive Neuroscience, 20, 1611–1623. doi:10.1162/jocn.2008.20111
- Eisenberg, N. (2000). Emotion, regulation, and moral development. Annual Review in Psychology, 51, 665–697. doi:10.1146/annurev.psych.51.1.665
- Fairhurst, M. T., Löken, L. S., & Grossman, T. (2014). Physiological and behavioral responses reveal human infants’ sensitivity to pleasant touch. Psychological Science, 25, 1124–1131. doi:10.1177/0956797614527114
- Falck-Ytter, T., Gredebäck, G., & Von Hofsten, C. (2006). Infants predict other people’s action goals. Nature Neuroscience, 9, 878–879. doi:10.1038/nn1729
- Fries, P., Reynolds, J. H., Rorie, A. E., & Desimone, R. (2001). Modulation of oscillatory neuronal synchronization by selective visual attention. Science, 291, 1560–1563. doi:10.1126/science.1055465
- Grossmann, T. (2015). The development of social brain functions in infancy. Psychological Bulletin, 141, 1266–1287. doi:10.1037/bul0000002
- Hobson, R. P. (1993). Understanding persons: The role of affect. In S. Baron-Cohen, H. Tager-Flusberg, & D. Cohen (Eds.), Understanding other minds: Perspectives from autism (pp. 204–227). Oxford, UK: Oxford University Press.
- Johnson, S., Slaughter, V., & Carey, S. (1998). Whose gaze will infants follow? The elicitation of gaze-following in 12-month-olds. Developmental Science, 1, 233–238. doi:10.1111/1467-7687.00036
- Keysers, C., Kaas, J. H., & Gazzola, V. (2010). Somatosensation in social perception. Nature Reviews Neuroscience, 11, 417–428. doi:10.1038/nrn2833
- Kuehn, E., Müller, K., Turner, R., & Schütz-Bosback, S. (2014). The functional architecture of S1 during touch observation described with 7 TfMRI. Brain Structure and Function, 219, 119–140. doi:10.1007/s00429-012-0489-z
- Kuhlmeier, V. A., Bloom, P., & Wynn, K. (2004). Do 5-month-old infants see humans as material objects? Cognition, 94, 95–103. doi:10.1016/j.cognition.2004.02.007
- Legerstee, M. (1992). A review of the animate-inanimate distinction in infancy: Implications for models of social and cognitive knowing. Infant and Child Development, 1, 59–67. doi:10.1002/edp.2430010202
- Markova, G., & Legerstee, M. (2006). Contingency, imitation, and affect sharing: Foundations of infants’ social awareness. Developmental Psychology, 42, 132–141. doi:10.1037/0012-1649.42.1.132
- Marshall, P. J., Saby, J. N., & Meltzoff, A. N. (2013). Infant bran responses to object weight: Exploring goal-directed actions and self-experience. Infancy, 18, 942–960. doi:10.1111/infa.12012
- Mundy, P., Card, J., Fox, N. (2000). EEG correlates of the development of infant joint attention skills. Developmental Psychobiology, 36, 325–338.
- Nyström, P., Ljunghammar, T., Rosander, K., & Von Hofsten, C. (2011). Using mu rhythm perturbations to measure mirror neuron activity in infants. Developmental Science, 14, 327–335. doi:10.1016/j.neulet.2012.10.006
- Paulus, M. (2014). How and why do infants imitate? An ideomotor approach to social and imitative learning in infancy (and beyond). Psychonomic Bulletin & Review, 21, 1139–1156. doi: 10.3758/s13423-014-0598-1
- Paulus, M., Hunnius, S., & Bekkering, H. (2013). Neurocognitive mechanisms subserving social learning in infancy: Infants’ neural processing of the effects of others’ actions. Social Cognitive and Affective Neuroscience, 8, 774–779. doi:10.1093/scan/nss065
- Paulus, M., Kühn-Popp, N., Licata, M., Sodian, B., & Meinhardt, J. (2013). Neural correlates of prosocail behavior in infancy: Different neurophysiological mechanisms support the emergence of helping and comforting. NeuroImage, 66, 522–530. doi:10.1016/j.neuroimage.2012.10.041
- Pihko, E., Nangini, C., Jousmäki, V., & Hari, R. (2010). Observing touch activates human primary somatosensory cortex. European Journal of Neuroscience, 31, 1836–1843. doi:10.1111/j.1460-9568.2010.07192.x
- Pineda, J. A. (2005). The functional significance of mu rhythms: Translating “seeing” and “hearing” into “doing”. Brain Research Reviews, 50, 57–68. doi:10.1016/j.brainresrev.2005.04.005
- Quadrelli, E., & Turati, C. (2015). Origins and development of mirroring mechanisms: A neuroconstructivist framework. British Journal of Developmental Psychology, Advance online publication. doi:10.1111/bjdp.12110
- Rakison, D. H., & Poulin-Dubois, D. (2001). Developmental origin of the animate–inanimate distinction. Psychological Bulletin, 127, 209–228. doi:10.1037/0033-2909.127.2.209
- Reddy, V. (2008). How infants know minds. Cambridge, MA: Harvard University Press.
- Reid, V. M., Striano, T., & Iacoboni, M. (2011). Neural correlates of dyadic interaction during infancy. Developmental Cognitive Neuroscience, 1, 124–130. doi:10.1016/j.dcn.2011.01.001
- Rizzolatti, G., & Craighero, L. (2004). The mirror-neuron system. Annual Reviews in Neuroscience, 27, 169–192. doi:10.1146/annurev.neuro.27.070203.144230
- Rizzolatti, G., Fadiga, L., Gallese, V., & Fogassi, L. (1996). Premotor cortex and the recognition of motor actions. Brain Research Cognitive Brain Research, 3, 131–141. doi:10.1016/0926-6410(95)00038-0
- Saby, J. N., & Marshall, P. J. (2012). The utility of EEG band power analysis in the study of infancy and early childhood. Developmental Neuropsychology, 37, 253–273. doi:10.1080/87565641.2011.614663
- Saby, J. N., Marshall, P. J., & Meltzoff, A. N. (2012). Neural correlates of being imitated: An EEG study in preverbal infants. Social Neuroscience, 7, 650–661. doi:10.1080/17470919.2012.691429
- Saby, J. N., Metzoff, A. N., & Marshall, P. J. (2013). Infants’ somatotopic neural responses to seeing human actions: I’ve got you under my skin. Plos ONE, 8, e77905. doi:10.1371/journal.pone.0077905
- Saby, J. N., Metzoff, A. N., & Marshall, P. J. (2015). Neural body maps in human infants: Somatotopic responses to tactile stimulation in 7-month-olds. NeuroImage, 118, 74–78. doi:10.1016/j.neuroimage.2015.05.097
- Schaefer, M., Flor, H., Heinze, H. J., & Rotte, M. (2006). Dynamic modulation of the primary somatosensory cortex during seeing and feeling a touched hand. NeuroImage, 29, 587–592. doi:10.1016/j.neuroimage.2005.07.016
- Schaefer, M., Xu, B., Flor, H., & Cohen, L. G. (2009). Effects of different viewing perspectives on somatosensory activations during observation of touch. Human Brain Mapping, 30, 2722–2730. doi:10.1002/hbm.20701
- Shimada, S., & Hiraki, K. (2006). Infant’s brain responses to live and televised action. NeuroImage, 32, 930–939. doi:10.1016/j.neuroimage.2006.03.044
- Singer, T., Seymour, B., O’Doherty, J., Kaube, H., Dolan, R. J., & Frith, C. D. (2004). Empathy for pain involves the affective but not sensory components of pain. Science, 303, 1157–1162. doi:10.1126/science.1093535
- Stapel, J. C., Hunnius, S., Van Elk, M., & Bekkering, H. (2010). Motor activation during observation of unusual versus ordinary actions in infancy. Social Neuroscience, 5, 451–460. doi:10.1080/17470919.2010.490667
- Stern, D. N. (1985). Affect attunement. In J. D. Call, E. Galenson, & R. L. Tyson (Eds.), Frontiers of infant psychiatry (Vol. 2, pp. 3–14). New York, NY: Basic Books.
- Streltsova, A., & McCleery, J. P. (2014). Neural time-course of the observation of human and non-human object touch. Social, Cognitive and Affective Neuroscience, 9, 333–341. doi:10.1093/scan/nss142
- Trevarthen, C. (1979). Communication and cooperation in early infancy: A description of primary intersubjectivity. In M. Bulkow (Ed.), Before speech: The beginning of interpersonal communication. Cambridge, UK: Cambridge University Press.
- Trevarthen, C., & Aitken, K. J. (2003). Infant intersubjectivity: Research, theory, and clinical applications. Journal of Child Psychology and Psychiatry, 42, 3–48. doi:10.1111/1469-7610.00701
- Turati, C., Natale, E., Bolognini, N., Senna, I., Picozzi, M., Longhi, E., & Cassia, V. M. (2013). The early development of human mirror mechanisms: Evidence from electromyographic recordings at 3 and 6 months. Developmental Science, 16, 793–800. doi:10.1111/desc.12066
- Wellman, H. M., Cross, D., & Watson, J. (2001). Meta-analysis of theory-of-mind development: The truth about false belief. Child Development, 72, 655–684. doi:10.1111/cdev.2001.72.issue-3
- Woodward, A. L. (1998). Infants selectively encode the goal object of an actor’s reach. Cognition, 69, 1–34. doi:10.1016/S0010-0277(98)00058-4
- Zahn-Waxler, C., Radke-Yarrow, M., Wagner, E., & Chapman, M. (1992). Development of concern for others. Developmental Psychology, 28, 126–136. doi:10.1037/0012-1649.28.1.126