Abstract
To increase the safety of stroma-free hemoglobin solution (SFH) as an artificial oxygen carrier source, we investigated the effect of heat treatment on virus inactivation in hemoglobin solution. The hemoglobin solution spiked with vesicular stomatitis virus (VSV) was treated at 60°C for 1 hr under either an air or CO atmosphere. VSV was inactivated at >5.8 log10 and >6.0 log10 under the air and CO atmosphere, respectively. Although the methemoglobin rate increased after the heat treatment under the air atmosphere, no methemoglobin formation was observed by the treatment under the CO atmosphere. Isoelectric focusing analysis revealed the denaturation of hemoglobin after the heat treatment under the air, while hemoglobin banding was not altered in the carbonylated condition. Some protein bands other than hemoglobin were weakened or disappeared on SDS-PAGE after the heat treatment under both conditions. In addition, the hemoglobin concentration in the SFH was higher after the heat treatment than before the treatment. These findings indicate that the heat treatment under the CO atmosphere inactivates viruses without hemoglobin denaturation, and hence, this method is a promising approach to prepare a safer SFH as artificial oxygen carriers.
INTRODUCTION
Hemoglobin-based oxygen carriers have been developed using human red blood cells (RBCs). Human RBCs are mainly supplied from blood centers as an outdated RBC component. Despite careful laboratory testing of all donations in blood centers, the risk of viral transmission still exists Citation[[1]]. Therefore, virus inactivation and/or removal methods are strongly required to prepare safe products.
We previously reported that vesicular stomatitis virus (VSV), an enveloped virus, suspended in stroma-free hemoglobin solution (SFH) was inactivated at >6 log10 by 1,9-dimethylmethylene blue phototreatment Citation[[2]]. In addition, human parvovirus B19, which is resistant to chemical and heat treatments, could be removed from SFH by the nanofiltration of BMM-15 with a mean pore size of 15 nm Citation[[3]]. In both methods, methemoglobin formation did not proceed during the treatment. Another approach to inactivate viruses in SFH is heat treatment. Azari et al. reported that many viruses, including human immunodeficiency virus (HIV) and porcine parvovirus, can be inactivated at >7 log10 by heat treatment at 74°C for 90 min Citation[[4]].
Hemoglobin vesicles, which consist of a lipid bilayer containing SFH, have been developed and characterized as an artificial oxygen carrier Citation[5-7]. The process of hemoglobin vesicle preparation includes the heat treatment to increase the purity of hemoglobin in SFH Citation[[8]]. However, hemoglobin is converted easily to methemoglobin by heat treatment under oxygenated conditions. To avoid methemoglobin formation, hemoglobin should be carbonylated before heating Citation[[8]]. The heat treatment also intended to inactivate viruses which may contaminate the SFH. However, it is uncertain that viruses are inactivated by the heat treatment under carbonylated conditions. In this study, we validated the effect of heat treatment on virus inactivation and on hemoglobin behavior in a carbonylated condition.
MATERIALS AND METHODS
Heat Treatment of Hemoglobin Solution
RBCs were washed twice with saline and then hemolyzed by adding an equal volume of H2O. A 100 mL of hemolysate spiked with VSV was carbonylated by flashing CO gas on the surface of the hemolysate in a flask with stirring for 30 min. The hemolysate in the sealed flask was then heated at 60°C for 1 hr in a water bath. Five milliliters of hemolysate were sampled before and after the treatment. After adding 2.5 mL of 1.8% NaCl to the hemolysates, SFHs were prepared as an upper phase by centrifugation at 1,800g for 20 min.
In another experiment, 2.5 mL of RBCs in a glass tube were hemolyzed using 2.5 mL of H2O, bubbled with CO and heated at 60°C for 1 hr. After the addition of 2.5 mL of 1.8% NaCl, the tubes were centrifuged at 1,800g for 20 min. The upper phase (SFH) was transferred to a new tube, and both SFH and the lower phase (stroma) were examined for volume and hemoglobin concentrations.
The above two experiments were carried out in a similar manner without carbonylation.
Measurement of VSV Infectivity
VSV infectivity was determined by cytopathic effect assay using host Vero cells as previously described Citation[[9]]. Briefly, 100 μmL of serially 10-fold diluted VSV samples were added to Vero monolayer cultured with Dulbecco's modified Eagle medium (Gibco BRL, Gaithersburg, MD, USA) containing 10% fetal calf serum in 96-well tissue culture plates. We seeded each dilution in six wells. After the incubation at 37°C in 5% CO2 for 3–5 days, cytopathologic effects were examined under a microscope. VSV infectivity was expressed as a median tissue culture infectious dose (TCID50) calculated by the Reed-Müench method Citation[[10]]. The detection limit of this assay was theoretically found to be 10-0.5 TCID50. We confirmed that hemoglobin in samples did not interfere with VSV infection in our CPE assay.
Measurement of Hemoglobin, Methemoglobin, and Protein Contents
Hemoglobin concentration was measured using a commercial kit based on a cyanmethemoglobin method (Hemoglobin Test-Wako; Wako Junyaku, Osaka, Japan). The rate of methemoglobin was determined by the cyanmethemoglobin method Citation[[11]]. Protein amount was determined by a colorimetric assay (BCA protein assay reagent kit; PIERCE, Rockford, IL, USA).
Electrophoresis
SFH was denatured by 1% sodium dodecyl sulfate (SDS) and 1% 2-mercaptoethanol at 100°C for 5 min and applied on 5–20% SDS-polyacrylamide gel electrophoresis (SDS-PAGE). After electrophoresis, proteins were stained with Coomassie Brilliant Blue R-250 (CBB-R).
Isoelectric focusing was performed using PhastSystem (Pharmacia, Uppsala, Sweden) with PhastGel IEF 5–8 (Pharmacia). After electrophoresis, proteins were fixed with 20% trichloroacetic acid and then stained with CBB-R. Hemoglobin A0 and A2, ferrous, (Sigma, St. Louis, MO, USA) were used as standards. Hemoglobin A0 and A2, ferric, were prepared by incubating ferrous compounds with 1.6% K3Fe(CN)6 for 10 min at room temperature followed by dialyzing against H2O with a 30 kD ultrafilter membrane.
The sample volume applied was adjusted against the hemoglobin concentration of each sample for the electrophoresis.
RESULTS
VSV Inactivation
The hemolysate spiked with VSV was heated at 60°C for 1 hr and SFH was prepared as described in Materials and Methods. Under the air atmosphere, 105.3 TCID50 VSV infectivity was not detected after the treatment (). Similarly, under CO atmosphere, 105.5 TCID50 VSV infectivity was dropped to below the detection limit, indicating that >5.8 log10 and >6.0 log10 inactivation (under air and CO, respectively) was achieved by the treatment ().
Table 1. VSV Infectivity Before and After the Heat Treatment
Methemoglobin Formation and Hemoglobin Recovery
The methemoglobin rate (%metHb) in SFH was significantly increased after the heat treatment under air (A). However, under carbonylated condi-tion, %metHb was slightly decreased, rather than unchanged, by the treatment (A). IEF analysis revealed that banding of hemoglobin A0 and A2, ferrous, weakened and ferric forms became marked after the treatment under air (). However, no change in hemoglobin banding was observed after the treatment under the carbonylated condition ().
Figure 1. Heat treatment of hemoglobin solution. Hemolysate was heated at 60°C for 1 hr under an air (stippled bar, n =3, mean ± SD) or CO (open bar, n = 5, mean ± SD) atmosphere. Methemoglobin rate (A), hemoglobin concentration (B) and protein concentration (C) in SFHs before and after the treatment.
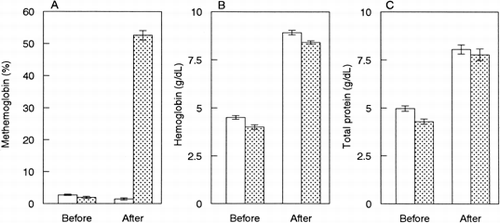
Figure 2. SDS-PAGE and IEF of SFHs. Before (B) and after (A) the heat treatment under the air or CO atmosphere. A0, hemoglobin A0; A2, hemoglobin A2. M.W., molecular weight marker.
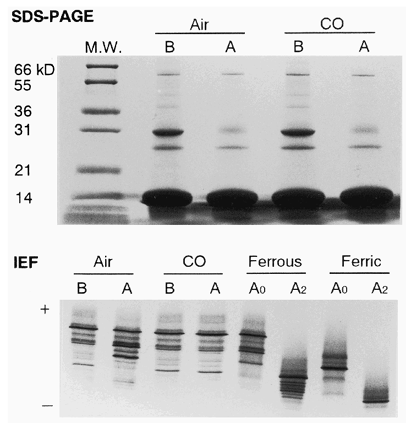
The hemoglobin concentration of SFH was higher after the heat treatment than before the treatment under both the air and CO atmospheres (B). This corresponded to the total protein content (C). SDS-PAGE showed that some protein bands other than hemoglobin were weakened or disappeared after the treatment under both the air and CO atmospheres ().
To clarify the increase in hemoglobin concentration of SFH after the treatment, hemoglobin behavior during the treatment was examined using the tube-closed system as described in Materials and Methods. As shown in A, the hemoglobin concentration in SFH was higher after the heat treatment than before the treatment. The hemoglobin concentration in stroma was much higher than that in SFH for the sample before the treatment whereas hemoglobin concentration was at a similar level between SFH and stroma after the treatment (A). Since the solution volume varied among the samples (B), the total hemoglobin amount was calculated (C). Hemoglobin recovery in SFH was comparatively higher for the sample treated under the air than the other two samples although the total hemoglobin recovery was low.
DISCUSSION
We observed no difference in the effect on virus inactivation by the heat treatment between the air and CO atmospheres. The heat treatment at 60°C was carried out for 10 hrs to prepare SFH based on the findings that human serum albumin has been treated in the plasma fractionation process and on the study which described that 12 hrs treatment did not affect the IEF pattern of hemoglobin Citation[[8]]. The findings presented here showed that more than 6 log10 inactivation of VSV was achieved by the treatment even for 1 hr in the carbonylated condition (). Therefore, 10 hrs treatment would guarantee further inactivation of viruses.
Attenuation of protein banding after the treatment suggested that those proteins were denatured and sedimented to stroma by the centrifugation, resulting in increase of hemoglobin purity of SFH (). However, to avoid hemoglobin oxidation, carbonylation of hemoglobin was required before the treatment ( and A).
The hemoglobin recovery into SFH was 44% by the treatment under the carbonylated condition (C). In our experience, the heat-denatured proteins other than hemoglobin were sedimented, and easily removed by filtration with a prefilter such as a Millistak (LSSA12HL, Millipore Co.), with a pore size of approximately 15 μm. The yield of hemoglobin was greater than 95%, if the sediments were washed a few times with water (data not shown). Under the air condition, the recovery into SFH was high, but low in total hemoglobin (C), suggesting that hemoglobin denaturation occurred during the treatment.
In conclusion, the heat treatment under the carbonylated condition could inactivate VSV by preventing hemoglobin oxidation and increasing its purity in SFH. Due to the risk, albeit very low, of contamination of thermo-stable viruses such as parvovirus B19 and hepatitis A virus, it will be important to combine with other methods such as nanofiltration Citation[[3]] to fully eliminate viruses.
ACKNOWLEDGMENTS
This study was supported, in part, by a Health Science Research Grant (Artificial Blood Project) from the Ministry of Health and Welfare, Japan, and by Grants-in-Aid for Scientific Research from the Japanese Ministry of Education, Science, Sports and Culture.
REFERENCES
- Schreiber G B, Busch M P, Kleinman S H, Korelitz JJ. The risk of transfusion-transmitted viral infections. N Engl J Med 1996; 334: 1685–1690
- Hirayama J, Wagner S J, Gomez C, MacDonald V W, Abe H, Ikeda H, Ikebuchi K, Sekiguchi S. Virus photoinactivation in stroma-free hemoglobin with methylene blue or 1,9-dimethylemethylene blue. Photochem Photobiol 2000; 71: 90–93
- Abe H, Sugawar H, Hirayama J, Ihara H, Kato K, Ikeda H, Ikebuchi K. Removal of parvovirus B19 from hemoglobin solution by nanofiltration. Artif Cells Blood Substit Immobil Biotechnol 2000; 28: 375–383
- Azari M, Ebeling A, Baker R, Burhop K, Camacho T, Estep T, Guzder S, Marshall T, Rohn K, Sarajari R. Validation of the heat treatment step used in the production of diaspirin crosslinked hemoglobin (DCLHb™) for viral inactivation. Artif Cells Blood Substit Immobil Biotechnol 1998; 25: 577–582
- Takeoka S, Sakai H, Terase K, Nishide H, Tsuchida E. Characteristics of Hb-vesicles and encapsulation procedure. Artif Cells Blood Substit Immobil Biotechnol 1994; 22: 861–866
- Sakai H, Hamada K, Takeoka S, Nishide H, Tsuchida E. Physical properties of hemoglobin vesicles as red cell substitutes. Biotechnol Prog 1996; 12: 119–125
- Sakai H, Tomiyama K I, Sou K, Takeoka S, Tsuchida E. Poly(ethylene glycol)-conjugation and deoxygenation enable long-term preservation of hemoglobin-vesicles as oxygen carriers in a liquid state. Bioconjug Chem 2000; 11: 425–432
- Sakai H, Takeoka S, Nishide H, Tsuchida E. Convenient method to purify hemoglobin. Artif Cells Blood Substit Immobil Biotechnol 1994; 22: 651–656
- Hirayama J, Abe H, Ikebuchi K, Horiuchi M, Shinagawa M, Kamo N, Sekiguchi S. Virus inactivation in superoxide dismutase preparations by ultra-violet light irradiation. Biol Pharm Bull 1998; 21: 621–623
- Reed L J, Müench H. A simple method of estimating 50 percent endpoints. Am J Hyg 1938; 27: 493–497
- van Kampen E J, Zijlstra W G. Determination of hemoglobin and its derivatives. Adv Clin Chem 1965; 8: 141–187