Abstract
Modified hemoglobins are being considered as possible “blood substitutes”. Experiments were performed to determine whether diaspirin cross-linked hemoglobin (DBBF-Hb) produces epithelial damage and whether this is reduced by selenium (Se). Anesthetized Sprague-Dawley rats, half of which received 2×10 −6 g/ml Se, daily for 3 weeks, in their drinking water, were injected with a 5 ml bolus of 10 mg/ml DBBF-Hb. Control animals received saline, (5 animals per group). After 30 min., the intestine was perfusion-fixed for light and electron microscopy. Eighty villi per rat were assigned an epithelial integrity index (E.I.), ranging from 1 (intact) to 3 (some cell-cell and cell-basement membrane separation). In non-Se rats, E.I. was significantly compromised by DBBF-Hb, compared to HBS-BSA (2.47±0.57 (SD) vs. 1.36±0.49, p<0.001). In Se rats, neither injection with DBBF-Hb, nor HBS-BSA, caused epithelial damage (1.03±0.17 vs. 1.07±0.26). Mast cell degranulation per villus (MCD) was measured in 60 villi per rat. In non-Se rats, MCD was significantly greater after DBBF-Hb than after HBS-BSA injection (1.83±1.42 vs. 0.2±0.4). Supplementary Se did not reduce this effect. In fact, MCD was significantly increased in both sets of rats compared to their non-Se counterparts: (3.27±2.40 and 1.48±1.70 for DBBF-Hb and HBS-BSA, respectively). Since mast cell mediators damage cells, Se must protect the mucosal epithelium in some way.
INTRODUCTION
Hemoglobin (Hb) is known to leave the circulation and produce toxic side effects if it is not contained within red blood cells, or erythrocytes. Most Hb-based molecules that are being developed for use as blood substitutes are not contained within erythrocytes or artificial “cells” (liposomes), and so the problem of extravasation needs to be considered. Apart from enclosing the Hb molecules, erythrocytes contain diphosphoglycerate, that inserts between the two beta globin chains of each Hb molecule. In this way, that the two alphabeta subunits are held together, thus stabilizing the Hb molecule. This property is also lost for free Hb. One way of minimizing the escape of free Hb from the circulation is to stabilize the Hb molecule by binding it to a substance such as polyethylene glycol (PEG). The molecular Stokes-Einstein radius of the blood substitute is then increased, thus reducing its chances of escape from the circulation. Another approach is to cross-link the alpha or beta globin chains to prevent the Hb molecules from dimerizing. Diaspirin cross-linked Hb (DBBF-Hb), in which the alpha chains are linked, was developed by the U.S. Army for use as a possible blood substitute.
Previously, it was shown that PEG-conjugated Hb (PEG-Hb), although five times larger than Hb (Stokes-Einstein radius of 250A versus 50A), extravasates rapidly from intestinal mucosal capillaries and causes epithelial detachment in villi near Peyer's patches (Citation[[1]], Citation[[2]]). Unfortunately, PEG-Hb is no longer available, and so subsequent experiments have been performed using DBBF-Hb. Both PEG-Hb and DBBF-Hb induced venular leakage in the mesentery, similar to that produced by histamine (Citation[[3]]) One property of hemoglobins that may account for their deleterious effects on the microcirculation and on intestinal tissue is the fact that they undergo auto-oxidation. Harmful reactive oxygen species, such as superoxide (O 2 −) and hydrogen peroxide (H 2O 2) are by-products of auto-oxidation. As stated by Alayash (Citation[[4]]), the reaction between oxy or ferric forms of Hb with H 2O 2 produced by auto-oxidation, or by neutrophils and macrophages, proceeds via the highly reactive ferryl intermediate Hb(Fe IV) and may lead to Hb degradation and cytotoxicity. Another possible redox mechanism involves the release of free iron from Hb after oxidative damage. The free iron then catalyzes the reaction between H 2O 2 and O 2 − to form the hydroxyl radical (OH −) (Citation[[5]]). However, Simioni et al. (Citation[[6]]) found that when Hb was reacted with H 2O 2 in vitro, from 15 minutes to 24 hours, very little Fe was released. Therefore, either modified hemoglobins are less stable in vivo than they are in vitro, or else most ROS are produced via the ferryl radical, Hb(Fe IV).
Previous experiments have shown that a bolus injection of DBBF-Hb produces ROS generation in the intestinal mucosa of rats (Citation[[7]]). The ROS were detected by fluorescence of dihydrorhodamine (DHR) 123. Pre-injection with the ROS scavengers, superoxide dismutase (SOD) and catalase, significantly reduced DHR fluorescence, but only for the first 10 minutes after injection of DBBF-Hb. Preliminary studies also indicated that injection with DBBF-Hb caused disruption of the intestinal epithelium, and that this was temporarily reduced by pre-injection with SOD and catalase. It is possible that tissue damage, caused by injection of modified hemoglobins, could be reduced for more extensive time periods by selecting an antioxidant that is more effective than SOD and catalase in the intestine.
It has been suggested that the activities of several antioxidant enzymes, including catalase, superoxide, and glutathtione peroxidase, are high in the intestinal epithelium (Citation[[8]]). Glutathione peroxidase is a tetrameric enzyme present in cytosol that reduces H 2O 2 as well as fatty acid hydroperoxides. Thus it provides protection against oxidative damage and accumulation of free radical products. The biological activity of glutathione peroxidase depends on the presence of Se covalently bound to the cysteine moiety of the protein (Citation[[9]]). Prevention of formation of lipid peroxides may be particularly important in the gastrointestinal tract as these tissues are frequently exposed to such substances. The activity of glutathione peroxidase increases with increasing levels of dietary selenium in tissues of experimental animals (Citation[[10]]). In addition, supplementation with Se can prevent the structural damage and disruption of cellular integrity caused by peroxidation of membranes and other cell components (Citation[[11]]). Simioni et al. (Citation[[12]]), demonstrated that treatment with Se was very effective in the prevention of oxidative damage induced by Hb. In these experiments, lipid peroxidation was evaluated by measuring the formation of conjugated dienes and thiobarbituric acid reactants. Both products were significantly increased in the liver, heart and plasma of control rats after injection of modified bovine Hb, but not in the plasma and tissues of rats that had received a dietary supplement of selenium selenite. In addition, injection of Hb caused histological changes in the liver and myocardium of control rats, but not in those that had received Se. However, in this study, treatment of the rats with Se only slightly activated the expression of glutathione peroxidase when measured after injection of modified Hb, in comparison with the untreated controls. It was postulated that large quantities of O 2 − formed following injection with Hb and that the O 2 − inhibited the activity of catalase and glutathione peroxide, allowing accumulation of H 2O 2 and subsequent formation of hydroxyl and ferryl radicals. In order to explain the protective effect of Se on epithelial integrity, the authors hypothesized that Se may act as part of a system other than glutathione peroxidase to prevent oxidative injury.
The effects of Hb injection and Se supplements on the intestine have not been investigated even though dietary Se appears to be a choice antioxidant for preventing oxidative damage in the intestine. For these reasons, experiments were performed to determine whether a bolus injection of DBBF-Hb causes damage to the epithelium of the intestinal mucosa, and whether this damage is reduced by prior treatment with Se. Experiments were also performed to determine whether Se reduces the production of excess ROS in intestinal mucosal tissue, following injection of DBBF-Hb.
MATERIALS AND METHODS
DBBF-Hb
The DBBF-Hb was produced by the United States Army (kind gift of Dr. Abdu Alayash, Center for Biologics Evaluation and research, Food and Drug Administration, NIH). It is stabilized by intramolecularly cross-linking the α-subunits within the Hb tetramer. The batch of DBBF-Hb used in these experiments contained 1.2% met Hb as measured by spectrophotometry.
Selenium Pretreatment
Male Sprague-Dawley rats (350 g–450 g) were housed two per cage, fed Harlan Tech Lab 485 rat chow, and placed on a light cycle with lights on between 6:00 A.M.. and 6:00 P.M.. Half of the rats received 2 μg/ml sodium selenite (Sigma Chemicals, St. Louis, MO) in their drinking water. Selenium has previously been administered to rats in their drinking water at a dose of 0.1 μg/ml and has been shown to be effective in increasing antioxidant enzyme activities (Citation[[13]]). In the present experiments, the rats drank an average of 32 ml water per day; therefore, they were receiving 64 μg of Se daily. The rats were kept, as described, for 3 weeks before experiments were performed on them.
Selenium Evaluation
Experiments were performed on 5 control and 5 Se-treated rats to determine the concentrations of Se in the blood and in the intestinal tissue. Each rat was anesthetized with 1 ml 3:1 ketamine:acepromazine. The abdominal cavity was opened by midline incision and the aorta exposed. An 18-gauge needle was attached to a heparinized syringe, and the needle was carefully inserted, retrograde, into the aorta so that 3 ml of blood could be collected. The blood was placed in 3 heparinized centrifuge tubes (1 ml in each), after removing the needle from the syringe to prevent hemolysis. The tubes were then shaken and placed in dry ice for later storage at −80°C. Next, the distal and proximal ends of the small intestine were clamped, as were the mesenteric artery and vein. The entire small intestine was then excised and divided into 3 segments. Each segment was wrapped in tinfoil, labeled, and placed on dry ice. The animals were euthanized with an injection of Beuthanasia in the jugular vein. Blood and tissue samples were packed in dry ice and sent to Oscar E. Olson Biochemistry Laboratories, South Dakota State University, for analysis of Se content using a fluorometric method (Citation[[14]]).
Experimental Design
The following study was performed to characterize the intestinal tissue damage caused by intravenous injection of DBBF-Hb in control and in Se-treated rats. The experimental design was as follows: a 5 ml bolus of 10 mg/ml DBBF-Hb was injected via the aorta in both groups of rats and allowed to circulate for 30 minutes before perfusion-fixing the small intestine and preparing tissue samples for light and electron microscopy. Half of the animals (control and Se-treated) received a saline injection instead of DBBF-Hb. Five animals were used in each of the 4 groups. This number of animals was justified to be sufficient by utilizing a sample size nomogram in conjunction with estimates of the difference in means that needed to be detected, and the mean standard deviation for each parameter (Citation[[15]]).
Surgical Procedures
Male Sprague-Dawley rats were anesthetized with pentobarbitone (6 mg/100 g wt., i.p.) In each rat a tracheometry was performed for artificial ventilation. Next, the aorta was cannulated just downstream from the superior mesenteric artery in a retrograde direction. The free end of the catheter tubing was connected to a reservoir of HBS, pH 7.4, at 37°C, which could be pressurized to 100 mmHg to allow for perfusion and thus check that the cannulation had been successful. A loop of intestinal ileum, close to the cecum, was pulled outside the body cavity and arranged on a plexiglas pillar attached to the plastic stage on which the rat was situated. Fifty mg of DBBF-Hb (10 mg/ml) in Hepes Buffered Saline (HBS), containing 2% bovine serum albumin (BSA), was injected through a 0.2 micron filter via the aortic cannula and allowed to circulate with the blood for 30 minutes. Half of the rats received HBS-BSA alone. After 30 minutes, the aorta was clamped proximal to (upstream of) the superior mesenteric artery, and the intestinal circulation was perfused with Karnovsky's fixative in phosphate buffer, pH 7.4, at 4°C. When perfusion was complete, the inlet pressure was dropped to 40 mmHg and the portal vein was clamped. The animal was killed with an intravenous injection of Beuthanasia. Fixation continued for 60 minutes and fixative was also applied to the outside of the intestinal segment. After fixation, the intestinal loop was excised and cut into several segments, each about 1 cm long, some containing a Peyer's patch; these segments were washed in buffered saline. Each segment was opened longitudinally and divided into squares a few mm long. Two squares were kept from each segment (one with a Peyer's patch) and processed as described below.
Tissue Preparation for Light and Electron Microscopy
Tissue squares were immersed in diaminobenzidine (DAB) overnight in the dark to stain specific granules in immune cells, and thus make the cells easier to identify. The DAB was prepared as follows (Citation[[16]]):
DAB (0.1 g) was added to 50 ml 0.1M monobasic phosphate buffer and the pH was adjusted to 7.2 very gradually with concentrated NH 4OH. The solution became a light tannish-pink color. Next, the tissue squares were rinsed in distilled water. Meanwhile, 25 ml DAB solution was added to 1.66 ml 3% H 2O 2 to give final concentration of 0.2%. The tissue was placed in this solution for 60 minutes and then rinsed three times in 0.15 M sodium cacodylate buffer. Finally, the tissue was dehydrated in increasing concentrations of ethanol and embedded in Spurrs resin. The pieces of tissue were oriented in the resin so that the blocks could be sectioned perpendicular to the villus plane. Thick sections (2 μm) were cut for light microscopy (Zeiss Axioplan), mounted on slides, and stained with toluidine blue. Ultrathin sections were cut for electron microscopy (Phillips CM12). Before examining the sections under electron microscopy, the grids were stained with lead citrate and uranyl acetate.
Data Acquisition and Analysis
Thick sections, cut longitudinally through the intestinal villi, were examined under light microscopy to count the numbers of degranulated mast cells and secreting goblet cells, per villus cross section, in 60 villi per animal. Only villus sections that contained a central lacteal were included because these villi were centrally sectioned. Mast cells were considered degranulated if they exhibited empty vacuoles (see ). For collection of data the slides were coded and the code was not revealed until the data were analyzed using one-way analysis of variance. If a significant difference was found between groups, pairs of groups were compared using the Tukey test. A probability value of <0.05 was chosen to denote statistical significance. Electron microscopy was used to assess the integrity of the epithelium. Sections cut transversely through eighty villi were observed for each animal at a magnification of ×3000. Each epithelial observation over a field of view, was assigned an Epithelial Integrity Index (E.I.), ranging from 1 to 3. Intact epithelium was assigned an E.I. of 1; epithelium with some cell-cell separation was assigned 2, and epithelial cells detaching from the basement membrane scored 3. The statistical analysis was identical to that previously described.
Measurement of ROS in Intestinal Mucosa
Experiments were performed on 12 Se rats and 12 control rats. The rats were anesthetized with 1ml 3:1 ketamine:acepromazine, followed by an intraperitoneal injection of sodium pentobarbital (6 mg/100 g wt.). In each rat a tracheometry was performed for artificial ventilation. Next, the aorta was cannulated just downstream from the superior mesenteric artery in a retrograde direction, as described previously. A 2–3 cm longitudinal slit was made down the side of the abdomen and a segment of intestinal mucosa about 2 cm long, containing a Peyer's patch, was cauterized longitudinally, slit open, and the mucosal surface spread over a plexiglass pillar incorporated within the plastic stage supporting the rat. The plastic stage was secured on a microscope stage, beneath a long working-distance objective and on top of a plate heated electrically to 37°C. The mucosal segment was kept moist with a HBS drip heated to 37°C, and examined under epifluorescence (100W mercury lamp light source) at 488 nm excitation wavelength, using a Zeiss Axioplan microscope with a ×20 objective of numerical aperture 0.6. The microscope was focused on the villi surrounding the Peyer's patch and any autofluorescence from the tissue was recorded by means of a video camera (Optronix VI 470) and a video recorder. Next, the HBS was replaced by HBS containing 0.001 g/dl dihydrorhodamine 123 (DHR). This substance is not fluorescent until oxidized by a high energy radical. The rate of accumulation of the fluorescent product for a constant suffusate concentration of DHR provides quantitative information on oxidant formation (Citation[[17]]). An image of fluorescence from the same villi was recorded. Each fluorescence observation was limited to a few seconds, after which a barrier filter was applied to block the fluorescence and prevent photobleaching. Next, a 5 ml bolus of 10 mg/ml DBBF-Hb, or HBS-BSA, was injected into the aorta through a 0.2 μm filter, and a timer was started on the video monitor. Every 2 minutes, for the next 30 minutes, a few drops of DHR were applied to the preparation, and an epifluorecent image recorded. Finally, the animal was sacrificed with an overdose of anesthetic.
Data Analysis for ROS Quantitation
The mean intensity of epithelial fluorescence of each image was measured using an analog-to-digital converter, a computer equipped with a video acquisition card, and computer software (NIH-Image), and the background value (DHR before injection) subtracted. Data were pooled among the 6 animals in each group for each time point, and the means and standard deviations calculated. Comparisons between group means were made using ANOVA statistics. If a significant F-value was obtained, significant differences between particular pairs were determined using the Tukey test.
RESULTS
Selenium Evaluation
The amount of Se in plasma of rats that were not given Se in their drinking water, was 0.496±0.011 (SD) μg/ml, (n=4). Rats given exogenous Se showed significantly more Se in their plasma [0.571±0.010 (SD), (n=4)]. The corresponding values for intestinal tissue were 0.363±0.027 (SD), n=4) and 0.460±0.038 (SD), (n=4); the Se-fed rats showed significantly more Se in their tissue. The basal value for Se in plasma is close to that obtained from previous studies in which rats were fed a diet with a standard content of Se (Citation[[18]], Citation[[19]]). These values were 0.414±0.038 (SD), and between 0.480 and 0.516, respectively. Administration of exogenous Se increased the plasma concentration of Se by 15% in this study, which is identical to the result from a previous study in which rats received 5 μg/kg body wt sodium selenite in their drinking water for 7 days, followed by an intramuscular injection of 5 μg/kg body wt sodium selenite (Citation[[12]]). Administration of exogenous Se increased the concentration of Se in the intestine by 27% in this study. No values for Se concentrations in intestinal tissue could be found in the literature.
Degranulated Mast Cells
Mucosal degranulated mast cells (dmc) were easy to identify by light microscopy because they stained intensely with toluidine blue and demonstrated empty vacuoles as shown in (arrowheads). An electron micrograph of a mucosal dmc is shown in d (arrowhead). Note the similarity between the cells as seen by light and electron microscopy. The electron micrograph of the dmc is typical of those shown in the literature (Citation[[20]]). A comparison of the degree of mast cell degranulation seen in the 4 groups is shown in . In villi adjacent to, and removed from Peyer's patches, significantly more mast cell degranulation was seen in Se rats that had received DBBF-Hb injection than in the other 3 groups. These rats showed significantly more mast cell degranulation than their non-Se counterparts, both adjacent to Peyer's patches (3.27±2.40 (SD) versus 1.83±1.42), and away from Peyer's patches (3.15±1.87 (SD) versus 1.33±1.17 (SD). Se rats that received injection of HBS-BSA showed a lower mast cell degranulation than their DBBF-Hb-injected, Se counterparts (1.48±1.70 (SD) versus 3.27±2.40 (SD)), but demonstrated a significantly higher mast cell degranulation than HBS-BSA injected, non-Se rats (1.48±1.70 (SD) versus 0.2±0.4 (SD)). These data are from villi near Peyer's patches, but similar results were observed away from Peyer's patches. Thus, administration of exogenous Se significantly increases mucosal mast cell degranulation following intravascular injection of DBBF-Hb and HBS-BSA.
Secreting Goblet Cells
The extent of goblet cell secretion, following injection of DBBF-Hb or HBS-BSA, in Se and non-Se rats is shown in . Injection of HBS-BSA in non-Se rats caused negligible goblet cell secretion, regardless of villus location (0.05±0.29 (Peyer's patch) and 0.13±0.53 (away)). In Se rats, the same treatment was accompanied by significantly more Goblet cell secretion, regardless of villus location (1.22±2.07 and 1.40±2.07). However, injection of DBBF-Hb did not give differing results according to whether or not the rats had received Se; in both cases, goblet secretion was high. In Se rats, the values were 3.11±3.38 (Peyer's patch) and 1.14±1.62 (away), while in non-Se rats the corresponding values were 3.04±4.05 and 1.42±2.22. Thus, it appears that administration of Se increases goblet cell secretion in otherwise unperturbed intestinal mucosa, but does not increase goblet cell secretion above that already caused by DBBF-Hb.
Electron Microscopy
shows typical electron micrographs of transverse sections through villi from the following groups: (a) HBS-BSA/non-Se, (b) HBS-BSA/Se, (c) DBBF-Hb/non-Se, and (d) DBBF-Hb/Se. In control preparations (i.e., non-Se rats that were injected with HBS-BSA), as shown in a, the epithelial cells (E) were intact, adhering closely to the basement membrane and to each other. An oblique section through a mucosal capillary (C) is also visible. Selenium rats that were injected with HBS-BSA also showed epithelium that was largely intact (b). Non-Se rats that were injected with DBBF-Hb showed disrupted epithelium (c). The cells were largely separated from each other and linked only by long, cytoplasmic protuberances. Sometimes the cells were no longer attached to the basement membrane. A goblet cell (GC) in the process of secretion can be seen in the electron micrograph. Selenium rats that were injected with DBBF-Hb did not show epithelial disruption (d). However, many deganulated mast cells could be seen. The average Epithelial Integrity indices for each of the four groups are depicted in . In non-Se rats, E.I. was significantly compromised by DBBF-Hb, compared to HBS-BSA, (2.47±0.57 (SD) vs. 1.36±0.45, p<0.001). In Se rats, neither injection with DBBF-Hb, nor HBS-BSA, caused epithelial damage (1.03±0.17 vs. 1.07±0.26).
Figure 4. Typical electron micrographs of transverse sections through villi from the following groups: (a) rats injected with HBS-BSA and fed a normal diet, (b) rats injected with HBS-BSA and fed selenium supplement, (c) rats injected with DBBF-Hb and fed a normal diet, and (d) rats injected with DBBF-Hb and fed selenium supplement. In (a) and (b), the epithelial cells, E, are intact, adhering closely to the basement membrane and to each other. Sections through mucosal capillaries, C, can be seen. In (c) the epithelial cells are separated from each other and linked only by long, cytoplasmic protuberances. A goblet cell, GC, in the process of secretion can be seen. In (d) the epithelial cells are intact but the mast cells are degranulated (dmc). Magnification: ×6000.
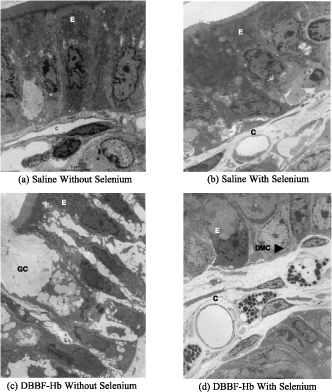
Figure 5. Histogram to show Epithelial Integrity Index of villi from tissue within, and remote from, Peyer's patches, after injection of saline or DBBF-Hb in rats fed supplemental selenium or a normal diet. The scale ranges from 1 to 3; a score of 1 means intact, and 3 means cels separated from each other and from the basement membrane.
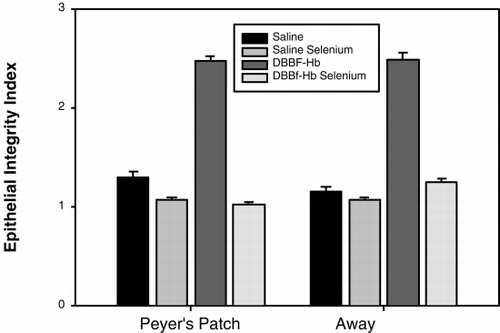
Reactive Oxygen Species
When non-Se rats were injected with HBS-BSA, the fluorescence in the epithelium (), and in the whole villus tissue (), gradually increased and peaked between 16 and 18 minutes after injection. The epithelium was brighter than the lamina propria from 2 minutes after injection until the end of the observation period, as shown in , in which the difference in fluorescence intensity between the epithelium and lamina propria at different time points is plotted. When Se rats were injected with HBS-BSA, the epithelium became bright less than 2 minutes after injection and remained bright for 18–20 minutes after injection (). Brightness averaged over the whole villus tissue followed a similar time course (). The epithelium was brighter than the lamina propria between 2 and 18 minutes after injection (). In Se rats, the epithelium, and the whole villus tissue were, on average, significantly brighter than those of the non-Se rats at each specific time period between 2 and 8 minutes after injection (p<0.05), but after 18 minutes the fluorescence rapidly faded ( and ). When the difference in brightness between the epithelium and the lamina propria was considered (), the results for Se rats and non-Se rats were very similar.
Figure 6. Histogram to show fluorescence intensity of dihydrorhodamine 123 in the epithelium of intestinal villi, after injection of saline or DBBF-Hb in rats fed supplemental selenium or a normal diet. On the abscissa the time after injection is plotted in minutes.
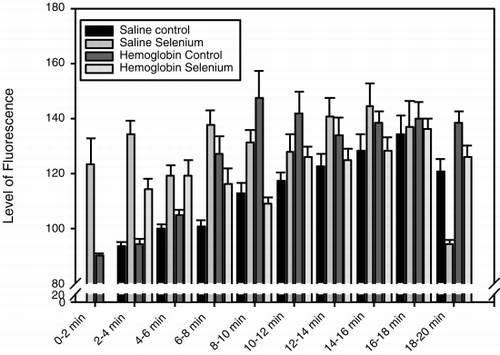
Figure 7. Histogram showing the fluorescence intensity of dihydrorhodamine 123 in the lamina propria of intestinal villi, after injection of saline or DBBF-Hb in rats fed supplemental selenium or a normal diet. On the abscissa is plotted the time after injection in minutes.
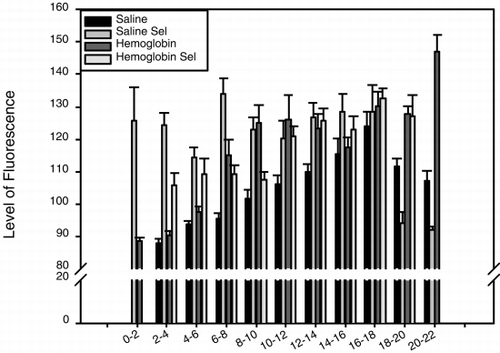
Figure 8. Histogram showing the difference in fluorescence intensity of dihydrorhodamine 123 between the epithelium and lamina propria of intestinal villi, after injection of saline in rats fed supplemental selenium or a normal diet. On the abscissa is plotted the time after injection in minutes.
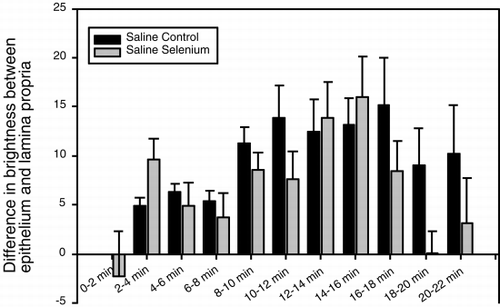
When the non-Se rats were injected with DBBF-Hb the fluorescence in the epithelium (), and in the whole villus tissue (), increased from 2 to 10 minutes after injection and remained intense for the whole observation period. The epithelium was brighter than the lamina propria throughout the observation period, and this was particularly marked at 8 to 10, and at 14 to 16 minutes after injection (). When Se rats were injected with DBBF-Hb, the epithelium became bright 2 minutes after injection and remained bright throughout the observation period (). Fluorescence intensity averaged over the whole villus tissue was also high 2 minutes after injection, and gradually increased throughout the observation period (). The epithelium was only brighter than the lamina propria during the first 2 to 8 minutes after injection (). In Se rats, the epithelium, and the whole villus tissue were, on average, significantly brighter than those of the non-Se rats between 2 and 4 minutes after injection (p<0.05) ( and ). A noticeable difference was seen between Se and non-Se rats when the difference in brightness between the epithelium and the lamina propria was considered (). A significantly greater difference was seen in non-Se rats compared to Se rats at times greater than 8 minutes after injection (p<0.05).
DISCUSSION
This study demonstrated that selenium reduced DBBF-Hb-induced epithelial disruption. This result is consistent with the fact that selenium supplementation increases concentrations of the endogenous antioxidant, glutathione peroxidase, which is abundant in epithelial cells. Glutathione peroxidase is a selenium-containing enzyme in cell cytoplasm that catalyzes the reduction of H 2O 2 and organic hydroperoxides. However, Se also increased mast cell degranulation in rats that received DBBF-Hb and HBS-BSA. Since many mast cell mediators cause cell damage, and Se reduced epithelial disruption in these experiments, it appears that Se has an overwhelmingly protective effect on the mucosal epithelium.
The effect of Se on mucosal mast cells may be explained by the fact that Se is able to activate neutrophils to produce oxidants. Selenium deficiency has been shown to inhibit the oxidative respiratory burst of neutrophils (Citation[[21]]), whereas supplementation with Se resulted in marked functional enhancement of neutrophil responses (Citation[[21]], Citation[[22]]). Kiremidjian-Schumacher and Stotzky (Citation[[23]]) postulated that the amount of selenite that interacts with the surface of immunocompetent cells may affect the surface properties of the cells and affect not only the uptake, retention, and efflux of molecules by the cells, but also their ability to respond to stimuli. It is known that neutrophils line the lamina propria of the intestinal villi (Citation[[24]], Citation[[25]]). In the intestine, oxidants produced by activated neutrophils can then cause mast cell degranulation because mast cells and neutrophils coexist in the mucosal lamina propria (Citation[[26]]). In the present study, administration of Se to rats produced goblet cell secretion even in the absence of DBBF-Hb. Since goblet cell secretion acts as a protective mechanism in the intestine, this is further evidence that Se may disturb the intestinal mucosa despite producing increased concentrations of glutathione peroxidase in the epithelium.
With regard to production of excess ROS in the intestinal epithelium, the results of DHR fluorescence indicate that immediately after injection of HBS-BSA or of DBBF-Hb, fluorescence intensity in the epithelium is transiently increased in Se rats compared to non-Se rats—up to 16 minutes after injection in the case of HBS-BSA, and up to 6 minutes in the case of DBBF-Hb. It is possible that this response is caused by activation of intraepithelial leukocytes that often lie between the basolateral aspects of epithelial cells (Citation[[22]]). The intraepithelial leukocytes then produce ROS, subsequently causing mast cells to degranulate and produce additional ROS. However, although it is known that leukocytes, when activated, can produce ROS (Citation[[27]]), there is no evidence that Se can activate intraepithelial leukocytes. In addition, further experiments are required to determine whether the observed epithelial brightness can be pinpointed to the leukocytes. Similar results were observed with fluorescence intensity in the lamina propria. In Se rats injected with HBS-BSA, the intensity in the lamina propria was greater than that seen in non-Se rats for up to 16 minutes after injection. In Se rats injected with DBBF-Hb, the fluorescence intensity was only greater than for non-Se rats for the first 6 minutes after injection. The fluorescence in the lamina propria probably arose from Se-induced activation of neutrophils. Dihydrorhodamine 123 has been used to detect activation of the respiratory burst of neutrophils (Citation[[28]]). However, further experiments are required to positively identify the neutrophils as perpetrators of the DHR fluorescence.
A major difference between injection of HBS-BSA and DBBF-Hb in the DHR experiments was that about 6 minutes after injection of DBBF-Hb, the non-Se rats, rather than the Se rats, showed higher fluorescence intensity in the intestinal villi. The increased intensity occurred in the epithelium and in the lamina propria, but was most marked, and longer lasting, in the epithelium. These responses did not occur after injection of HBS-BSA. It is possible that this somewhat delayed increased fluorescence, and hence ROS production, occurred as follows. Epithelial cells, due to their high density of mitochondria, produce high quantities of ROS (superoxide (O 2 −) and H 2O 2) as products of respiration. Normally, the excess ROS are scavenged by endogenous anti-oxidants, such as catalase, superoxide dismutase and glutathione peroxidase. However, in the experiments described above, injection of either HBS-BSA or DBBF-Hb diluted the concentration of these scavengers in the blood, and this resulted in excess ROS in the circulation. This phenomenon has been noted previously in another study in which it was shown that when resuscitation fluids lacking ROS scavengers, such as pasteurized plasma protein solution, or 0.9% saline, are injected into the circulation, peroxyl radical trapping capacity is decreased (Citation[[29]]). This does not occur when fresh plasma or donor red blood cells are used. It was suggested that the decreased antioxidant capacity was a result of dilution of the blood by the resuscitation fluids.
After injection of DBBF-Hb, it is possible that the excess ROS reacted with the hemoglobin and oxidized it to the ferric form, methemoglobin, which destabilizes the protein and allows the heme group to become detached (Citation[[30]]). The heme then releases free iron that may catalyze production of the hydroxyl radical by the Fenton reaction, thus increasing the ROS concentration (). Alternatively, the functional form of hemoglobin, that contains iron in the ferrous state, may react with H 2O 2 to produce the ferryl hemoglobin radical and the hydroxyl radical (Citation[[31]]). Rats that had received Se, would have a higher concentration of glutathione peroxidase in their epithelial cells, and so it is probable that the initial formation of excess ROS, caused by ROS scavenger dilution and possible activation of intraepithelial leukocytes, would be reduced in Se rats. The inhibition of DBBF-induced increased ROS in the epithelium by Se is consistent with our observations of high epithelial integrity in those Se animals that received DBBF-Hb.
Figure 10. Diagram of the possible roles of selenium in reducing hemoglobin-induced intestinal damage. MCD: mast cell degranulation.
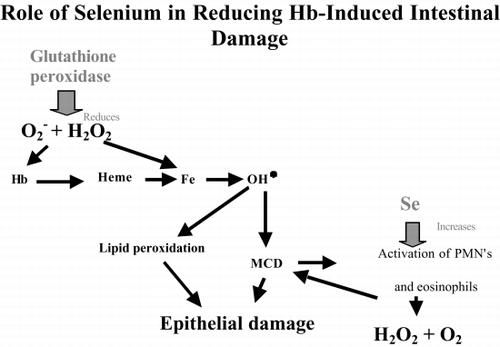
One rather puzzling result in this study was our observation of high DHR fluorescence intensities in the epithelium of Se rats immediately after injection with HBS-BSA, and lasting for 16–18 minutes () without accompanying epithelial disruption, as measured 30 minutes after injection ()b. One possibility could be that the epithelium had repaired itself after 30 minutes. However, our previous experiments indicate that epithelial damage, induced by PEG-Hb at least, takes from between 60 to 90 minutes to repair (Citation[[2]]). Another possibility is that, although the DHR epithelial fluorescence in this case was similar in intensity to that caused, at later time points, in non-Se rats injected with DBBF-Hb, the species of ROS produced were different. Royall and Ischiropoulos (Citation[[32]]) reported that DHR is most useful as a marker of a change in general cellular oxidant production. Dihydrorhodamine 123 is oxidized by a variety of H 2O 2-dependent reactions, and so oxidation, and hence fluorescence, could be produced by several measurements. These include (1) the reaction of H 2O 2 with hemoglobin to give the ferryl and the hydroxyl radicals; (2) the reaction of H 2O 2 with intracellular cytochrome c, an electron carrier in the respiratory chain; or (3) by excess H 2O 2 alone (Citation[[32]]). Excess H 2O 2 in the tissue would be produced by plasma dilution after injection of HBS-BSA, and by itself would not cause the extensive tissue damage that is perpetrated by the ferryl radical (Citation[[12]], Citation[[31]]). Thus, DHR fluorescence can be associated with different species of ROS, some of which produce much more cellular damage than others.
Finally, it is possible that Se may also interact directly with hemoglobins. Morrison et al. (Citation[[33]]) reported that whole blood contains Se bound to hemoglobin. Studies are currently in progress to determine the effect of Se on the oxidation status of several modified hemoglobins.
To summarize, this study has shown, for the first time, that Se is effective in reducing the damage to the intestinal mucosa that is caused by hemoglobin-derived blood substitutes. It has also demonstrated that Se must be used with caution, because although Se protects epithelial cells by increasing intracellular concentrations of glutathione peroxidase, it may, in some cases, increase production of ROS, possibly by activating neutrophils. If Se were to be used as an antioxidative agent in conjunction with hemoglobin-derived blood substitutes, it would be necessary to administer both substances together, rather than priming the subject with Se in advance. Therefore, future studies will be aimed at evaluating other methods of Se supplementation.
ACKNOWLEDGMENTS
This work was funded by National Heart, Lung and Blood Institute Grant HL-53047.
REFERENCES
- Baldwin A. L. Blood Substitutes and the Intestinal Microcirculation: Extravasation and Ultrastructural Alterations. Advances in Blood Substitutes: Industrial Opportunities and Medical Challenges, R. M. Winslow, K. D. Vandergriff, M Intaglietta, 1997; 19–37
- Baldwin A. L., Wilson L. M., Valeski J. E. Ultrastructural effects of intravascularly injected polyethylene glycol-hemoglobin in intestinal mucosa. Am. J. Physiol. 1998; 275(Heart Circ. Physiol. 44)H615–H625
- Baldwin A. L. Modified hemoglobins produce venular interendothelial gaps and albumin leakage in the rat mesentery. Am. J. Physiol. 1999; 277(Heart Circ. Physiol. 46)H650–H659
- Alayash A. I. Hemoglobin-based blood substitutes: oxygen carriers, pressor agents, or oxidants?. Nat. Biotechnol. 1999; 17: 545–549
- Gutteridge J. M.C. Iron promoters of the Fenton reaction and lipid peroxidation can be released from hemoglobin by peroxides. FEBS Lett. 1986; 201: 291–295
- Simioni J., Simoni G., Lox C. D., Feola M. Reaction of human endothelial cells to bovine hemoglobin solutions and tumor necrosis factor. Art. Cells, Blood Subs., and Immob. Biotech. 1994; 22(3)777–787
- Baldwin A. L., Wilson L. Intravascularly injected hemoglobin produces excess reactive oxygen species in intestinal mucosa. FASEB J. 1998; 12(4)127, A22
- Manohar M., Balasubramaniank A. Glutathione peroxidase contains selenium (Se) as selenocysteine. Indian J. Biochem. Biophys. 1986; 23: 274–278
- Stadtman T. C. Selenium-dependent enzymes. Annu. Rev. Biochem. 1980; 49: 93–110
- Ganther H. E., Hafeman D. G., Lawrence R. A., Serfass R. E., Hoestra W. G. Selenium and Glutathione Peroxidase in Health and Disease-A Review. Trace Elements in Human Health and Disease, A. S. Prasad. Academic Press, New York 1976; 165–234
- Tappel A. L. Lipid peroxidation damage to cell components. Fed. Proc. 1973; 32: 1870–1874
- Simoni J., Simoni G., Garcia E. L., Prien S. D., Tran R. M., Feola M., Shires G. T. Protective effect of selenium on hemoglobin mediated lipid peroxidation in vivo. Art. Cells, Blood Subs., and Immob. Biotech. 1995; 23(4)469–486
- Zikic R. V., Stajn A. S., Ognjanovic B. I., Saicic A. S., Kostic M. M., Pavlovic S. Z., Petrovic V. M. The effect of cadmium and selenium on the antioxidant enzyme activities in rat heart. J. Environ. Pathol., Toxicol. Oncol. 1998; 17(3–4)259–264
- Brown M. W., Watkinson J. H. An automated fluorimetric merthod for the determination of nanogram quantities of selenium. Anal. Chim. Acta 1977; 89: 29–35
- Young M. J., Bresnitz E. A., Strom B. L. Sample-size nomograms for interpreting negative clinical studies. Ann. Intern. Med. 1983; 99: 248–251
- Milici A. J., Bankston P. W. Fetal and neonatal rat intestinal capillaries: permeability to carbon, ferritin, hemoglobin, and myoglobin. Am. J. Anat. 1982; 165: 165–186
- Henderson L. M., Chappell J. B. Dihydrorhodamine 123: a fluorescent probe for superoxide generation?. Eur. J. Biochem. 1993; 217(3)973–80
- Bauer P., Belleville-Nabet F., Watelet F., Dubois F., Larcan A. Selenium, oxygen-derived free radicals, and ischemia-reperfusion injury. An experimental study in the rat. Biol. Trace Elem. Res. 1995; 47: 157–163
- Wolfram S., Arduser F., Scharrer E. In vivo intestinal adsorption of selenate and selenite by rats. J. Nutr. 1985; 115: 454–459
- Barclay R. L., Dinda P. K., Morris G. P., Paterson W. G. Morphological evidence of mast cell degranulation in an animal model of acid-induced esophageal mucosal injury. Dig. Dis. Sci. 1995; 40: 1651–1658
- Aziz E. S., Klesius P. H., Frandsen J. C. Effects of selenium on polymorphonuclear leukocyte function in goats. Am. J. Vet. Res. 1984; 45: 1715–1718
- Aziz E. S., Klesius P. H. Depressed neutrophil chemotactic stimuli in supernatents of ionophore-treated polymorphonuclear leukocytes from selenium-deficient goats. Am. J. Vet. Res. 1986; 47: 140–152
- Kiremidjian-Schumacher L., Stotzky G. Review selenium and immune responses. Environ. Res. 1987; 42: 277–303
- Blakeborough M. H., Owen R. W., Bilton R. F. Free radical generating mechanisms in the colon: their role in the induction and promotion of colorectal cancer?. Free Radical Research Communications 1989; 6(6)359–367
- Grisham M. B., Granger D. N. Neutrophil-mediated mucosal injury. Role of reactive oxygen metabolites. Dig. Dis. Sci. 1988; 33(3 Suppl.)6S–15S
- Stead R. Innervation of mucosal immune cells in the gastrointestinal tract. Reg. Immunol. 1992; 4(2)91–99
- Jourd'heuil D., Morise Z., Connor E. M., Kurose I., Grisham M. B. Oxidant-regulation of gene expression in the chronically inflamed intestine. Keio J. Med. 1997; 46(1)10–15
- Van Pelt L. J., van Zwieten R., Weening R. S., Roos D., Verhoeven A. J., Bolscher B. G.J.M. Limitations on the use of dihydrorhodamine 123 for flow cytometric analysis of the neutrophil respiratory burst. J. Immunol. Methods 1996; 191: 187–196
- Moison R. M.W., van Hoof E. J.H.A., Clahsen P. C., van Zoeren Grobben D., Berger H. M. Influence of plasma preparations and donor red blood cells on the antioxidant capacity of blood from newborn babies: an in vitro study. Acta Paediatr. 1996; 85: 220–224
- Bunn H. F., Jandl J. H. Exchange of heme among hemoglobins and between hemoglobin and albumin. J. Biol. Chem. 1968; 243(3)465–475
- D'Agnillo F., Alayash A. Site-specific modifications and toxicity of blood substitutes. The case of diaspirin cross-linked hemoglobin. Adv. Drug Delivery Rev. 2000; 40(3)199–212
- Royall J. A., Ischiropoulos H. Evaluation of 2′,7′-dichlorofluorescin and dihydrorhodamine 123 as fluorescent probes for intracellular H 2O 2 in cultured endothelial cells. Arch. Biochem. Biophys. 1993; 302: 348–355
- Morrison D. G., Bansal M. P., Kittrell F., Medina D. Distribution of selenium-binding proteins in diferent tissues of the mouse and rat. In Vivo. 1989; 3(3)167–172