Abstract
A cross-linked hemoglobin membrane has been created with discerning permeability between dissolved hemoglobin and small molecules. Such a membrane could be used to enclose a sphere of hemoglobin solution thereby allowing the entire “cell” to transport oxygen. The hemoglobin membrane was cross-linked on a polycarbonate support; the mechanical support was necessary for diffusion experiments in this study and would not be used during any sphere preparation. A 30% methemoglobin solution in phosphate buffer was used to fill the pores of the 10 μm polycarbonate support, then cross-linked with a homobifunctional cross-linking agent. The cross-linked hemoglobin within the support was evaluated for hemoglobin and benzoic acid permeability in a side-by-side diffusion cell. Disuccinimidyl glutarate, disuccinimidyl suberate and disuccinimidyl tartrate were used as cross-linking agents. Disuccinimidyl glutarate, 4.65 mM, created a hemoglobin-impermeable membrane after cross-linking for 20 minutes, reducing the concentration to 0.46 mM required a cross-linking time to 60 minutes. Benzoic acid, representing a typical small molecule, was capable of diffusing through the disuccinimidyl glutarate cross-linked hemoglobin membrane at 87.2% of its diffusion through buffer.
INTRODUCTION
The need for an artificial blood substitute continues to grow, especially because of the risk of contracting viral infections such as HIV or hepatitis C in today's society Citation[[1]]. Encapsulating hemoglobin has been explored since the 1950's Citation[[2]] with the idea that the formation of a microcapsule would more closely resemble an artificial red blood cell, as well as have a longer circulation half-life and better oxygen releasing capabilities then a hemoglobin solution. The microcapsule membrane material has been the main focus of encapsulation and and has included synthetics such as nylon and silicone rubber, lipid bilayers and cross-linked protein Citation[[3]]. Chang tried to create a microcapsule with a cross-linked protein membrane Citation[[4]]. Hemoglobin microdroplets were created through emulsification followed by reaction with glutaraldehyde to create a cross-linked protein membrane. The small size of the droplets as well as the rapid reaction of glutaraldehyde resulted in the entire microcapsule becoming cross-linked, forming polyhemoglobin. Davis et al. also created a microcapsule that consisted of a hemoglobin droplet with a glutaraldehyde cross-linked hemoglobin membrane Citation[[5]]. They concluded that the glutaraldehyde may have harmed the hemoglobin as well as penetrated the microcapsule and polymerized some or all of the hemoglobin solution inside. Eisman and Schnaare proposed creating a similar microcapsule, which again consisted of a hemoglobin droplet with a cross-linked hemoglobin membrane Citation[[6]]. In this study lipophilic cross-linkers, such as disuccinimidyl glutarate and terephthaldicarboxaldehyde, were selected in order to limit cross-linking to the interface of the hemoglobin droplet, allowing hemoglobin solution to remain inside the sphere. Overall, a hemoglobin cross-linked microcapsule was formed, although the cross-linked hemoglobin membrane was permeable to the hemoglobin solution within the droplet.
Glutaraldehyde has been widely used in order to cross-link hemoglobin microspheres. The resulting microspheres have become completely polymerized thereby not allowing any hemoglobin solution to remain inside the sphere. Levy et al. created microdroplets through emulsification and then in turn cross-linked the outside of the droplet with several acyldichlorides Citation[[7]]. They also studied the stability of the hemoglobin microcapsules after lyophilization and concluded that upon reconstitution, the microcapsules regained their spherical shape but the hemoglobin inside the sphere diffused out.
The aim of this study is to prepare a cross-linked hemoglobin membrane, which is impermeable to hemoglobin yet permeable to small molecules such as oxygen. The objective is to use homobifunctional lipophilic cross-linkers, N-hydroxysuccinimidyl esters (NHS ester), of varying spacer arm length, in order to create a hemoglobin cross-linked membrane, which is impermeable to dissolved hemoglobin.
MATERIALS AND METHODS
Materials
Freeze-dried bovine hemoglobin crystals (MW 64,500) were obtained from ICN Biomedicals, Inc.; benzoic acid from Aldrich Chemical Company, Inc.; disuccinimidyl glutarate, disuccinimidyl suberate and disuccinimidyl tartrate from Pierce; potassium phosphate monobasic, sodium phosphate and glutaraldehyde (25%) from Fisher Scientific. Polycarbonate membranes (5, 10, 14 μm) were from Poretics®. All other materials were reagent grade or better and were used as received from the manufacturer.
Preparation of Methemoglobin Solution
The 30% hemoglobin solution was prepared by weighing 30.0 g freezedried bovine hemoglobin. To a 250 mL beaker, 50 mL of phosphate buffer pH 6.8 was added along with a magnetic stir bar. Hemoglobin crystals were added in very small amounts, a small spatula full, and stirred until dissolved. This was repeated until all hemoglobin was added and dissolved. Any froth formed by stirring was allowed to dissipate, the solution transferred to a 100 mL volumetric flask and sufficient phosphate buffer added to make 100 mL.
Cross-Linking Reagents
Solutions of disuccinimidyl glutarate (DSG), disuccinimidyl suberate (DSS) and disuccinimidyl tartrate (DST) were prepared in buffer saturated o-xylene. Concentrations used were 4.65 and 0.46 mm for DSG, 4.65 mM for DSS and a saturated solution of DST.
Preparation of Cross-Linked Hemoglobin Membrane
The polycarbonate support was laid for 30 seconds on the surface of the 30% hemoglobin solution in order to fill the pores from one side only. The loaded support was then carefully removed from the hemoglobin solution with forceps and the excess hemoglobin solution was allowed to trickle off the support. The loaded support was then laid, hemoglobin side up, over a vessel containing the cross-linking solution for the desired amount of time. The cell was covered during cross-linking in order to limit evaporation. After cross-linking, the polycarbonate support whose pores filled with cross-linked hemoglobin membrane was removed with a forceps and the entire assembly rinsed in 1 M tris buffer in order to quench the cross-linking reaction. The assembly was next rinsed in phosphate buffer solution and then loaded into a side-by-side diffusion cell for evaluation.
Side-by-Side Diffusion
A side-by-side diffusion cell was used in order to measure permeability through the cross-linked hemoglobin membrane. The apparatus consists of two 125 mL Erlenmeyer flasks, donor and receiver, each with a side arm where a membrane and an o-ring are clamped into place securing the two flasks together. The donor cell is capped with a stopcock adapter while the receiver flask has a simple stopper. The adapter was placed on the donor flask and the donor flask then laid on its side. A magnetic stir bar was added and the flask was filled with diffusing solution, making sure to remove all air. The polycarbonate membrane was then placed on the donor side arm, followed by the o-ring and then the receiver flask was added and clamped in place. While turning the apparatus upright, 100 mL of receiver solution was added to the receiver flask followed by a magnetic stir bar and the stopper. The entire apparatus was placed in a 37°C water bath and the stirring begun. Samples (5 mL) were removed from the receiver flask at preselected timepoints and analyzed using a Hewlett Packard 8452A Diode Array Spectrophotometer at 224 nm for benzoic acid and 405 nm for hemoglobin. Each sample was replaced with warm buffer solution.
RESULTS AND DISCUSSION
Support Membrane Characterization
Benzoic acid, 0.4 mg/mL, was diffusion through polycarbonate supports, having pore sizes of 5, 10 and 14 m, in order to calibrate the supports in the sideby- side diffusion cell. Data were plotted according to Equation 1.D is the diffusion coefficient, ν1 and ν2 are the volume of the donor and receiver chambers respectively, c1 is the concentration of the donor chamber, m is the total mg diffused, A is the total surface area of the pores, h is membrane thickness and t is time.
The slopes obtained in are equivalent to (A/h)eff and are equal to 199, 176 and 349 cm for the 5, 10 and 14 μm polycarbonate supports, respectively. In order to determine the validity of the value of (A/h)eff, the manufacturer's value for total pore area, 0.16 cm2 for all three pore sizes, was used to calculate a value for h, membrane thickness. Overall, membrane thickness for the 5, 10 and 14 μm pore size polycarbonate supports were 8, 9 and 5 μm, respectively. These compare to the manufacturer's specifications of 10, 10 and 6 μm. The slopes determined by plotting the side-by-side diffusion data according to Equation 1 yield realistic values of membrane thickness thereby calibrating the polycarbonate supports.
Figure 1. Benzoic acid in the side-by-side diffusion cell through the 5, 10, and 14 μm polycarbonate supports. (n=3) Error bars represent standard deviation.
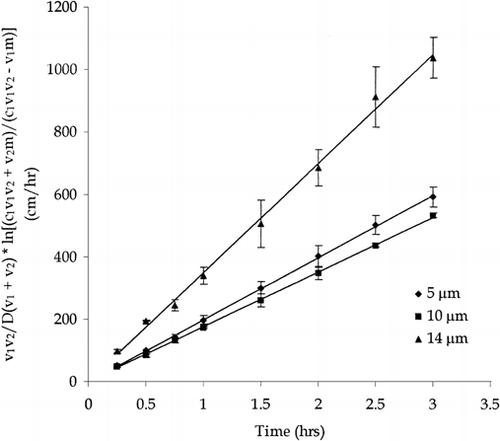
In order to verify this calibration, hemoglobin, 5 mg/mL, was diffused through the empty 10 μm polycarbonate support. The value of (A/h)eff, 176 cm, determined with benzoic acid was used in Equation 1, thus allowing the diffusion coefficient of hemoglobin to be directly determined from the slope. The diffusion coefficient obtained was 13.2 × 10−7 cm2/sec for the 5 mg/mL methemoglobin solution. A 10 mg/mL methemoglobin solution, the nearest concentration found in the literature Citation[[8]], had a diffusion coefficient of 12.0 × 10−7 cm2/sec. Since the diffusion coefficient of hemoglobin increases with decreasing concentration, the diffusion coefficient obtained correlates with the data in the literature.
Cross-Linking
The group of cross-linkers chosen were NHS-esters, which are homobifunctional lipophilic compounds that react with primary amines. Cross-linkers selected were of varying spacer arm length; DST (6.4Å), DSG (7.7Å) and DSS (11.4Å). The difference in spacer arm lengths amongst these cross-linkers places them in different classes, namely intramolecular and intermolecular cross-linkers. Typically a spacer arm less then 8Å is considered to be an intramolecular cross-linker while greater then 8Å is classified as intermolecular. The reaction mechanism for the cross-linking of hemoglobin with the NHS-ester is shown in Reaction .
A relatively quick method of initially assessing the extent of cross-linking was desired instead of lengthy diffusion experiments. Examination of the cross-linked hemoglobin in the support pores microscopically, as well as visually, was selected as a qualitative indicator of the extent of cross-linking and the adequacy of the cross-linking time.
The degree of cross-linking was evaluated as the change in diffusional parameter, A/h, following cross-linking. Knowing the value of (A/h)eff (176 ± 3) for the empty polycarbonate support, a new value of (A/h)eff was determined for the cross-linked hemoglobin. This allowed for the determination of the percent reduction in (A/h)eff due to the presence of cross-linked hemoglobin in the pores. The diffusion coefficient of hemoglobin varies with concentration and therefore was evaluated according to Equation 1 where the slope is equal to (DA/h)eff (cm3/hr). The slope of methemoglobin diffusion, 5 mg/mL, through an empty 10 μm polycarbonate support was determined to be 0.79 ± 0.06 cm3/hr. A new value of (DA/h)eff was determined for the cross-linked hemoglobin membrane, which allowed for the determination of the percent reduction in (DA/h)eff due to cross-linking.
Disuccinimidyl Suberate Cross-Linking
Preliminary microscopic and visual evaluations indicated that 60 minutes of cross-linking resulted in all the pores being filled. Diffusion of hemoglobin through the DSS cross-linked hemoglobin membrane did occur and therefore a hemoglobin-impermeable membrane was not created. Cross-linking time was increased in 20 minute intervals up to 140 minutes. Results, as shown in , indicated that even with the increased cross-linking time, methemoglobin was still able to permeate the DSS cross-linked membrane. Actual values of (DA/h)eff and the corresponding percent reduction are shown in . Cross-linking time was not increased above 140 minutes since the excess methemoglobin solution on the surface of the membrane had begun to dry out and crack at these extended cross-linking times.
Figure 2. Methemoglobin diffusion through the 4.65 mM DSS cross-linked hemoglobin membrane. Error bars represent standard deviation. (n=3)
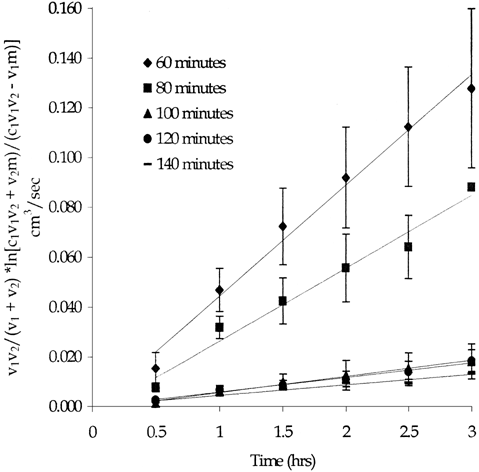
Table 1. Summary of Methemoglobin and Benzoic Acid Diffusion Through the Hemoglobin Membrane Cross-Linked with 4.65 mM DSS in o-Xylene (n=3)
Disuccinimidyl Tartrate Cross-Linking
Due to poor solubility of 4.65 mM DST in o-xylene a saturated solution of DST in o-xylene was used. Diffusion experiments started with 40 minutes of cross-linking which resulted in a 95.9% reduction in (DA/h)eff. Increasing the cross-linking time to 80 minutes resulted in a 98.2% reduction in (DA/h)eff. Cross-linking time was further increased to 120 minutes, which resulted in a 99.1% reduction in (DA/h)eff. Results, as plotted in , show that hemoglobin diffusion still occurred even with 120 minutes of cross-linking with saturated DST. Further increasing the cross-linking time caused the cross-linked hemoglobin membrane to dry out and become cracked.
Disuccinimidyl Glutarate Cross-Linking
Diffusion of methemoglobin through the hemoglobin cross-linked membrane cross-linked with DSG for 60 minutes resulted in no methemoglobin diffusion. In order to determine the minimum cross-linking time required to create a hemoglobin-impermeable membrane, the cross-linking time was decreased until methemoglobin diffusion was obtained. Results showed that decreasing the cross-linking time to 15 minutes was required to obtain a hemoglobin permeable membrane. The 15 minutes of cross-linking with 4.65 mM DSG resulted in a decrease in the value of (DA/h)eff by 99.8%. Further reduction in the cross-linking time to 5 minutes also resulted in a reduction of (DA/h)eff by 99.8%. Thus, even 5 minutes of cross-linking with 4.65 mM DSG resulted in a very high reduction in (DA/h)eff. A cross-linking time of 20 minutes was necessary to create a hemoglobin-impermeable membrane.
Due to the fast reaction time of DSG, the concentration of cross-linker was decreased to 0.46 mM in order to gain more control of the degree of cross-linking. Diffusion of methemoglobin through the 0.46 mM DSG cross-linked membrane, cross-linked for 20 minutes, resulted in a 93.7% reduction in (DA/h)eff. Cross-linking time was again increased in 20 minute increments until a hemoglobin-impermeable membrane was obtained. It was found that 60 minutes of cross-linking time was required to create a hemoglobin-impermeable membrane with the 0.46 mM DSG cross-linking solution. Results of both 4.65 mM and 0.46 mM DSG diffusion experiments are summarized in .
Table 2. Summary of Methemoglobin and Benzoic Acid Diffusion Through the Hemoglobin Cross-Linked Membrane Cross-Linked with Both 4.65 and 0.46 mM DSG in o-Xylene (n=3)
Having created a hemoglobin-impermeable membrane, the diffusion of benzoic acid was essential in showing that even though hemoglobin could not diffuse, small molecules could. Diffusion of benzoic acid was performed through both the 4.65 and 0.46 mM DSG cross-linked hemoglobin membranes. Results, , showed that the diffusion of benzoic acid occurred even when the membrane created was impermeable to hemoglobin. Both the 4.65 and 0.46 mM DSG cross-linked hemoglobin membranes showed this capability.
Spacer Arm Length
The difference in spacer arm lengths between the three cross-linkers used is 6.4, 7.7 and 11.4 Å for DST, DSG and DSS respectively. This places them in two different classes, namely intramolecular, less than 8 Å, and intermolecular, greater than 8 Å. Hemoglobin impermeable membranes were not created with the longest and shortest cross-linkers but rather solely with the cross-linker having a spacer arm length of 7.7 Å. Several explanations for this result include the fact that, due to their different spacer arm lengths, one of the cross-linkers may have had access to an amino group that the other cross-linker was incapable of reaching. Secondly, a 30% (w/v) hemoglobin solution was used for cross-linking and at this high concentration, molecules are close together thereby allowing for intermolecular cross-links to occur, even when using an intramolecular cross-linker (DSG). In contrast, DSS, being primarily an intermolecular cross-linker may have been incapable of forming intramolecular cross-links due to the length of the spacer arm. Lastly, the fact that the spacer arm length of DSG, being 7.7 Å, is borderline in size as being characterized as an intra versus intermolecular cross-linker, DSG may contribute more to intermolecular cross-links then the shorter DST.
CONCLUSION
Cross-linking studies began with using lipophilic N-hydroxysuccinimide esters of varying spacer arm length. The cross-linkers were selected in order to react with the primary amine of the hemoglobin molecule. Cross-linking with disuccinimidyl suberate alone was not capable of creating a hemoglobinimpermeable membrane even after 140 minutes of cross-linking. This was also true when using a saturated solution of the cross-linker disuccinimidyl tartrate. Finally, the cross-linker disuccinimidyl glutarate did produce a hemoglobinimpermeable, hemoglobin cross-linked membrane. Two separate concentrations of DSG, 0.46 and 4.65 mM, were used resulting in hemoglobin-impermeable membranes at cross-linking times of 60 and 20 minutes respectively. Benzoic acid diffusion, being representative of a small molecule, was also studied on both the hemoglobin permeable and impermeable membranes. In both cases benzoic acid was still capable of diffusing through the membranes. Altogether, a hemoglobin cross-linked membrane was created that was impermeable to hemoglobin solution and permeable to benzoic acid.
ACKNOWLEDGMENTS
Gratefully acknowledge the research support of the Mary E. Groff Surgical Medical Research and Education Charitable Trust.
REFERENCES
- Snyder S. R., Welty E. V., Walder R. Y., Williams L. A., Walder J. A. HbXL99α: A hemoglobin derivative that is cross-linked between the α subunits is useful as a blood substitute. Proc. Natl. Acad. Sci. 1987; 84: 7280–7284
- Chang T. M.S. Pharmaceutical and therapeutic applications of artificial cells including microencapsulation. European Journal of Pharmaceutics and Biopharmaceutics 1998; 45: 3–8
- Chang T. M.S. Artificial Cells. Charles C. Thomas, Springfield, IL 1972; 4
- Chang T. M.S. Attempts to find a method to prepare artificial hemoglobin corpuscles. Biomaterials, Artificial Cells and Artificial Organs 1988; 16(1–3)1–19
- Davis T. A., Asher W. J., Wallace H. W. Artificial red cells with crosslinked hemoglobin membranes. Applied Biochemistry and Biotechnology 1984; 10: 123–132
- Eisman J. M., Schnaare R. L. The formation of a crosslinked carboxyhemoglobin membrane at an organic-aqueous interface. Art. Cells, Blood Subs., and Immob. Biotech. 1996; 24(3)185–196
- Keller K. H., Canales E. R., Yum S., II. Tracer and mutual diffusion coefficients of proteins. The Journal of Physical Chemistry 1971; 75(3)379–387
- Levy M. C., Rambourg P., Levy J., Potron G. Microencapsulation IV: Cross-linked hemoglobin microcapsules. Journal of Pharmaceutical Sciences 1982; 71(7)759–762