Abstract
Hemoglobin based oxygen-carrying solutions (HBOCs) as hemoglobin replacement therapeutics are being tested for clinical use. Some of these products are associated with elevations in both systemic and pulmonary vascular resistances but their effect on the distribution of blood flow to major organs in larger animals have not been extensively described. We tested two formulations of o-raffinose cross-linked human hemoglobin, Hemolink™ (frozen Hemolink™-1 and refrigerated Hemolink™-2) and compared them to Pentaspan® a colloid volume expander in extensive clinical use. Cardiovascular measurements and the distribution of blood flow (radionuclide-labeled microspheres) to the major organs were determined in Beagle dogs (n = 5 per group). After baseline measurements, either Hemolink™-1, or Hemolink™-2, or Pentaspan® was exchange transfused in an isovolemic manner (resulting in hematocrit reduction to approximately 20–25%); measurements were made 30, 60, 120 and 180 min post-exchange. There was no significant difference in cardiac output, mean arterial pressures and systemic or pulmonary vascular resistance after exchange in any of the three groups. Myocardial blood flow increased in all three groups post-exchange but the increase was more sustained in the Hemolink™ groups. Endocardial/epicardial flow ratios were also maintained after exchange in all groups. Thus, Hemolink™ is ideally suited for volume replacement when used in conjunction with acute normovolemic hemodilution because under these circumstances, the adverse hemodynamic effects are alleviated while extra hemoglobin is added to the blood.
INTRODUCTION
The recent development of “commercial” modified hemoglobin-based oxygen carriers (HBOCs) of high purity offer several potential uses including enhancement of blood conservation procedures for surgical practice involving acute normovolemic hemodilution, and replacement of allogeneic red cell transfusions for resuscitation during hemorrhage. HBOC's combine the rheological changes due to dilution of RBCs by conventional volume expanders with the advantage of the added oxygen carrying capacity.Citation[[1]] Thus, they may reduce the requirement for augmented cardiac output and organ blood flow that normally accompany normovolemic hemodilution to maintain systemic and organ oxygen delivery.
Hemolink™ is a HBOC that is currently being investigated in Phase III clinical trials for use as a volume replacement in blood conservation during cardiac surgery. It is derived from approved, outdated human RBCs and is prepared by pasteurization and viral filtration. It is cross-linked with oxidized raffinose, a polyaldehyde derivative of the naturally occurring trisaccharide raffinose. Hemolink™ is >99% hemoglobin Ao, of which approximately 54–62% is oligomerized (molecular weight ≥ 64–500 kDa(2)). The viscosity of Hemolink™ is 1.1 cSt, similar to that of plasma.
The present in vivo study was undertaken to describe the effects of Hemolink™ on hemodynamics, cardiac function and organ blood flow (radionuclide-labeled microspheres) in the dog using an acute normovolemic hemodilution procedure and to compare the effects to those observed with hemodilution using the conventional volume expander Pentastarch®. Pentastarch® is a high viscosity, hyperoncotic starch solution with approximately 45% hydroxyethyl substitution that does not have the oxygen transporting capability of Hemolink™. Two different preparations of Hemolink™ were assessed, a preparation in the oxy-ligand state of Hemolink™ that is stored frozen and a preparation in the deoxy-ligand state of Hemolink™ that is stable when stored at room or refrigerator temperatures.
MATERIALS AND METHODS
Beagle dogs (7–10 Kg body weight) were acclimatized for 2 weeks before the study. Dogs were housed individually in a controlled environment and fed a standard certified commercial dog chow and given water ad libitum. A clinical veterinarian carried out a detailed physical examination and blood analysis to ensure good health before dogs were included in the study. All procedures were carried out in accordance with the “Guide to the Care and Use of Experimental Animals” (vols. 1 and 2) of the Canadian Council on Animal Care and also in accordance with the Good Laboratory Practice Regulations of the United States Food and Drug Administration (21 CFR Part 58 and subsequent amendments), OECD Principles of Good Laboratory Practice and the specifications of the Japanese Ministry of Health and Welfare (Ordinance No. 21) under the auspices of the Quality Assurance Department of ITR Laboratories Canada Inc.
Experimental Preparation
After fasting for 24h, anesthesia was induced with isoflurane (2–5%) by mask. Animals were ventilated mechanically with room air. End-tidal CO2 was monitored continuously and blood gases were maintained within normal physiological limits by adjusting the rate and volume of ventilation. An electrocardiogram was obtaining using bipolar limb leads (I, II, III, aVR, aVL, aVF). Dogs were placed on a heating pad in the decubitus position. A catheter was inserted in the left cephalic vein for administration of the test or control articles. A catheter inserted in the right cephalic vein was used for infusion of normal saline (200 mL/hr) to maintain fluid balance during the surgical protocol. Saline infusion was discontinued during and after acute isovolemic hemodilution. A 7F flow-directed catheter with thermodilution capacity (Baxter Healthcare Corp., Irvine, CA, U.S.A.) was introduced into the right femoral vein and directed into the pulmonary capillary wedge position to measure pulmonary arterial pressures and cardiac output. Correct positioning of the catheter was verified by the pressure tracings. A catheter (7F) was placed in the abdominal aorta via the right femoral artery for measurement of arterial blood pressure, blood gas sampling and withdrawal of reference arterial blood samples for the radionuclide-labeled microsphere blood flow technique. An 8F vascular introducer (Cordis Corp. Miami, FL, U.S.A.) was placed in the left femoral artery. A 7F pigtail catheter (Cordis Corp., Miami, FL, U.S.A.) was then passed through the introducer and positioned in the LV chamber for pressure measurement and injection of microspheres.
Experimental Protocol
Dogs (n = 5/group) were randomly allocated to one of the following treatments: 1-Pentaspan® (i.e., controls), 2-Hemolink™ 1, the oxy-ligand form of Hemolink™ and 3-Hemolink™ 2, the deoxy-ligand form of Hemolink™ (Hemosol Inc., Toronto, ON). A 30-minute equilibration period was allowed after surhgical instrumentation before acute isovolemic hemodilution. Hemodilution was initiated by partial exchange transfusion to a hematocrit level of approximately 25% in the control group and 20% in the two Hemolink™ groups. Hemolink™ treated dogs were exchanged to a greater extent than control dogs to reach the same target hemoglobin concentration in all groups. Hemolink™ adds hemoglobin to the plasma phase, therefore, a lower hematocrit was required in the Hemolink™ groups. Both formulations of Hemolink™ and Pentaspan® (DuPont Merck Pharma Inc.) were warmed for 30 min at 37°C prior to use. In the two Hemolink™ groups and in the control group receiving Pentaspan®, the exchange volume was 40 mL/kg and 33 mL/kg respectively. Both control and test articles were infused through an in-line filter (0.22 μm) concurrently with withdrawal of arterial blood at a rate of 8 mL/min. The pH of the test articles was measured prior to administration and confirmed to be within the normal range (pH = 7.35–7.45). Pressure transducers were connected to a Hewlett Packard monitoring system (Model M1117A; Hewlett Packard, Mississauga, ON). Electrocardiographic leads were connected to a Siemens Secard 444 monitoring system (Siemens, Montreal, Qc). Briefly, cardiac output was measured by injecting 2 mL of cold water (0–1°C) via the thermodilution catheter into the right atrium, via the atrial port of the pulmonary artery catheter. Cardiac outputs were determined in duplicate and analyzed using computer software associated with the Hewlett Packard M1117A monitoring system. Arterial, pulmonary, central venous pressures and heart rate were continuously measured. Cardiac output, arterial and venous blood gases, pH and hematocrit were measured prior to transfusion (i.e. baseline) and 15, 30, 60, 120 and 180 minutes after the exchange transfusion. Blood gases and pH were analyzed using a Stat Profile 1 blood gas analyzer (Nova Biochemical, Boston, MA) and hematocrit was determined by capillary tube centrifugation.
Solutions
10% Pentaspan®, a hyperoncotic solution was obtained sterile and ready for use. Hemolink™-1 (oxy-ligand form—Lot #98HMUS24) and Hemolink™-2 (deoxy-ligand form—Lot #99HDUS39), are pasteurized solutions of cross linked human hemoglobin (10 g/dL) dissolved in lactated Ringer's solution and stored either frozen at −80°C (oxy-ligand form) or at 4°C (de-oxy-ligand form). Mean physicochemical characteristics including: pH (7.7), P50 (45 mmHg), kinematic viscosity (1.11 cSt), colloid osmotic pressure (25.4 mmHg), metHb content (4.3%) and endotoxin levels (<0.06 EU/mL) were within acceptance limits.
Measurement of Regional Blood Flow
Distribution of blood flow was measured using the radionuclide-labelled microsphere technique.Citation[[3]] Five different radionuclide-labelled microspheres 113Sn, 85Sr, 95Nb,46Sc, 114In], each with a diameter of 15 ± 1 μm, were obtained from NEN (Boston, MA, U.S.A.). Immediately prior to injection, the microsphere suspension was agitated in a vortex mixer for 2 minutes. Each injection comprised 2–4 × 106 microspheres administered into the LV chamber as a bolus over 15–20 seconds and flushed with 15 ml of warmed saline. For each microsphere injection, a timed collection of arterial blood was performed with a calibrated infusion/withdrawal pump (Gemini PC-1; Imed Corporation, San Diego, CA) from the right femoral artery catheter at a constant rate of 8 mL/min beginning 10 seconds before microsphere injection and continuing for 2 minutes. Blood flow was evaluated at five time points: immediately prior to infusion of control or test article, at 30, 60, 120 and 180 minutes after hemodilution.
Post-mortem Analysis
At the end of the 3 hr study period, dogs were euthanized by an intravenous overdose of Euthanyl™ (Bimeda-MTC Animal Health, Cambridge, ON). Tissues were collected from the brain, heart, kidneys, large intestine, liver, lung, pancreas, small intestine and stomach. Tissue samples were quickly rinsed in saline and fixed by immersion in neutral buffered formalin (10%; v/v).
For blood flow analysis in the heart, the right ventricle was removed and the LV (including interventricular septum) was cut into slices (i.e., 6 mm thick; same thickness for all subsequently described tissue slices from different organs) from apex to base parallel to the atrioventricular groove. Three transverse myocardial sections (beginning with the second most apical slice) were used. The LV was divided into anterior, posterior and septum segments and further subdivided into endocardial, midmyocardial and epicardial portions. For blood flow analysis of the brain, consecutive sections from the forebrain were carefully dissected to separate white and grey matter. The brainstem was sectioned into three pieces. Two polar slices from each kidney were subdivided into medulla/cortex regions; four pieces of tissue from the liver, lung, large and small intestine, stomach and pancreas were also analyzed. Blood flows in tissue samples from the same organ were averaged.
Radioactivity in all tissue and blood reference samples was measured in a gamma-well scintillation counter (Cobra II, Canberra Packard Instruments, Montreal, CAN) with standard window settings. Tissue counts were corrected for background, decay and isotope spillover. Blood flow (ml/min/g) was calculated using the PCGERDA computer software (Packard Instruments, Montreal, CAN) and expressed in mL/min/gm.
Data Analysis
Values are expressed as mean ±1 SD. Paired t-tests were used to compare the baseline hemodynamic and hematocrit measurements to those obtained 15-min post-exchange. The 15-min post-exchange measurement were also compared to those obtained 3 hrs after exchange using paired t-tests. A sphericity test was initially carried out to check for a correlation between time-dependent variables for the different experimental groups. An analysis of variance (ANOVA) with repeated measures was subsequently carried out to analyze the blood flow data using SAS statistical software package (SAS Inc., Cary, NC, U.S.A.). Because of the large variation in the blood flow data for hepatic arterial flow a regression approach was performed using the logarithm of the standard deviations versus the logarithm of the means for group and time. This approach was used to stabilize variance between group means. The statistical comparisons for hepatic arterial flow were performed using these transformed values. A p≤0.05 was considered significant. A statistical trend was defined as a p value between 0.05 and 0.10.
RESULTS
Fifteen dogs were entered into the study. All animals survived the respective interventions, were sacrificed at the time the three hour study period was completed, and were included in the data analyses. No anaphylaxis or other adverse effects occurred after infusion of either test article. Electrophysiological recordings revealed minimal disturbances. One dog in each of the Pentaspan® and Hemolink™-1 groups exhibited transient isorhythmic tachycardia during the post-exchange period. Two premature ventricular contractions (PVCs) were observed prior to exchange transfusion in one animal in the Hemolink™-1 group. In another animal in the same group, two PVCs were observed post-exchange. A single PVC was also reported post-exchange in the Hemolink™-2 group. The remaining dogs maintained normal sinus rhythm throughout the experimental protocols.
Hematological Findings
A summary of the hematological findings is presented in . Hematocrit decreased significantly after the exchange transfusion in all groups. As per protocol, the hematocrit was significantly lower in both the Hemolink™ groups as compared to the Pentaspan® group (p<0.05 for both Hemolink™-1 and Hemohlink™-2 versus Pentaspan®) 30 minutes post-exchange. In the Pentaspan® group, hematocrit increased from 23 ± 1% at 30 min post-exchange to 25 ± 1% by the end of the three hour observation period (p = 0.03). Presumably, this increase reflects faster clearance of Pentaspan® from the circulation (approx. 2 hrs). In both Hemolink™ groups, no significant increase in hematocrit was observed from 30 minutes to 180 minutes post-exchange. There was no difference between any of the three groups in arterial blood gases, arterial O2 content or arterial pH at any time-point in the study. In all groups, exchange transfusion resulted in a progressive decrease in the venous PO2, O2 saturation and O2 content, indicative of increased oxygen extraction. There was no difference in post-exchange venous PO2, O2 saturation and O2 content in any of the three groups at any time-point.
Table I. Hematocrit and Blood Measurements
Effects of Hemodilution in the Control Group
summarizes the effects of exchange transfusion on hemodynamic variables in the three groups. Values are expressed as a percentage of baseline in the same animal prior to exchange, thus allowing each animal to serve as its own control. There were no significant differences in baseline values in any of the hemodynamic variables in any of the three groups (See ).
Figure 1. Changes in cardiac output (A), mean arterial pressure (B), heart rate (C), stroke volume (D), pulmonary capillary wedge pressure (PCWP) (E), central venous pressure (CVP) (F), systemic vascular resistance (SVR) (G), and pulmonary vascular resistance (PVR) (H), and left (I), and right (J) cardiac work during the 3-hour recovery period following isovolemic exchange-transfusion with Pentaspan® (squares); Hemolink™-1 (triangles) or Hemolink™-2 (upside-down triangles). Values are expressed as a percentage of the baseline value in the same animal prior to the exchange transfusion. Data are expressed as mean ± 1 SD.
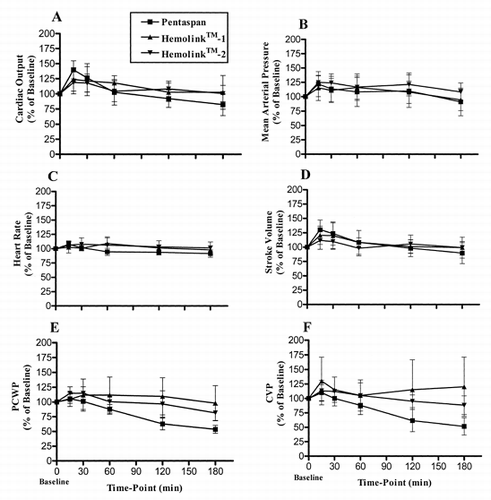
Table II. Baseline Hemodynamics in the Pentaspan® and Hemolink™ Groups
Consistent with the expected compensatory responses to this degree of hemodilution, dogs receiving Pentaspan® exhibited an increase in cardiac output (p = 0.006) 15 min post-exchange which was mediated by both an increase in heart rate (p = 0.03) and stroke volume (p = 0.02) (See ). Left and right ventricular minute work and stroke work (data not shown) were also increased (p<0.05). There was also a trend towards increased mean arterial pressures, although this did not reach statistical significance (p = 0.07). All of these increases were transient, since values for all of these measurements were at or below baseline values by 3 hrs post-exchange. There were no significant changes in pulmonary arterial wedge pressure, central venous pressure, systemic or pulmonary vascular resistances at the 15 min post-exchange time-point. However, pulmonary arterial wedge pressure and central venous pressure progressively declined over the 3 hr observation period while pulmonary vascular resistance tended towards an increase (p = 0.007).
Effects of Hemodilution in the Hemolink™ Groups
In both Hemolink™ groups, cardiac output also increased significantly 15 minutes post-exchange (p = 0.02 for Hemolink™-1 and p = 0.05 for Hemolink™-2). This increase in cardiac output was mediated more by an increase in stroke volume rather than an increase in heart rate since heart rate did not increase significantly in either the Hemolink™-1 or Hemolink™-2 groups. Similar to the control group, left and right ventricular minute work and stroke work (data not shown) were either significantly increased or showed a trend towards increase (p<0.07) immediately after the exchange. There was no significant change in mean arterial pressure or pulmonary arterial wedge pressure immediately after exchange in the Hemolink™-1 group. However, in the Hemolink™-2 group, both mean arterial (p = 0.004) and pulmonary capillary wedge pressure (p = 0.03) were increased after the exchange. Neither systemic nor pulmonary vascular resistances were significantly increased in either group 15-minutes following exchange, although pulmonary vascular resistance progressively increased in all groups by 3 hrs.
Unlike the control group in which the cardiac output, heart rate and stroke volume had significantly declined from the 15-min post-exchange value by 3 hrs, no significant declines in these measurements were observed in the Hemolink™ groups. Furthermore, unlike the control group that showed significant declines in central venous pressures and all measures of cardiac work from 15 min to 3 hrs post-exchange, no such decreases were observed in Hemolink™ groups. Pulmonary arterial wedge pressure also significantly declined from 15 min to 3 hrs postexchange in both the Pentaspan® and Hemolink™-2 group, but to a lesser extent in the Hemolink™-1 group.
Effects of Hemodilution on Distribution of Blood Flow
Changes in blood flow patterns after hemodilution with each of the test solutions is indicated in . There were no significant differences in baseline values in any of the organ flows in any of the three groups (See ).
Figure 2. Blood flow in the heart (A), brain (B), lung (C), brainstem (D), kidney cortex (E), pancreas (F), hepatic arterial (G), stomach (H), small intestine (I), and large intestine (J), during the 3-hour recovery period following isovolemic exchange-transfusion with Pentaspan® (squares); Hemolink™-1 (triangles) or Hemolink™ 2 (upside-down triangles). Values are expressed as a percentage of the baseline value in the same animal prior to the exchange transfusion. Data are expressed as mean ± 1 SD. It should be noted that the measured flow to the lung represents the sum of the bronchial arterial blood flow and the very small flow through systemic-venous shunts.
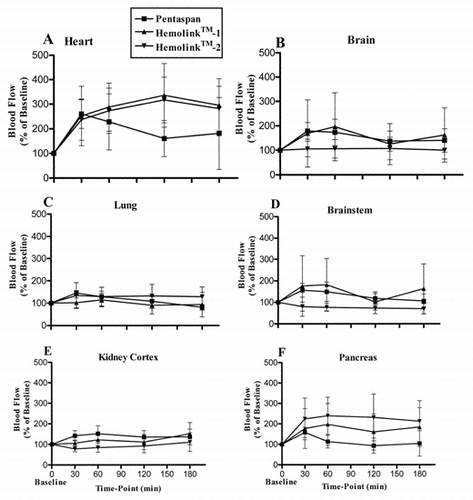
Table III. Baseline Organ Blood Flows in the Pentaspan® and Hemolink™ Groups
In the heart, blood flow increased significantly in the first 30 minutes after hemodilution with all test solutions. The increased myocardial blood flow persisted in the Hemolink™-1 and Hemolink™-2 groups for the duration of the study. However, in dogs given Pentaspan® the increase in blood flow was transient and declined to near baseline values by 3-hrs post-exchange. In all treatment groups the endocardial/epicardial blood flow ratio remained constant (data not shown) indicating preserved blood flow across the LV wall i.e., no coronary steal or ischemia. In the brain and brainstem, the increase in blood flow was transient in both the Pentaspan® and Hemolink™-1 groups. Blood flow was not changed over the duration of the study in animals given Hemolink™-2. In the kidney cortex, blood flow was increased transiently in the Pentaspan® group but was unchanged in the Hemolink™-1 and Hemolink™-2 groups. There were no differences between the groups in the flows to the stomach, large and small intestine. Pentaspan® produced a marked increase in blood flow 30 minutes post-exchange in the liver. These higher blood flow levels were maintained for the duration of the experiment in this organ. Interestingly, pancreatic blood flow was also greater 30 minutes post-exchange in both the Hemolink™-1 and Hemolink™-2 groups while in the Pentaspan® group blood flow returned to near baseline levels by the end of the experimental protocol.
DISCUSSION
Acute normovolemic hemodilution (ANH) has been developed as part of an overall strategy to minimize red cell loss in surgical bleeding, and to provide autologous blood. The cardiovascular effects of ANH include a decrease in viscosity and systemic vascular resistance with a compensatory increase in stroke volume and cardiac output, thus maintaining arterial blood pressure. There is also an associated redistribution of blood flow in favor of the vital organs. Although oxygen content of the arterial blood is decreased by ANH, because of the reduction in hematocrit and hemoglobin concentration, a compensatory increase in cardiac output maintains oxygen delivery and tissue oxygenation.Citation[[3]] The development of hemoglobin based oxygen carriers (HBOCs), such as Hemolink™, offer the potential of enhancing the ability of ANH by providing additional O2 transport capacity in the plasma, beyond that due to RBC hemoglobin thereby preventing excessive dilution of the blood's oxygen carrying capacity, currently a major limitation of ANH.
The purpose of the current study was to examine the effects of Hemolink™ on hemodynamics and organ blood flow in a large animal model that simulates conditions of ANH. Pentaspan® was used as the control test article in this study because it is one of the colloids commonly used for hemodilution in the surgical setting. It was not the intention of this study to match all properties, including the viscosities and oncotic pressures of the test and control articles, but rather to investigate the effects of ANH with these solutions under conditions that simulate their planned application.
Hemodynamic Responses to Hemodilution
In the current study, hemodilution with Pentaspan® was associated with an increase in cardiac output mediated by both an increase in heart rate and stroke volume and maintenance of arterial blood pressure. Cardiac work was increased. Acid-base balance, as judged by arterial pCO2 and pH, were not significantly altered by this degree of hemodilution (i.e. replacement of approximately 27% of the blood volume). These results are consistent with earlier findings on the effects of hemodilution.Citation[4-6] However, previous studies have also reported a significant reduction in systemic vascular resistance,Citation[5-6] which was not statistically significant in the current study.
In preclinical investigations of the cardiovascular effects of Hemolink™, and indeed all other HBOCs that are currently in clinical trials, a recurring theme has been the vasopressor effect combined with bradycardia. Since oxygen delivery depends not only on the oxygen content in the blood but also on cardiac output and blood flow to the various vascular beds, it is possible that the increased blood oxygen content produced when Hemolink™ is used, relative to that with a conventional colloid as a volume replacement is offset by a reduction in cardiac output and organ flow rate secondary to the rise in blood pressure. We saw no evidence of this in the current study. Cardiac output increased significantly following hemodilution in both Hemolink groups, to an extent that was not significantly different from controls. Furthermore, in both the Hemolink™ groups, arterial blood pressure and heart rate following hemodilution was similar to that observed in the control group. Other hemodynamic parameters were unremarkable except for a maintained increase in central venous pressure and cardiac work, in the Hemolink™ groups over the 180 minute period. Pulmonary arterial wedge pressure was also better maintained with Hemolink™. These pressures, and the general lack of hemodynamic deterioration in the Hemolink™ groups are indicative of sufficient intravascular volume and are probably reflective of the greater vascular persistence of Hemolink™. Further indicative of Hemolink™'s vascular persistence is the absence of hemoconcentration in the Hemolink™ groups over 180 min post-exchange that was evident in the Pentaspan® group. The absence of effect of an approximately 44% exchange transfusion on arterial pH, PCO2 in the Hemolink™ groups attests to the maintenance of overall acid-base homeostasis.
As reviewed by Adamson et al. previous studies have examined the hemodynamic effects of Hemolink™ in several hemorrhagic hypotension models.Citation[[2]] However, it should be noted that the isovolemic exchange model used in the current study is quite different from the hemorrhagic hypotension models studied previously, because confounding perturbations that are present in hemhorragic hypotension are not present during isovolemic exchange. Nevertheless, studies in these models show that Hemolink™ administration was generally followed by a transient 10–20% increase in mean arterial pressure above pre-infusion values although in some exchange models no increase in arterial pressure was observed. Heart rate decreases of about 10–30% were observed frequently in conscious animals, but these increases were absent in anesthetized animals. After acute hemorrhage, Hemolink™ effectively restored arterial blood pressure, left ventricular filling pressure and cardiac contractility. Neither systemic nor pulmonary vascular resistances were increased in these resuscitative models.
In addition, two published studiesCitation[7-8] have evaluated Hemolink™ in isovolemic exchange models in anesthetized rabbits where hematocrit was reduced to approximately 30%. In these studies, Hemolink™ resulted in an approximately 10–15% increase arterial blood pressure. Heart rate was unaffected by Hemolink™ administration, although vascular resistance was increased. Renal hemodynamics were not altered by exchange transfusion.
Organ Blood Flow Responses to Hemodilution
Organ flow, as measured by the microsphere technique, was transiently increased after hemodilution with Pentaspan® in all organs studied. Hepatic arterial blood flow in the Pentaspan® group also increased but was highly variable possibly due to the hepatic breakdown of Pentaspan®. Increased hepatic arterial flow following hydroxyethyl starch administration has previously been reported.Citation[[9]]
In the Hemolink™ groups, elevated blood flow levels to the heart were maintained throughout the 180 minutes post-exchange. In the heart, transmural distribution of blood flow is altered under ischemic conditions and flow is redistributed from the endocardium to the epicardium. As such, the endocardial tissue layer, perfused principally during diastole, is vulnerable to ischemia and infarction. In both Hemolink™ groups, the endocardial/epicardial flow ratios were maintained near unity throughout the post-hemodilution period, indicative that blood flow to the subendocardium was well maintained. In all other organs, there was no evidence of reduced blood flow relative to pre-exchange baseline levels, suggesting that tissue perfusion was not impaired.
In summary, when Hemolink™ is used as a volume replacement in a model of ANH in the anesthetized dog, cardiac output, mean arterial pressure and systemic and pulmonary vascular resistance were similar to those observed in the control group. Indicators of intravascular volume, including central venous pressure and pulmonary artery wedge pressure were better maintained in the Hemolink™ groups during the 3 hr post-exchange period. Blood flow to the heart, an organ particularly sensitive to the effects of ischemia, was maintained higher in the Hemolink™ groups throughout the post-exchange period. When compared to the pre-exchange condition, there was no evidence of significant impairment of tissue perfusion in any of the organs studied. Furthermore, acid-base homeostasis was well maintained with Hemolink™, despite an approximate 44% exchange transfusion in these groups.
Thus, while previous results have clearly and correctly identified HBOCs, including Hemolink™, to have some vasoconstrictive properties, the emergence of these properties depends critically on the model in which it is investigated. Our studies show that when used as a volume replacement in conjunction with ANH, adverse hemodynamic effects resulting from vasoconstriction can be alleviated, most likely by the reduction in blood viscosity, while maintaining blood flow.
REFERENCES
- Menu P, Bleeker W, Longrois D, Caron A, Faivre-Fiorina B, Muller S, Labrude P, Stoltz J F. In vivo effects of Hb solutions on blood viscosity and rheologic behavior of RBCs: comparison with clinically used volume expanders. Transfusion 2000; 40: 1095–1103
- Adamson J G, Moore C. Hemolink, an o-raffinose crosslinked hemoglobin-based oxygen carrier. Blood substitutes: principles, methods, products and clinical trials, T MS Chang. Karger Landes Systems, BaselSwitzerland 1998; II
- Baer R W, Payne B D, Verrier E D, Vlahakes G J, Molodowitch D, Uhlig P N, Hoffman J I. Increased number of myocardial blood flow measurements with radionuclide-labeled microspheres. American Journal of Physiology 1984; 246: H418–H434
- Doss D N, Estafanous F G, Ferrario C M, Brum J M, Murray P A. Mechanism of systemic vasodilation during normovolemic hemodilution. Anesthesia and Analgesia 1995; 81: 30–34
- Habler O, Kleen M, Hutter J, Podtschaske A, Tiede M, Kemming G, Corso C, Batra S, Keipert P, Faithfull S, Messmer K. Effects of hemodilution on splanchnic perfusion and hepatorenal function. II. Renal perfusion and hepatorenal function. Eur J Med Res 1997; 2: 419–424
- Tu Y K, Liu H M. Effects of isovolemic hemodilution on hemodynamics, cerebral perfusion, and cerebral vascular reactivity. Stroke 1996; 27: 441–445
- Caron A, Menu P, Faivre-Fiorina B, Labrude P, Alayash A I, Vigneron C. Cardiovascular and hemorheological effects of three modified human hemoglobin solutions in hemodiluted rabbits. Journal of Applied Physiology 1999; 86: 541–548
- Caron A, Menu P, Faivre-Fiorina B, Labrude P, Alayash A, Vigneron C. Systemic and renal hemodynamics after moderate hemodilution with HbOCs in anesthetized rabbits. Am J Physiol Heart Circ. Physiol 2000; 278: H1974–H1983
- Kleen M, Habler O, Hutter J, Podtschaske A, Tiede M, Kemming G, Corso C, Batra S, Keipert P, Faithfull S, Messmer K. Effects of hemodilution on splanchnic perfusion and hepatorenal function. I. Splanchnic perfusion. Eur J Med Res 1997; 2: 413–418