Abstract
To elucidate the cytotoxic induction mechanisms of the hydrophilic HEMA, the comparative cytotoxic activities of HEMA and the hydrophobic monomers TEGDMA and MMA were studied, using erythrocytes, gingival fibroblasts and a salivary gland carcinoma cell line. Also, the gel-to-fluid phase transition properties (i.e. temperature, Tm; cooperativity, H/HHW; enthalpy, ΔH) of dipalmitoylphosphatidylcholine (DPPC) and DPPC/cholesterol (CS) liposomes (as a model for biological membranes) induced by methacrylates were investigated, using differential scanning calorimetry (DSC). In addition, the methacrylate-chemical-shifts in DPPC liposomes were assayed using NMR spectroscopy. Both the hemolytic and cytotoxic activity declined in the order: TEGDMA> HEMA>MMA. The changes in Tm increased in the order: HEMA <MMA<TEGDMA. The H/HHW declined in the order of MMA >>TEGDMA, while in contrast, that of HEMA was slightly increased without changes in the ΔH. The DSC changes in DPPC/CS liposomes with HEMA were the largest of those recorded. The cytotoxicity of HEMA may be induced by the hydrophobic interaction derived from the molecular association of OH groups of HEMA and, in addition, by the preferential interaction with CS.
INTRODUCTION
HEMA and triethyleneglycol dimethacrylate (TEGDMA) are well-known components of composite, dentin bonding materials and the resin-modified/light-polymerized type of glass-ionomer cements. The cytotoxicity of HEMA and/or TEGDMA, against immortal or primary cells such as mouse fibroblasts Citation[[1]], VERO cells Citation[[2]], pulp fibroblasts Citation[[3]], HeLa S3 cells Citation[[4]] and permanent 3T3 and three primary human fibroblasts Citation[[5]] have been reported, indicating that the cytotoxicity of HEMA was lower than TEGDMA. In addition, the potency of HEMA to induce inflammation and allergic reaction of HEMA have been recently reported Citation[6-12]. The cytotoxic induction mechanism of the amphipathic monomer, HEMA, has, however, not been sufficiently investigated.
Methacrylates such as HEMA and TEGDMA will predominantly exist in the lipid phase or in the aqueous phase in biological systems, depending on their hydrophobicity. Partitioning of monomers into the lipid bilayer in living cells causes changes to the phase of the transition properties (i.e. enthalpy and entropy) of membrane lipids. We previously employed lecithin/cholesterol liposomes as a biological membrane model system for investigating the interaction between the lipid-bilayer and methacrylate monomers Citation[[13]]. Also, we previously investigated the phase-transition properties (i.e. phase-transition temperature, Tm; enthalpy, H; height/half height-width, H/HHW) of dipalmitoylphosphatidylcholine (DPPC) liposomes induced by methacrylates, using differential scanning calorimetry (DSC) and, in addition, investigated the NMR chemical shifts of methacrylates in DPPC liposomes, using NMR spectroscopy Citation[14-17]. It was demonstrated that approximately 40% of TEGDMA was impregnated into DPPC liposomes, and its migration rate was higher than that of MMA, indicating that TEGDMA with ethylene glycol groups appeared to be activated on liposomes as a surfactant-like reagent Citation[14-16]. Also, MMA showed a lower cytotoxicity than that of n-butyl (meth)acrylates, possibly due to the effect of the hydrophobic interaction with liposomes Citation[[17]].
It was previously demonstrated that methacrylates contained in resins were bioactive in low concentrations as well as being cytotoxic and that concentrations below 1.5 mM of HEMA altered the proliferation of monocytes after the long exposure Citation[[10]]. HEMA is a hydrophilic monomer, therefore, it is suggested to exhibit smaller intraction with biological membranes, due to the small hydophobic interaction.
In the present study, to elucidate the cytotoxic induction mechanisms of HEMA, we investigated the comparative cytotoxic activity of HEMA, TEGDMA and methyl methacrylate (MMA) against dog erythrocytes, human gingival fibroblasts (HGF) and a human salivary gland carcinoma cell line (HSG). Also, we investigated the DSC changes in the Tm, H and H/HHW of DPPC or DPPC/cholesterol liposomes in the presence of HEMA, TEGDMA or MMA and, in addition, studied the changes in NMR chemical shifts of HEMA, TEGDMA or MMA in DPPC liposomes. A possible link between the biological activities and changes in DPPC or DPPC/CS liposomes as a model for biological membranes in the presence of HEMA is discussed in comparison with those of TEGDMA and MMA.
MATERIALS AND METHODS
The following chemicals and reagents were obtained from the indicated companies. HEMA, TEGDMA, MMA (Tokyo Kasei Chem.Co., Tokyo, Japan); L-α-dipalmitoyl phosphatidylcholine (DPPC), cholesterol (CS), (Sigma Chemical Co., USA); deuterium oxide (D2O), 3-(trimethylsilyl)propionic acid sodium salts (TMSPA) (Merck, Darmstadt, Germany); MEM (Minimum essential medium Eagle), α-MEM (Minimum essential medium alpha modification), fetal bovine serum (FBS), new born calf serum (NBCS) (JRH Biosciences, Lenexa, KS, USA); Cell Titer 96 AQueous solution MTT [3-(4,5-dimethylthiazo-2-yl)-2,5-diphenyl tetrazolium bromide] (Promega., Madison, WI, USA). Methacrylate monomers were used after purification.
Hemolysis Study
Dog erythrocytes were isolated from blood anticoagulated with EDTA by centrifugation (× 1,000) for 10 min. The plasma and buffy coat were then removed and erythrocytes were washed three times with 0.1 M phosphate buffer solution (PBS), pH 7.4 before being dispersed in PBS. An appropriate concentration of HEMA, MMA or TEGDMA was prepared in PBS. One mL of the solution obtained was placed in each assay tube and 1 ml of the cell suspension containing 0.5% erythrocytes was added to it and incubated at 37°C for 1 h. After incubation, the tubes were centrifuged at 2,000 rpm for 10 min and the absorbance of the supernatant at a wave length of 545 nm was measured. The hemolysis percentage (H%) for each specimen was calculated using the following equation. H%=(Aspecimen-A0)/(A100-A0)×100, where A100 and A0 are the absorbance (545 nm) of 100% hemolysis and non-hemolyzed cells, respectively. Hemolyzing for 100% had taken place by freezing and thawing.
Cytotoxicity Assay
The reactivity of methacrylate monomers to human gingival fibroblasts (HGF) can be extrapolated to the clinical situation around subgingival restoration. Also, the choice of cell line and HGF or HSG cells used in continuous culture permitted an accurate evaluation of the changes in cytotoxic activity of the dental materials Citation[[18]]. HSG and HGF cells were prepared in the same manner as previously reported Citation[[18]]. These cells were inoculated into 96-well plates at a density of 4 × 103 cells/well in 0.1 ml of MEM supplemented with 10% NBCS or α-MEM supplemented with 10% FBS and cultured at 37°C for 48 h. The medium was replaced by serum-free medium 1 h before the assay. TEGDMA, HEMA and MMA (10−5 to 1 M) dissolved in ethanol solution was added to the cells, providing a final concentration of 10−7 to 10−2 M. The final ethanol concentration was 1%. After incubation for 48 h, the viable cell number was estimated using the MTT method. In brief, 20 or 30 μl of Cell Titer 96 AQueous solution was added into each well. After incubation for 3 h at 37°C, the absorbance at 492 nm was determined. The relative viable cell number was defined as the ratio (percent) of the absorbance in the experimental well to that of the control well (no test compound, but 1% ethanol). A dose-response curve of relative cell viability (% of control) was plotted to delineate the concentrations of the monomers that depressed MTT-formazan production of 50% (IC50 value).
Liposome Preparation
An appropriate amount of DPPC mixed in chloroform solution were subjected to a vacuum and then dispersed by vortexing in a 0.07 M phosphate buffer solution (PBS) at pH 6.8. DPPC and the CS mixture (DPPC: CS = 10:1, or 4:1, molar ratio) were added to a chloroform solution and were subjected to a vacuum and then dispersed by vortexing in a 0.07 M PBS at pH 6.8. The DPPC or DPPC / CS mixture in PBS was sonicated under a nitrogen atmosphere for 5 min.
DSC Studies
An aliquot sample (20 μl) together with DPPC and the indicated concentration of monomer were put into a DSC specimen container. The specimen was allowed to equilibrate for 14 h at 5°C and then, the specimen was scanned in a sealed calorimetric container on a DSC-Rigaku calorimeter (Rigaku Denki Co. Ltd., Tokyo, Japan) at a heating rate of 5°C min−1 with a range setting of 0.5 mcal s−1. The instrument was calibrated with indium as a standard. Enthalpy (H) and height/half-height width (H/HHW) were calculated from the area under the DSC curves and DSC peak, respectively Citation[[10]]. DSC in the DPPC/CS liposomes was carried out in a similar manner to that of DPPC liposomes. The concentration of DPPC in DPPC liposomes or DPPC/CS liposomes was 0.070 M in PBS.
NMR Studies
1H-NMR spectra were measured at 30°C and 52°C using a JEOL JNM-GX-270 spectrometer (JEOL, Tokyo, Japan) at 270 MHz. TEGDMA/DPPC (1:1 molar ratio), HEMA/DPPC (1:1) and MMA/DPPC (1:1) liposomes were prepared in D2O in a similar manner to that previously reported (10). DPPC was 10% w/v in D2O. The chemical shifts of HEMA, TEGDMA, MMA and DPPC are reported in ppm downfield from the external standard, TMSPA. The accuracy of the chemical shifts was 0.01 ppm in present study.
RESULTS
Hemolytic Activity
Results are shown in . Hemolytic activity of TEGDMA and MMA were rapidly enhanced at concentrations of 1 mM and 10 mM, respectively. The activity of HEMA at low concentrations below 10 mM was greater than that of MMA, whereas the converse was true above 10 mM. The concentration required to produce 20% hemolysis (H20) of TEGDMA, HEMA and MMA was approximately 1.0, 4.5 and 10.0 mM, respectively.
Figure 1. Hemolysis (%) of HEMA, TEGDMA and MMA as a function of concentration. TEGDMA (▵), MMA (□), and HEMA (○). HEMA (
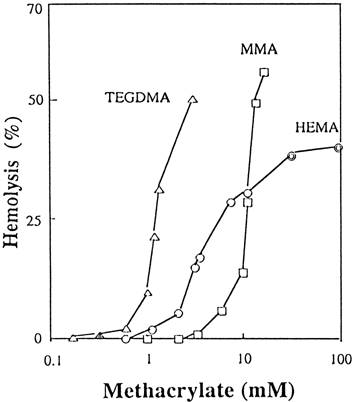
Cytotoxic Activity
Cytotoxic activities of TEGDMA, HEMA and MMA against HSG and HGF cells are shown in a and b, respectively. The IC50 of HEMA and TEGDMA against HSG cells was approximately 2.10 mM and 0.33 mM, respectively. That of HEMA and TEGDMA against HGF cells was approximately 5.60 mM and 0.33 mM, respectively. That of MMA against both cells was >10 mM. The cytotoxic activity declined in the order: TEGDMA>HEMA>MMA.
Figure 2. The viability (%) of HEMA, TEGDMA and MMA against HGF cells (a) or HSG cells (b) as a function of concentration. HEMA (▪), MMA (•), and TEGDMA (▴). Values are presented as the mean ±SD of 8 different determinations. The IC50 of TEGDMA against both HSG and HGF was approximately 0.33 mM. That of HEMA against HSG and HGF was approximately 2.10 and 5.60 mM, respectively. The % viability curves of the methacrylate response, the responses of HEMA, TEGDMA and MMA were significantly different (p<0.01) in both HSG (a) and HGF (b) cells as determined by two-way analysis of variance (ANOVA).
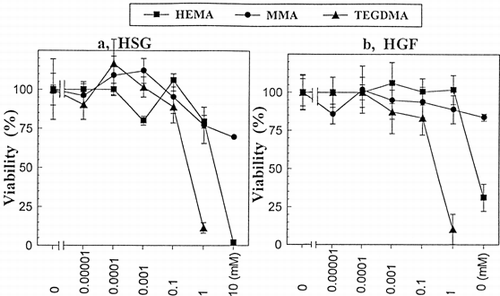
Phase Transition Properties of DPPC/CS Liposomes
shows the DSC curves of DPPC/CS liposomes induced by methacrylates and findings from the presentation in are summarized in . DPPC liposomes with increasing CS were markedly broadened (A a, b, and c). Conversely, DSC curves of DPPC/CS (4:1, molar ratio) by MEOH (B d-f) or that of DPPC/CS (10:1) by HEMA (C g-i) were markedly enlarged with increasing MEOH or HEMA. The H of 50% MEOH (7.8 kcal/mol) (f) or 50 mM HEMA (8.9 kcal/mol)(i) approached the enthalpy of the DPPC liposomes without additives (
H 8.7 kcal/mol) (a). The Tm of the latter shifted to a lower temperature of 35°C. However, 140 mM MMA did not show any changes in the DSC curves, despite its large concentration (k). TEGDMA at 140 mM (m), but not at 50 mM (i), showed a Tm shift of 2.5°C to a lower temperature with little change in enthalpy. It was clear that HEMA had a larger effect on DPPC/CS liposomes than TEGDMA or MMA.
Figure 3. Typical DSC phase-transition profiles for dipalmitoyl phosphatidylcholine/cholesterol (DPPC/CS) liposomes doped with methanol (MEOH), 2-hydroxyethyl methacrylate (HEMA), triethylene glycol dimethacrylate (TEDGMA), or methyl methacrylate (MMA) in phosphate buffer, pH 6.8. (A) Effect of CS on DPPC liposomes: CS was added to DPPC in chloroform and then, DPPC/CS liposomes were prepared as described in the text. (a) DPPC liposomes without CS (control): a main phase-transition peak at 40.5°C and *pretransition peak at 33.5°C, (b) DPPC/CS (10:1, molar ratio): abolishing of the pretransition peak, and (c) DPPC/CS (4:1): broadened DSC; (B) Effect of MEOH on DPPC/CS (4:1) liposomes: (d) 12.5% MEOH, (e) 25% MEOH, and (f) 50% MEOH; (C) Effect of HEMA on DPPC/CS (10:1) liposomes in 12.5% MEOH: (g) 5 mM HEMA, (h) 25 mM HEMA, and (i) 50 mM HEMA; (D) Effect of MMA or TEGDMA on DPPC/CS (10:1) liposomes in 12.5% MEOH; (j) DPPC/CS (10:1) liposomes, (k) 140 mM MMA, (l) 50 mM TEGDMA, and (m) 140 mM TEGDMA.
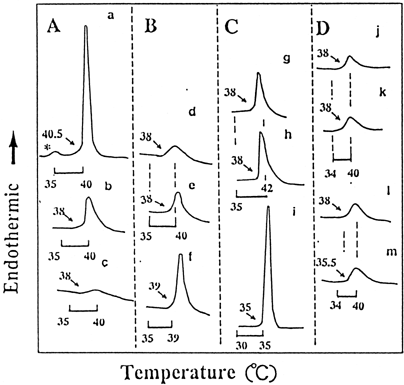
Figure 4. Phase-transition temperature (°C)(A) and height/half height-width (H/HHW) (B) of DPPC liposomes with HEMA (▵) or MMA (○) and TEGDMA/DPPC liposomes (□), as a function of the concentration of monomers in phosphate buffer solution at pH 6.8. An appropriate concentration of HEMA or MMA was added to DPPC liposomes. TEGDMA/DPPC liposomes were prepared in a similar manner to that of DPPC/CS liposomes in Materials and Methods due to the high hydrophobicity of TEGDMA. Values presented are the mean of two different determinations. Computational error for Tm or H/HHW was ≦ ca 5%. The relative H/HHW values = (H/HHW of specimen/ H/HHW of control) × 100.
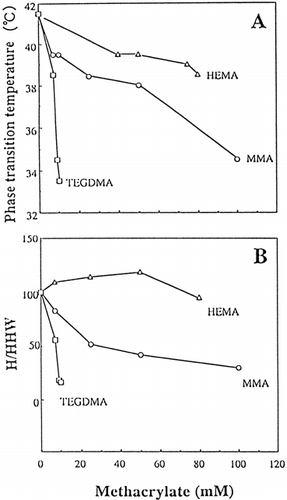
Table I. Phase Transition Temperature (Tm) and Enthalpy (ΔH) of DPPC, DPPC/CS Liposomes with or without Methanol (MEOH), HEMA, or TEGDMA in Phosphate Buffer Solution at pH 6.8#
DSC Phase-Transition Properties of DPPC Liposomes
The Tm (A) and H/HHW (B) as a function of the concentration of TEGDMA, HEMA or MMA are also shown in A and B, respectively. A typical DSC curve of DPPC liposomes without any additives (control) are shown in a. DSC curves of DPPC liposomes with TEGDMA or MMA broadened dose-dependently with the phase-transition-temperature' shifting to a lower temperature (data not shown). This variation was exhibited by the Tm and H/HHW values (). The Tm was decreased in the order of HEMA> MMA>> TEGDMA. Also, the H/HHW was decreased in the order of HEMA>MMA >> TEGDMA. HEMA showed a slightly increased H/HHW value. Methacrylates showed few changes in H, even though the concentrations were increased (data not shown).
Changes in NMR Chemical-Shift
1H-NMR spectra of TEGDMA/DPPC, HEMA/DPPC or DPPC liposomes are shown in . The summarized results of the NMR chemical-shift are shown in . The broadened proton signals of TEGDMA in TEGDMA/DPPC liposomes at 30°C were shifted to a higher field by 0.1–0.3 ppm, indicating a large shielding. The proton signal of α-methyl of TEGDMA at 30°C was split into two peaks that showed a higher and lower field signal (, TEGDMA/DPPC, No. 1), indicating that the portion of α-methyl of TEGMA was located in the outside and inside of the liposomes. Whereas, no signals of TEGDMA or of choline methyl (N+Me3), acyl chains [(CH2)14] and the terminal methyl group in DPPC were observed or were markedly broadened at undetectable levels at 52°C, due to saturation, which indicated the strong interaction between TEGDMA and the acyl chains of DPPC liposomes. However, the signals of HEMA much more broadened at 52°C than at 30°C and did not shift to a higher field (). This suggests that HEMA were significantly located in the surface region of DPPC liposomes. The broadened proton signals of N+Me3, (CH2)14 and the terminal methyl derived from DPPC appeared at 52°C in the HEMA/DPPC or DPPC liposomes, indicating that the liposomes existed in a liquid phase at this temperature. The phase transition temperature of DPPC liposomes was approximately 40.5°C and above this temperature the liposomes were in a liquid phase. In MMA/DPPC liposomes, the proton signals were increasingly but slightly shifted to a higher field, indicating the impregnation of the CH2=C group into the lipid bilayer.
Figure 5. 1H-NMR spectra of HEMA/DPPC (1:1 molar ratio), TEGDMA/DPPC (1:1, molar ratio), and DPPC liposomes in deuterium oxide (D2O), as a function of temperature at 30°C and 52°C (A) and the chemical structure of triethylene glycol dimethacrylate (TEGDMA), 2-hydroxyethyl methacrylate (HEMA), and L-α-dipalmitoyl phosphatidylcholine (DPPC), and their numbering system (B). HEMA/DPPC: The indicated concentration of HEMA was added to DPPC liposomes; TEGDMA/DPPC: TEGDMA/DPPC liposomes were prepared in a similar manner to that of DPPC/CS liposomes in Materials and Methods; DPPC: DPPC liposomes were prepared as described in Materials and Methods. After preparation, the specimens were allowed to equilibrate for 6 h at 5°C before they were measured. X, y, and z is are the terminal methyl, acyl chains and choline methyl group of DPPC, respectively(B). TMSPA was used as an external reference in deuterium oxide and the chemical shifts are ppm downfield from TMSPA. DHO, deuterium oxide contained very small quantities of H2O.
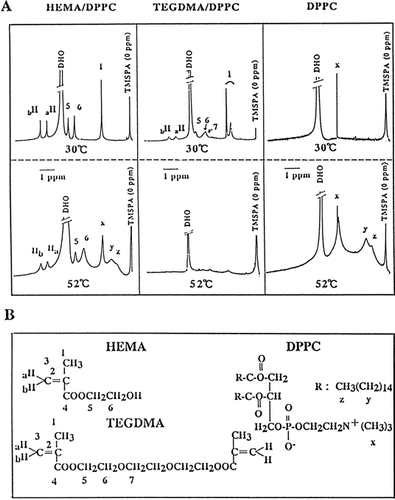
Table II. The Chemical Shift Differences (Footnote#
DISCUSSION
HEMA, which was first introduced in 1973 Citation[[19]], is widely used as a resin adhesive system or in the resin-modified/light-polymerized types of glass-ionomer cement in dentistry Citation[20-21]. Recently, HEMA was reported to have cytotoxic and/or allergic activity Citation[1-9]. Since HEMA contacts wet dentin surfaces as dentin bonding agents or light-polymerized types of glass-ionomer cement in dentistry, this could have a direct influence either on its toxic effects or by the induced inflammation reaction. However, when applied to dentin, HEMA was previouly reported to rapidly diffuse dentin towards the pulp below the concentrations caused acute toxicity Citation[[12]]. However, TEGDMA and HEMA were previously reported to be released from the composite restoration into the pulp chamber as late as 50 days following polymerization Citation[[22]]. The IC50 of HEMA, TEGDMA or MMA for HGF and HSG cells in the present study were similar to those against various cells previously reported Citation[1-5] and the toxicity rank was in the order: TEGDMA>HEMA>MMA.
The hemolytic activity of TEGDMA and MMA was rapidly enhanced at 1 mM and 10 mM, respectively (). Above 10 mM, HEMA did not reach the H50, possibly due to the denaturation of hemoglobin caused by the primary alcohol action of HEMA to proteins.
The cytotoxic activity of methacrylate monomers was dependent on the hydrophobicity (Log P, octanol/water partition coefficient)Citation[[4]]. However, the penetration of drugs to chicken blood cells was previously reported to be more highly correlated to (Log P) × (water solubility) than Log P Citation[[23]]. In consideration of the above findings, despite HEMA showing a lower cytotoxicity and lower Log P than TEGDMA, HEMA could have a higher cytotoxic activity than TEGDMA, because HEMA is capable of maintaining high concentrations in biological systems due to its high water solubility. However, despite HEMA being a hydrophilic monomer, HEMA at relatively lower concentrations showed H/HHW >100, indicating that this may have been governed by the hydrophobic environment caused by the molecular association due to the OH group of HEMA (R—O—H…O(H)—R) in an aqueous medium. It was suggested from this that the molecular association due to the OH group of HEMA is possibly converted, in part, to diethylene glycol dimethacrylate-like substances via its dehydration in hydrophobic acyl chains of phospholipids The H/HHW was >100, which is due to the high cooperativity in the phase transition of liposomes, as previously reported in hydrophobic monomer systems such as butyl methacrylate Citation[[17]]. Also, the role of the ethyl alcohol in the transition to the interdigited state was reported to stabilize the interdigitated state by binding to the lipid surface and interacting with the hydrophobic terminal methyl groups which are near the surface of the lipid Citation[[24]]. Thus, HEMA which is a primary alcohol, was thought to show hydrophobic interactive behaviors at lower concentrations. Sub-lethal damage of lower concentrations of HEMA to macrophages has been previously reported Citation[[10]], suggesting the hydrophobic interaction between HEMA and cell membranes.
One aspect of the adverse effect of degradation products of HEMA (i.e. HEMA is decomposed into both methacrylic acid and ethylene glycol by hydrolysis, and furthermore, methacrylic acid produces formaldehyde due to oxidation) was previously reported, suggesting that HEMA impairs formation of phosphatidic acid (PA), possibly by acylation of ethylene glycol released by hydrolysis of the HEMA Citation[[11]]. We previously reported that methacrylic acid showed less interaction with DPPC liposomes at pH 7.0, due to the effects of both the ionization and the small hydrophobicity, therefore, ethylene glycol may be activated on liposomes as a surfactant-like agents Citation[[14]].
CS can be found in many biological membranes at very high concentrations, up 50 mol% in the case of human erythrocytes. Thus, phospholipid liposomes containing cholesterol are the best model for studying the interaction of methacrylate monomers with biological membranes. Our DSC study showed that CS broadened the gel-to-liquid crystalline phase transition of phospholipid liposomes as well as decreasing the calorimetrically observed transition enthalpy. This finding agreed with previously reported observations Citation[25-26]. Conversely, the increasing HEMA sharply enlarged the DSC curves of DPPC/CS liposomes, resulting in increased H (), possibly due to the extraction of CS in DPPC/CS liposomes.
TEGDMA showed a large decrease in the Tm and H/HHW of DPPC liposomes and large NMR changes, indicating that TEGDMA markedly affects the lipid bilayer by inducing an increase in membrane fluidity, disorder and mobility of the lipid bilayer, which can modulate the function of membrane proteins. TEGDMA, but not MMA, at a higher concentration of 140 mM lowered Tm by 2°C, even though the H of DPPC/CS liposomes was not affected. This indicates that the entropy (ΔS) of TEGDMA increased more than that of MMA in the system, as calculated from the folmula: ΔS=ΔH×Tm−1 (, i.e. the ΔS of MMA (k) and TEGDMA (m) were 14.8 and 16.5 cal/k × mol, respectively). HEMA showed larger ΔS than TEGDMA (, i.e. the ΔS of HEMA at 5, 25 and 50 mM were 17.7, 22.5 and 28.9 cal/k × mol, respectively), indicating that the membrane structure of DPPC×CS liposomes was markedly affected by HEMA. The preferential target of HEMA is probably cholesterol in the biomembranes.
In the modern composite resin system, Bis-GMA and UDMA are widely used as components of monomers, as well as TEGDMA. NMR spectroscopic findings showed that the movement of Bis-GMA in DPPC/CS liposomes was significantly more restricted than that of TEGDMA Citation[[16]]. Also, the NMR proton signals of UDMA in DPPC/CS liposomes shifted to a higher field (i.e. shielding), while those of TEGDMA did not change greatly in DPPC/CS liposomes Citation[[16]]. This indicated that Bis-GMA showed higher interaction with acyl chains in DPPC than TEGDMA or UDMA. A number of cytotoxic studies have reported that Bis-GMA had a larger cytotoxicity than that of TEGDMA or UDMA Citation[[5]], Citation[[27]]. It was suggested that as one aspect of the cytotoxicity induction, the migration of small lipid-soluble methacrylates into the lipid bilayer plays an important role in the cytotoxic induction.
Thus, these findings of the hydrophobicity of methacrylates, and in addition, their partitioning into the lipid bilayer, is a rationale for estimating the cytotoxic induction because the toxic activity of methacrylates is initially derived from their interaction with membranes in living cells. Therefore, monitoring of the biological activity might reasonably expect to show a correlation to the present DSC and NMR spectroscopic study of liposomes.
ACKNOWLEDGMENTS
This study was supported, in part, by a Grant-in-Aid from the Ministry of Education, Science, Sports and Culture of Japan (S. Fujisawa: No. 10671842).
REFERENCES
- Ratanasathien S., Wataha J. C., Hanks C. T., Dennison J. B. Cytotoxic interactive effects of dentin bonding components on mouse fibroblasts. J Dent Res 1995; 74: 1602–160
- Bruce G. R., McDonald N. J., Sydiskis R. J. Cytotoxicity of retrofill materials. J Endodont 1993; 19: 228–292
- Li N., Miao X., Takakuwa M., Sato K., Sato A. Effect of dental material HEMA monomer on human dental pulp cells. Art Cells Blood Subs Immob Biotech 1999; 27: 85–90
- Yoshii E. Cytotoxic effects of acrylates and methacrylates: Relationships of monomer structures and cytotoxicity. Biomed Mater Res 1997; 37: 517–524
- Geyrtsen W., Lehmann F., Spahl W., Leyhausen G. Cytotoxicity of 35 dental resin composite monomers/additives in permanent 3T3 and three human primary fibroblast cultures. J Biomed Mater Res 1998; 41: 474–480
- Kanerva L., Alanko K. Allergic contact dermatitis from 2-hydroxyethyl methacrylate in an adhesive on an electrosurgical earthing plate. Eur J Dermatol 1998; 8: 521–524
- Katsuno K., Manabe A., Kurihara A., Itoh K., Hisamitsu H., Wakumoto S., Yoshida T. The adverse effect of commercial dentin-bonding systems on the skin of guinea pig. J Oral Rehabil 1998; 25: 180–184
- Rustemeyer T., De Groot J., Von Blomberg B. M.E., Frosch P. J., Scheper R. Cross-reactivity patterns of contact-sensitizing methacrylates. Toxicol Appl Pharmacol 1998; 148: 83–90
- Andersson T., Bruze M., Bjorkner B. In vitro testing of the protection of gloves against acrylates in dentin-bonding systems on known contact allergy to acrylates. Contact Dermatitis 1999; 41: 254–259
- Bouillaguet S., Wataha J. C., Virgillito M., Gonzalez L., Rakich D. R., Meyer J-M. Effect of sub-lethal concentration of HEMA (2-hydroxyethyl methacrylate) on THP-1 human monocyte-macrophages, in vitro. Dent Mater 2000; 16: 213–217
- Schuster G. S., Caughman G. B., Rueggeberg F. A. Changes in cell phospholipid metabolism in vitro in the presence of HEMA and its degradation products. Dent Mater 2000; 16: 297–303
- Bouillaguet S., Wataha J. C., Hanks C. T., Ciucchi B., Holz J. In vitro cytotoxicity and dentin permeability of HEMA. J Endodont 1996; 22: 244–248
- Fujisawa S., Kadoma Y., Masuhara E. Action of drugs, detergents, and monomers on liposomes. J Dent Res 1982; 61: 1206–1210
- Fujisawa S., Kadoma Y., Komoda Y. 1H and 13C NMR studies of the interaction of eugenol, phenol, and triethyleneglycol dimethacrylate with phospholipid liposomes as a model system for odontoblast membranes. J Dent Res 1988; 67: 1438–1441
- Fujisawa S., Kadoma Y., Komoda Y. Nuclear magnetic resonance spectroscopic studies of the interaction of methyl methacrylate and ethylene dimethacrylate with phosphatidylcholine liposomes as a model for biomembranes. Biomaterials 1989; 10: 51–55
- Fujisawa S., Kadoma Y., Komoda Y. Changes in 1H-NMR chemical shifts of Bis-GMA and its related methacrylates induced by their interaction with phosphatidylcholine/cholesterol liposomes. Dent Mater J 1991; 10: 121–127
- Fujisawa S., Atsumi T., Kadoma Y. Cytotoxicity of methyl methacrylate (MMA) and related compounds and their interaction with dipalmitoyl phosphatidylcholine (DPPC) liposomes as a model for biomembranes. Oral Dis. 2000; 6: 215–221
- Fujisawa S., Kashiwagi T., Atsumi T., Iwakura I., Ueha T., Hibino Y., Yokoe I. Application of bis-eugenol to a zinc oxide eugenol cement. J Dent 1999; 27: 291–295
- Masuhara E. Uber die Entwicklung eines am Zahnschmelz haftfaehigen Kunststoffes und seine spezielle klinische Anwendung. Deutscher Zahnaerzte-Kalender. Carl Hanser Verlag. 1973; 58–70
- Nakabayashi N., Takarada T. Effect of HEMA on bonding to dentin. Dent Mater 1992; 8: 125–130
- Nakamura K., Hayakawa T., Tomita T., Yamazaki M. Effect of the application of dentin primers and a dentin bonding agent on the adhesion between the resin-modified glass-ionomer cement and dentin. Dent Mater 1998; 14: 281–286
- Gerzina T. M., Hume W. R. Effect of hydrostatic pressure on the diffusion of monomers through dentin in vitro. J Dent Res 1995; 74: 369–373
- Suzuoki Z., Suzuoki T. The concentrative uptake of thiamine alkyl disulfides by rabbit of chick blood cells. J Biochem(Tokyo) 1953; 40: 11–20
- Rowe E. S. Thermodynamic reversibility of phase transitions. Specific effects of alcohols on phosphatidylcholines. Biochim Biophys Acta 1985; 813: 321–330
- Xiang T. X., Anderson B. D. Phase structures of binary lipid bilayers as revealed bypermeability of small molecules. Biochim Biophys Acta 1998; 1370: 64–76
- Estep T. N., Mountcastle D. B., Biltonen R. I., Thompson T. E. Studies on the anomalous thermotropic behavior of aqueous dispersions of dipalmitoylphosphatidylcholine-cholesterol mixtures. Biochemistry 1978; 17: 1984–1989
- Babichi H., Tipton D. A. In vitro cytotoxicity of bisphenol A to human gingival epithelial S-G cells. In vitro Mol Toxicol 1999; 12: 233–244