Abstract
The contribution of green microalgae has been recognized in the production of useful products such as chemicals, fatty acids, proteins, carotenoids, pigments, polysaccharides, and pharmaceuticals. One of the challenges to the development of profitable bioproduct markets is the design, development, modeling, and evaluation of cost-effective production systems. The photobioreactors for microalgae can be either open or closed systems in large-scale production. In various situations, it has been reported that closed photobioreactors offer many advantages over open systems. These advantages include lower contamination, higher biomass densities, and better process control. Nevertheless, for the scale-up of enclosed tubular photobioreactors, the accumulation of oxygen as a photosynthetic byproduct has been a major limitation because it severely inhibits growth of microalgae. In this study, we use the distinctive feature of liquid perfluorocarbons (PFCs) as a carrier that efficiently removes the accumulated oxygen from algal medium phase in the spinner culture flasks. The results show the growth kinetics of microalgae cultivated by this approach is significantly improved.
INTRODUCTION
The production of unicellular microalgae has long been recognized as having vast commercial potential in the production of useful products such as fine chemicals, fatty acids, proteins, carotenoids, pigments, polysaccharides, pharmaceuticals, and food for all commercially important aquatic animals (e.g., fish, oysters, shrimp). The potential uses of microalgae as a source of fuel gas (e.g., hydrogen, methane) are also under exploration Citation[1-2]. For long-term sojourns in space, microalgal cultures are also likely to be an essential part of regenerative life-support systems Citation[[3]]. The application of microalgae as oxygen generators for waste oxygenation and nutrient control is the most economical process available for waste oxygenation at present Citation[[4]].
Traditionally, microalgae have been cultivated in open channels and ponds where the culture environment (i.e., salinity and pH) is selected to favor algal growth relative to that of contaminating microorganisms. However, many algal species cannot be cultured reliably in these systems. The obvious drawback of open systems is the potential for contamination from competitors, pathogens, and predators. Open cultures have also suffered from a lack of control, including inadequate control of physical and chemical conditions (e.g., turbulence, nutrient concentrations, dissolved gases) that determine the growth rate of the microbial culture. Production of high-value algal products from strains that cannot be maintained in open ponds requires closed systems such as tubular photobioreactors.
In the last decade, increased emphasis has been given to the development of closed systems for the large-scale culture of microalgae Citation[5-6]. A wide variety of systems have been studied, including stirred tank reactors with internal illumination Citation[[7]], hanging bags Citation[[8]], alveolar and flat panels Citation[9-10], and tubular reactors Citation[11-12]. Among these closed systems, tubular photobioreactors are ideal when a high degree of control over the culture conditions is required, as in large-scale culture of microalgae. The main design considerations have been the following: 1) to reduce the light path in order that the algae receive the maximum amount of light; 2) to increase turbulence (Reynolds number); 3) to remove excess photosynthetically produced oxygen; 4) to add carbon dioxide efficiently; and 5) to control the culture temperature.
It has been reported that oxygen levels above air saturation (i.e., dissolved oxygen of 0.2247 mM at 20°C) could inhibit photosynthesis in many algal species even if carbon dioxide concentration is maintained at elevated levels Citation[[13]]. Weissman et al. Citation[[14]] also pointed out that the hypercritical oxygen concentrations are one of the crucial factors limiting the mass culture of microalgae in tubular systems. In order to enhance mass production of microalgae, it is necessary to remove oxygen, which is deleterious to microalgae if its concentration is too high. Among the design challenges mentioned earlier, oxygen removal is the one that has had no satisfactory solutions for overcoming reactor design limitation.
We propose to tackle the problem of high concentrations of oxygen through the use of perfluorocarbons (PFCs). In clinical studies, PFC emulsions, due to their high solubility of respiratory gas (40–60 mL O2/dL and 160–210 mL CO2/dL, respectively) and inert chemical characteristics, have been used to transport and deliver fresh oxygen to animal tissues and remove metabolically accumulated carbon dioxide Citation[[15]]. For photosynthetic microalgae, the carbon in carbon dioxide is their nutrient, and oxygen, if accumulated, is their harmful waste. Therefore, the function of PFCs used with microalgae will be the reverse of its function with animals. That is, PFCs act as the carbon-dioxide-releasing and oxygen-carrying vehicles ().
Figure 1. The application of PFCs on gas exchange systems for human and algae. A) PFCs carry oxygen from lungs to tissues, while carbon dioxide released from cells is transported to the lung for ventilation by PFCs; B) The function of PFCs in the human system is reversed in the algal photosynthetic system.
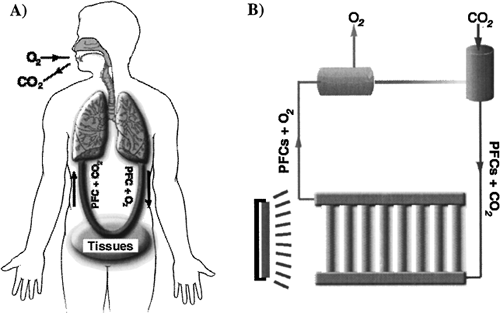
The purpose of this study is to evaluate the beneficial effects of PFC liquids on algal growth in specially designed spinner flasks. Based on this preliminary investigation, we can further apply PFCs to enclosed photobioreactors for mass algal cultivation. The commercially interesting species of microalgae, Chlorella vulgaris, was employed for this investigation.
MATERIALS AND METHODS
Cells
The C. vulgaris was obtained from Algae Culture Collection (University of Texas, Austin, TX) and maintained in TAP media. The TAP media—consisting of distilled water with 1 mL glacial acetic acid, 2 M Tris pH 7.5, 7.5 mM NH4Cl, 0.5 mM MgSO4 · 7H2O, 0.325 mM CaCl2 · 2H2O, 0.62 mM K2HPO4, 0.39 mM KH2PO4, 100 μM H3BO3, 10 μM MnCl2 · 4H2O, 2 μM Na2MoO4 · 2H2O, 1 μM NaVO3, 0.5 μM ZnSO4, 0.1 μM CuCl2 · 2 H2O, and 0.05 μM CoCl2 · 6 H2O—was autoclaved for 30 min at 121°C (118 KPa steam pressure). Culture was maintained in shake flasks on an orbital shaker (VWR Scientific Product, San Dimas, CA) with the speed of 200 rpm at 25 ± 1°C under cool light fluorescent tubes with intensity of 70 μE/m2/s.
PFC
FC-77 (a mixture of perfluoroalkanes and perfluorocylic ether), purchased from 3M Company (St. Paul, MN), has the following properties: density 1.73 g/mL, molecular weight 415 g/mol, and boiling point 97°C. Its oxygen dissolution capacity is 56 mL of gas/100 mL of solvent. This solution was sterilized by filtration via 0.2-μm membrane filter.
Oxygen Inhibition
To determine the oxygen inhibition effect, the C. vulgaris culture was taken from stock culture into 150-mL spinner flasks which contained 50 mL of TAP media. The inoculation density was 1 × 105 cells/mL. The culture was maintained on the spinner table with the speed of 25 rpm, which generates negligible shear stresses on algae, at 25 ± 1°C under 150 μE/m2/s light intensity. Various concentrations of oxygen gas (20, 30, 60, and 80%) balanced with nitrogen were introduced to the culture medium phase in the spinner flasks. Cell concentrations were determined by a hemocytometer at selected times.
Reduction of Oxygen Inhibition by PFC
The C. vulgaris was cultivated in spinner flasks to determine the reduction of oxygen in algae culture by PFC. Since the PFC, with specific gravity around 1.8, is heavier than water and immiscible with culture medium, it will remain in the bottom half of the spinner flask. The main reason for using spinner flasks rather than shaker flasks is that the FC-77 phase is prone to be disturbed by the orbital shaker, whereas the spinner flasks provide a very stable interface and homogeneous mixing. Cells were cultured under the same conditions as the oxygen inhibition experiment. To create a high oxygen concentration similar to oxygen generated and accumulated by algal photosynthesis, a 60% oxygen and 40% nitrogen gas mixture was continuously supplied to the culture medium phase of the spinner flask. The PFC liquid, containing no oxygen, which was purged out by nitrogen gas in the PFC reservoir, was also continuously pumped into the PFC region in the spinner flask through a tube with extremely low gas permeability. gives the schematic illustration of the experimental design and operation.
Figure 2. Experiment designed for investigating the oxygen-removing capability of PFCs. The microalgae were cultured in spinner flasks at 25 rpm. Flask 1 is the positive control; flask 2 is the algae cultured in presence of FC-77; flask 3 is the negative control, which has 60% oxygen continuously pumped in; and flask 4 has the FC-77 remove oxygen pumped into the culture medium. The recycled FC-77 was pumped back to the flask from the reservoir, which was continuously purged by N2.
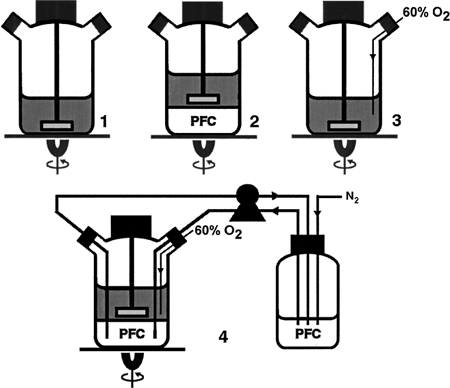
RESULTS AND DISCUSSION
shows the growth kinetics of C. vulgaris under various oxygen concentrations. The maximum cell concentration and growth rate are decreased incrementally with oxygen concentration. The results show that, above 30% oxygen concentration, the growth of C. vulgaris was highly inhibited. Under 80% oxygen concentration (close to a supercritical oxygen environment), the growth of C. vulgaris was totally blocked. This observation is consistent with the results reported by Weissman et al. Citation[[14]].
shows the growth kinetics of C. vulgaris over nine days. The FC-77 can remove oxygen from the culture flask and improve maximum cell concentration of C. vulgaris 10 times from 3.9 × 105 to 4.8 × 106 cells/mL, thus reducing the inhibition problem caused by oxygen.
Figure 4. Cell growth kinetics of C. vulgaris. 1) Positive control; 2) algae cultured in presence of FC-77; 3) negative control, which has 60% oxygen continuously pumped in; and 4) oxygen pumped into the flask was removed by FC-77, which was recycled into the culture medium.
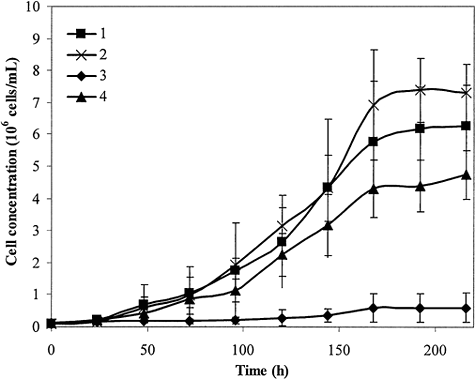
The results indicate that the PFC liquid phase is able to remove the oxygen supplied into the spinner flask culture medium phase, thus abating the inhibition problem caused by the accumulation of high oxygen concentration. Comparing to the growth of algae under 60% oxygen, high algal cell concentration was obtained when the recycled FC-77 was pumped back to the spinner flask from the reservoir, which was continuously purged by N2.
It is also observed that FC-77 has negative effects on neither algal growth nor physiology. As a matter of fact, FC-77 can improve the growth kinetics of C. vulgaris. Since PFCs are nearly inert chemicals, FC-77 should not be able to provide any positive factor for stimulating algal growth. One possible explanation is that FC-77 can extract the toxic compounds or wastes from the algal culture through the immisible interface because of its hydrophobic feature. Wardrop et al. Citation[[16]] has reported that perfluorodecalin can decrease the accumulation of toxic compounds in their plant culture system. The other explanation is that the high accumulation of oxygen (> 20%) in the medium phase of spinner flask, caused by the photosynthesis of microalgae, can be ameliorated by FC-77, simply because FC-77, with its high oxygen-solubility features, can decrease oxygen concentration in the medium phase of culture flask to the suitable oxygen concentration (< 20%) for the algal growth.
Since PFCs are immisible with culture medium, it is necessary to make emulsified PFC microparticles for the removal of oxygen in enclosed tubular photobioreactors. These PFC-containing particles will act as both the sink for the oxygen and the source for the carbon dioxide. As algae in the reactor consume carbon dioxide for photosynthesis, the local concentration of carbon dioxide near the algae surface falls and carbon dioxide then diffuses from the PFC microparticles towards the algae. The delivery and transport of oxygen are just the reverse of this process.
CONCLUSION
To guarantee a successful cultivation of microalgae in closed culture systems, the excess oxygen must be removed from the system. In this study, we purged high oxygen concentration accumulated within the algal culture medium by using a PFC liquid. By this approach, the algal cells cultivated within the spinner flask where PFC is being recycled can obtain much higher cell concentrations than those without PFC. This investigation showed that the development of large-scale enclosed tubular photobioreactors with perfluorocarbon-based internal gas exchange could possible make commercial applications of microalgal biotechnology.
REFERENCES
- Rao K. K., Hall D. O. Hydrogen Production by Cyanobacteria: Potential, Problems and Prospects. J. Marine Biotechnol. 1996; 4: 10–15
- Minowa T., Sawayama S. A. A Novel Microalgal System for Energy Production with Nitrogen Cycling. Fuel 1999; 78: 1213–1215
- Olson R. L., Oleson M. W., Slavin T. J. CELSS for Advanced Manned Mission. Hort. Science 1988; 23: 275–286
- Micro-Algal Biotechnology, M. A. Borowitzka, L. J. Borowitzka. Cambridge University Press, Cambridge 1988
- Lee Y. K. Enclosed Bioreactor for the Mass Cultivation of Photosynthetic Microorganisms: The Future Trend. Trends Biotechnol. 1986; 4: 186–189
- Borowitzka M. A. Closed Algal Photobioreactors: Design Considerations for Large-Scale Systems. J. Marine Biotechnol. 1996; 4: 185–191
- Hilaly A. K., Karim M. N., Guyre D. Optimization of an Industrial Microalgae Fermentation. Biotechnol. Bioeng. 1994; 43: 314–320
- Cohen E., Arad S. A. Closed System for Outdoor Cultivation of Porphyridium. Biomass 1989; 18: 59–67
- Tredici M. R., Materassi R. From Open Ponds to Vertical Alvelar Panels: The Italian Experience in the Development of Reactors for the Mass Cultivation of Phototrophic Microorganisms. J. Appl. Phycol. 1992; 4: 221–231
- Hu Q., Guterman H., Richmond A. A Flat Inclined Modular Photobioreactor for Outdoor Mass Cultivation of Photoautotrophs. Biotechnol. Bioeng. 1996; 51: 51–60
- Pirt S. L., Lee Y. K., Walach M. R., Pirt M. W., Balyuzi H. H., Bazin M. J. A Tubular Bioreactor for Photosynthetic Production of Biomass from Carbon Dioxide: Design and Performance. J. Chem. Technol. Biotechnol. 1983; 33B: 35–58
- Richmond A., Boussiba S., Vonshak A., Kopel R. A New Tubular Reactor for Mass Production of Microalgae Outdoors. J. Appl. Phycol. 1993; 5: 327–332
- Aiba S. Growth Kinetics of Photosynthetic Microorganisms. Adv. Biochem. Eng. 1982; 23: 85–156
- Weissman J. C., Goebel R. P., Benemann J. R. Photobioreactor Design: Mixing, Carbon Utilization, and Oxygen Accumulation. Biotechnol. Bioeng. 1988; 31: 336–344
- Lowe K. C. Perfluorochemical Respiratory Gas Carriers: Applications in Medicine and Biotechnology. Sci. Progr. 1997; 80: 169–193
- Wardrop J., Davey M. R., Marchant R., Power J. B., Lowe K. C. Carbon Dioxide Gassed Fluorocarbon Enhances Micropropagation of Rose (Rosa chinesis JacQ.). Plant Cell Rep. 1997; 17: 17–21