Abstract
In strokes, myocardial infarctions, severe sustained hemorrhagic shock, and donor organs, inadequate blood supply results in lack of oxygen to the tissue (ischemia). If ischemia is sustained, reperfusion with the needed oxygen can result in tissue injury (ischemia-reperfusion injury) due to formation of reactive oxygen species. We are studying an oxygen-carrying solution with anitoxidant activity formed by cross-linking hemoglobin, superoxide dismutase, and catalase to form PolyHb-SOD-CAT. The present report studies its effect on the blood-brain barrier and cerebral edema when used in a transient global brain ischemia-reperfusion rat model. We compare this solution to sham-control, oxygenated saline, stroma-free hemoglobin (SF-Hb), polymerized hemoglobin (PolyHb), and a mixture of SF-Hb, SOD, and CAT in free solution. The results show that the cross-linked PolyHb-SOD-CAT solution, unlike the other solutions, can supply oxygen to ischemic tissues without causing reperfusion injury in the transient global brain ischemia-reperfusion model.
INTRODUCTION
Obstruction of blood vessels supplying the brain, due to embolism or arteriosclerosis, can decrease blood flow sufficiently to result in inadequate oxygen supply to the brain (ischemia). Each year, half a million Americans experienced some form of stroke,—80% in the form of ischemic stroke and more than 29% die from the resulting complication Citation[[1]]. Reperfusion of the ischemic brain with oxygen-carrying solutions would supply the needed oxygen, however, this can also result in the formation of reactive oxygen species that can promote tissue injury (ischemia-reperfusion injury) Citation[[2]]. In this study, we analyze the use of a solution formed by polymerizing together hemoglobin, superoxide dismutase, and catalase. The hypothesis we are testing is that this solution may be able to supply oxygen without causing ischemia-reperfusion injury in a rat model of transient global brain ischemia reperfusion.
Cross-linking hemoglobin results in the linking of 3–4 hemoglobin molecules together to form an oxygen-carrying polyhemoglobin solution Citation[3-5]. This product is now in Phase III clinical trials, especially for uses in surgery Citation[5-7]. There could also be a potential use of this product in obstructive ischemia. Being a solution and not particulate red blood cells, it can more easily perfuse through blood vessels partially obstructed by embolism or arteriosclerosis and deliver the required oxygen to the ischemic tissues. However, there is a problem related to reactive oxygen species when such an oxygen-carrying solution is used in ischemia reperfusion (Citation[[3]], Citation[[8]], Citation[[9]]). We, therefore, prepared an oxygen-carrying solution with antioxidant properties. The solution is prepared by cross-linking together hemoglobin, superoxide dismutase, and catalase into a soluble polymer (PolyHb-SOD-CAT) Citation[[9]]. In vivo and in vitro studies show that this solution can carry out the dual function of supplying oxygen and at the same time scavenging harmful reactive oxygen species (Citation[[9]], Citation[[10]]).
Reactive oxygen species have been implicated in the development of neurological disorders and brain dysfunctions after an episode of stroke Citation[11-13]. Superoxide, in particular, has been correlated to the alteration of the blood-brain barrier permeability, edema formation, and neurological cell death (Citation[[14]], Citation[[15]]). Different approaches have been investigated for treating these conditions with varying results Citation[[16]]. Our present study is not for the treatment of ischemia-reperfusion injury after it occurs. We are looking into the use of a suitable oxygen-carrying solution that would not promote the formation of oxygen reactive species and prevent ischemic-reperfusion injury before it occurs.
The present report describes its use as an oxygen carrier with antioxidant properties in a transient global brain ischemia-reperfusion rat model. We follow its effects on the blood-brain barrier and brain edema and compare this solution to sham-control, oxygenated saline, stroma-free hemoglobin (SF-Hb), polymerized hemoglobin (PolyHb) and a mixture of SF-Hb, SOD and CAT in free solution. The results show that the crosslinked PolyHb-SOD-CAT solution, unlike the other solutions, can supply oxygen to ischemic tissues without causing reperfusion injury in the transient global brain ischemia-reperfusion model.
MATERIALS AND METHODS
Materials
Purified ultrapure bovine stroma-free hemoglobin was purchased from Biopure Corporation (Boston, MA). Superoxide dismutase from bovine erythrocytes (EC 1.15.1.1; 4324 U/mg stated activity), catalase from bovine liver (EC 1.11.1.6; 38 080 U/ml stated activity), cytochrome c from horse heart, bovine milk xanthine oxidase, and xanthine were obtained from ICN Biomedicals (Aurora, OH). Glutaraldehyde (111-30-8; 25%), hemoglobin assay kit, molecular distribution standards, and all other analytical-grade reagents were purchased from Sigma-Aldrich (Ontario, Canada).
Cross-linked PolyHb and PolyHb-SOD-CAT
The procedure for cross-linking SF-Hb to obtain PolyHb and PolyHb-SOD-CAT was based on techniques described in D'Agnillo and Chang Citation[[9]] with slight modifications. This is a laboratory procedure; the resulting polyhemoglobin should not be considered as identical to the commercial polyhemoglobins that are now in clinical trials. A hemoglobin-enzyme mixture was prepared to contain hemoglobin (7 g/dl), SOD (21,000 U/dl) and catalase (2,100,000 U/dl) in 0.1 M sodium phosphate buffer (PBS) (pH 7.4). The entire process was conducted in a cold room at 4°C. The mixture was constantly stirred by the motion of an orbital shaker at a speed of 150 rpm. Lysine was added at a molar ratio of 5:1 (Lysine:Hb) prior to the initiation of polymerization. Cross-linking reaction was started with the addition of glutaraldehyde. Glutaraldehyde (5%) was added to the Hb-enzyme-PBS mixture at a rate of 30 μl/min for a period of 16 minutes and at a molar ratio of 17:1 (glutaraldehyde:Hb). After various times (5, 10, or 24 hours), the reaction was stopped with the addition of excess lysine at a molar ration of 100:1 (Lysine:Hb). The PolyHb solution was prepared in similar manner, with the exception that SOD and CAT were added to the hemoglobin solution.
Molecular Weight Distribution
Samples from 5-, 10-, and 24-hour cross-linked PolyHb or PolyHb-SOD-CAT solutions were analyzed by size-exclusion gel filtration liquid chromatography. A Sephadex G-200 column (1.6 cm×70 cm, Vtotal=105 ml), was prepared and equilibrated with 0.1 M Tris-HCl (pH 7.5). Samples (1 ml of 10× dilution) were passed through the column at a rate of 12 ml/hour. The eluting fractions were collected every 15 minutes for a period of 8 hours. The elution profiles were monitored at 280 nm.
SOD and Catalase Activity Assay
SOD activity was based on the reduction of cytochrome c by superoxide Citation[[25]]. Catalase activity was measured by monitoring the decomposition of hydrogen peroxide at 240 nm Citation[[26]]. The eluted fractions from 2.5 to 3.5 hours (≥450 kDa), 3.75 to 5.5 hours (greater than 100 kDa but less than 450 kDa), and 5.75 to 7.5 hours (≤100 kDa), obtained from the size-exclusion chromatography process, were pooled as three separate samples. The samples were then concentrated to 0.7 g/d with the use of a 100 kDa centriplus concentrators (Amicon, Beverly, MA) and then assayed for SOD and catalase activity as described by D'Agnillo and Chang Citation[[14]].
Oxygen-Hemoglobin Dissociation
Analyses of oxygen affinity for SF-Hb, PolyHb, and PolyHb-SOD-CAT were performed with TCS Hemox-Analyzer Model B (Huntingdon Valley, PA). Samples (5 ml) containing 0.3 g/dl of 24-hour cross-linked PolyHb, 24-hour cross-linked PolyHb-SOD-CAT, and ultrapure bovine SF-Hb in 37°C 0.1 M PBS (pH 7.4) were used to obtain oxygen-hemoglobin dissociation curves.
Transient Global Cerebral Ischemia Reperfusion
Global cerebral ischemia was induced by bilateral common carotid arteries (BCCA) occlusion with hemorrhagic hypotension Citation[[27]]. Male Sprague-Dawley rats (235–255 g) were anesthetized with intraperitoneal injection of pentobartbital (Somnotol, 65 mg/kg). Approximately 5 ml of blood were withdrawn from the left femoral-artery cannula to reduce the mean blood pressure to approximately 30–35 mmHg; and the BCCA were temporary occluded with microvascular clips to prevent circulation to the brain. The mean blood pressure was maintained by withdrawn or reinfusion of blood. Preliminary experiments using different durations of ischemia [0 (control), 20, 30, 40 and 60 minutes] were performed and 1 hour of ischemia was chosen for the rest of the experiments. Various preparations of SF-Hb, SF-Hb+superoxide dismutase+catalase, PolyHb, PolyHb-SOD-CAT, and oxygenated saline solutions were subsequently used to perfuse the animals at a rate of 0.8 ml/minute to regain preischemic blood pressure. For the sham control group, the animals were subjected only to the surgical procedure as described above.
Evans Blue Permeability and Brain Water Content Measurement
The structural integrity of the blood-brain barrier and brain edema were examined by techniques as described in Chan et al. Citation[[28]], with the following modifications. One ml of Evans blue (4% in 0.9% saline solution) was administered through the left femoral veins at various experimental time periods after reperfusions and allowed to circulate for 30 minutes prior to the sacrifice of the animals. The animals were then perfused with 5 ml of 0.9% saline at room temperature through the aorta via the left ventricle by peristaltic pump at a rate of 0.8 ml/minute. The brains of the animals were quickly removed from the skulls. The forebrains were weighed and then dried at 105°C for 1 week. The dried brains were reweighed and placed in 2 ml of 0.1 M PBS pH 7.4 for another week. The samples were then homogenized in PBS and centrifuged at 1,000 G for 5 minutes. Samples of 0.7 ml of the supernatants were mixed with equivalent volume, 0.7 ml, of 12% (w/v) trichloroacetic acid (to precipitate proteins). The mixtures were incubated at 4°C for 18 hours, then centrifuged at 1,000 G for 30 minutes. The Evans blue concentration of the supernatants was quantitatively measured at 610 nm for absorbance.
The water content for each forebrain was calculated as the difference between the forebrain wet weight and dry weight. The changes in water content (per forebrain), as presented in , were calculated as the differences between the forebrain water contents obtained from 1-, 2-, 4-, and 6-hour groups, and that of the 0-hour group.
RESULTS
Molecular Weight Distribution
The molecular weight distributions for PolyHb and PolyHb-SOD-CAT (5-, 10-, and 24-hours cross-linking) show three molecular components (low, intermediate, and high molecular weight) (). Tetrameric hemoglobins were eluted in the molecular range of less than 100 kDa. The intermediate molecular weight ranged between 100 kDa and 450 kDa. The high molecular components were greater than 450 kDa. Non–cross-linked tetrameric hemoglobin shows only the low molecular component (less than 100 kDa).
Figure 1. Molecular distribution of cross-linked and non–cross-linked ultrapure bovine SF-Hb. Elution profiles of 5-, 10-, 24-hour cross-linked PolyHb, PolyHb-SOD-CAT and non–cross-linked bovine SF-Hb were obtained by running 1 ml of the 10× diluted samples on Sephadex G-200 1.6 cm×70 cm column (VT=105.5 ml, Vo=38 ml, equilibrated with 0.1 M Tris-HCl, pH 7.5, and eluted at 11.5 ml/hr).
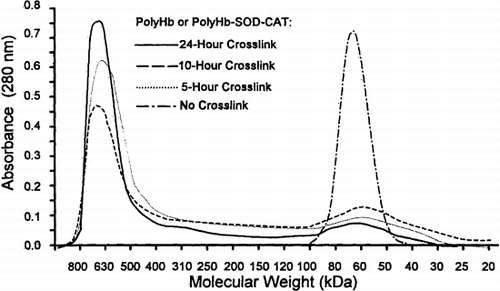
Ultrafiltration led to a decrease in tetrameric hemoglobin concentration (). Furthermore, the filtered 24-hour cross-linking samples yielded the highest percentage (77%) of molecular component with ≥450 kDa and the lowest percentage (7%) of product with ≤100 kDa. The remaining 16% was intermediate, with molecular weight between 100 kDa and 400 kDa.
Table 1. Molecular Components of PolyHb and PolyHb-SOD-CAT
SOD and Catalase Activity
SOD and catalase activity assays from the collected fractions of the 24-hour cross-linked PolyHb-SOD-CAT and PolyHb samples showed that PolyHb-SOD-CAT retained enzymatic activity after polymerization, while PolyHb had no enzymatic activity. summarized the SOD and catalase enzymatic activity after polymerization and ultrafiltration.
Table 2. Molecular Distribution and SOD and CAT Activity
Most of the SOD and catalase activity (83±3% and 82±2%, respectively) are found in the PolyHb-SOD-CAT samples with a molecular weight range of ≥450 kDa. Only 7±2% and 11±1% of SOD and catalase activity, respectively, were in the components with molecular weight between 100 kDa and 450 kDa. The lowest SOD and catalase activity (4±1% and 5±1%, respectively) were found in elutions representing molecular component of ≤100 kDa.
Oxygen-Hemoglobin Dissociation
Oxygen-hemoglobin dissociation analysis was determined for the 24-hour cross-linked PolyHb (n=6), the 24-hour cross-linked PolyHb-SOD-CAT (n=6) and the ultrapure bovine SF-Hb (n=6) (). The P50 value of PolyHb was found to be 26.1±0.2 mmHg, while that of PolyHb-SOD-CAT was 24.7±0.2 mmHg and ultrapure SF-Hb was 27.8±0.0 mmHg.
Figure 2. Oxygen-hemoglobin dissociation. The 24 hours of cross-linking with glutaraldehyde did not significantly affect the P50 value of SF-Hb. PolyHb and PolyHb-SOD-CAT P50 values were 26.1±0.2 mmHg and 24.7±0.2 mmHg, respectively. The SF-Hb P50 value was 27.8±0.0 mmHg. All oxygen-hemoglobin dissociation curves are presented as averages of 6 trials (n=6).
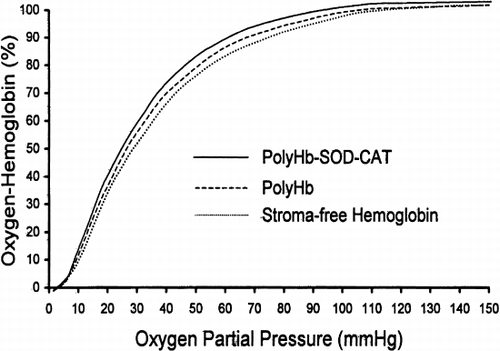
Baseline Study on the Effects of Duration of Ischemia Before Reperfusion as Measured by Evans Blue Extravasation
Evans blue extravasation of forebrain tissue was determined for 4 groups of animals (sham control, oxygenated saline, SF-Hb, and PolyHb). Solutions were infused (reperfusion) after 0, 20, 30, 40, or 60 minutes of ischemia (). There was no significant elevation in Evans blue content (18.1±1.0 μg to 21.4±3.1 μg) as ischemia was increased from 0 to 60 minutes in the sham control group. Additionally, there were no significant differences in Evans Blue content between the sham group and ischemic groups for 0, 20, and 30 minutes of ischemia.
Figure 3. Determining the required ischemic duration. Animals were subjected to either 0, 20, 30, 40, or 60 minutes of ischemia before reperfusion. With 60 minutes of ischemia, the level of Evans blue in the sham control was significantly lower than that of oxygenated saline, stroma-free Hb (SF-Hb), and PolyHb. Statistical significance is P<0.01.
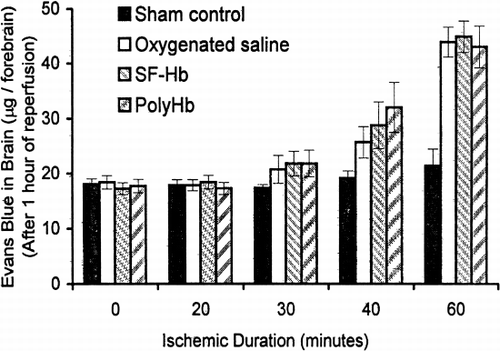
When reperfusion was carried out 40 minutes after ischemia, there were significant differences in Evans blue extravasation between the sham control group (19.2±1.3 μg), oxygenated saline (25.7±2.8 μg), SF-Hb (28.8±4.2 μg), and PolyHb (32.0±4.5 μg). When reperfusion took place after 60 minutes of ischemia, there were further increases in the differences in Evans blue content between the sham and test groups. Evans blue content in the groups that were reperfused with oxygenated saline, SF-Hb, and PolyHb (44.0±2.8 μg, 45.0±2.9 μg and 43.1±3.8 μg, respectively) was significantly higher than the sham control group (21.4±3.1 μg). There was no significant difference between the three reperfused groups. Based on these results, we selected 60-minute ischemia before reperfusion for all subsequent studies.
Ischemia Reperfusion Studies Using Different Solutions
After 60 minutes of global brain ischemia, the animals were reperfused using different solutions. Influx of Evans blue into cerebral tissues was analyzed at 0, 1, 2, 4, and 6 hours after ischemia reperfusion (). The initial Evans blue measurements at the zero-hour mark for PolyHb (17.7±1.3 μg), SF-Hb (17.2±1.1 μg), SF-Hb+SOD+CAT (17.4±1.1 μg), oxygenated saline (18.4±1.2 μg), and PolyHb-SOD-CAT (17.4±1.1 μg) treated group were not significantly different from the sham control group (17.8±1.0 μg).
The sham control group exhibited no significant elevation of Evans blue (19.2±1.3 μg) during the 6 hours of study (). For PolyHb-SOD-CAT, the maximal level was 30.9±2.3 μg, much lower than for the other solutions. The use of the other solutions, oxygenated saline, SF-Hb, PolyHb, or the Hb+SOD+CAT mixture resulted in steady increase in Evans blue. Thus, two hours after reperfusion, the Evans blue levels were 48±2 μg for SF-Hb+SOD+CAT; 47±2 μg for oxygenated saline; 54±4 μg for PolyHb; and 54±6 μg for SF-Hb. For SF-Hb+SOD+CAT the Evans blue levels increased to 53±1 μg by the forth hour and to 56±4 μg by the sixth hour ().
Changes in Cerebral Water Content
There were no significant differences in cerebral water contents between the sham control and PolyHb-SOD-CAT groups throughout the entire six hours. However, for the other four solutions the cerebral water contents steadily increased for the entire 6 hours ().
Figure 5. Brain edema: changes in brain water content. The changes in brain water content of PolyHb-SOD-CAT–treated animals were not significantly different from that of the sham control. The increase in water contents of saline, SF-Hb, SF-Hb+SOD+CAT, and PolyHb were significantly different from that of the sham control and PolyHb-SOD-CAT group by the 4th hour and increased with time. Statistical significance is P<0.01.
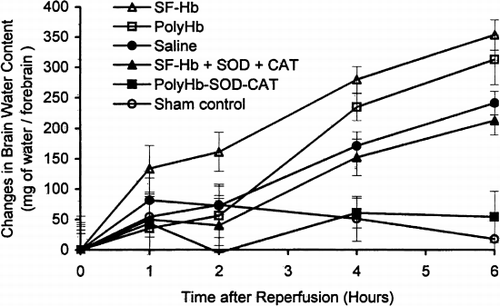
SF-Hb group exhibited significantly more water influx into the forebrain than the other groups by the end of the second hour with an increase of 161.3±32.1 mg. By the fourth hour following reperfusion, the cerebral water content of the animals continued to increase, reaching 234.8±21.9 mg for PolyHb, 279.8±21.8 mg for SF-Hb, 152.3±30.2 mg for SF-Hb+SOD+CAT, and 171.3±23.0 mg for oxygenated saline. Cerebral water content continued to increase by the 6th hour, reaching 313.3±41.1 mg for PolyHb; 354.5±25.4 mg for SF-Hb; 213.3±23.8 mg for SF-Hb+SOD+CAT; and 241.8±19.3 mg for the oxygenated saline group. The increase in cerebral water content of the PolyHb and SF-Hb group was significantly higher than the SF-Hb+SOD+CAT and oxygenated saline groups. However, they were all significantly higher than the sham control. The polymerized PolyHb-SOD-CAT solution is the only one that is not significantly different when compared to the sham control.
DISCUSSION
Several preclinical studies in animal stroke models show that crosslinked hemoglobin has the ability to deliver oxygen to ischemic tissue Citation[[18]]. Later studies in humans, however, have also showed that this may result in adverse effects Citation[[24]], which could very likely be explained by ischemia-reperfusion injuries caused by reactive oxygen species. Reactive oxygen species (superoxide in particular) are involved in pathogenesis of brain edema and microvascular abnormality following cerebral ischemia reperfusion (Citation[[20]], Citation[[21]]).
The present study shows that a solution consisting of polymerized Hb, SOD, and CAT has the ability to scavenge reactive oxygen species (particularly superoxide and hydrogen peroxide, in vitro) and the capability to carry oxygen. Additionally, this investigation indicates that the use of PolyHb-SOD-CAT solution in a transient global brain ischemia-reperfusion injury model leads to less disruption of the blood-brain barrier as compared to the uses of other oxygen-carrying solutions (particularly PolyHb solution).
Our study also demonstrates that cross-linked ultrapure hemoglobin, PolyHb, and PolyHb-SOD-CAT exhibit similar oxygen-carrying characteristic. Furthermore, the P50 values of PolyHb and PolyHb-SOD-CAT solution are not significantly different. This suggests that cross-linking SF-Hb with SOD and catalase has minimal effect on the oxygen affinity of the polymerized product, PolyHb-SOD-CAT. Additionally, enzymatic assays reveal that SOD and catalase retained their respective enzymatic activities even after cross-linking, and that the highest oxygen radical scavenging activities were present in fractions representing molecular components that were greater than 450 kDa.
Analysis of the Evans blue extravasation and brain-water content data suggests that the uses of oxygen-carrying solutions in cerebral ischemia reperfusion result in the disruption of the blood-brain barrier and increase in brain edema. However, the Evans blue level in cerebral tissue is significantly low with the uses of PolyHb-SOD-CAT as compared to PolyHb, SF-Hb, SF-Hb+SOD+CAT, and oxygenated saline solution. Interestingly, it is noted that the administration of SF-Hb solution containing nonpolymerized SOD and CAT suppresses the elevation of Evans blue influx into the cerebral tissue in a manner similar to PolyHb-SOD-CAT. This occurrence, however, is observed only during the first hour after reperfusion. After this period, the Evans blue level increases for next 5 hours, reaching the same level as that of PolyHb, SF-Hb, and oxygenated saline solution. The transient protective effect noted during the first hour after the administration of SF-Hb with free native SOD and catalase is most likely due to the short half-life of free SOD and catalase (approximately 4–6 minutes and 15 minutes, respectively) in the circulation Citation[[9]]. Similar results are also detected in the brain-edema measurements in the present study.
Numerous studies have suggested that superoxide radicals, formed from single-electron reduction of oxygen, are involved in edema formation Citation[[22]]. Superoxide anions are produced by endothelial cells of the vessel walls and escaped into the extracellular space Citation[[20]]. Additionally, xanthine oxidase, the primary enzyme involved in the formation of superoxide during ischemia-reperfusion, is reported to have high activity in the capillaries of the blood-brain barrier compartment Citation[[23]]. The existence of xanthine oxidase in the brain capillaries enhances the formation of superoxide, which can interact with nitric oxide and produce peroxynitrile (ONOO−) Citation[[24]]. Peroxynitrile, consequently, leads to the disruption of the blood-brain barrier and edema formation. Hence, it is believed that PolyHb-SOD-CAT may exert its effect on this pathway by scavenging the superoxide anions and preventing the interaction of superoxide anions with nitric oxide. However, the present study does not provide a direct link to this pathway. Future comparative studies, which are designed to examine levels of superoxide and nitric oxide in the vicinity of cerebral vessels, may further support the viewpoint presented here. The present study, using Evans blue, measures the functional disruption of blood-brain barrier. An electron microscopic examination of the blood-brain barrier tight junctions would be useful as additional evidence.
In conclusion, our present study is not designed to suggest a treatment for ischemia-reperfusion injury. We are merely studying the use of a suitable oxygen-carrying solution that would not cause the disruption of blood-brain barriers under conditions of cerebral ischemia reperfusion. As such, the PolyHb-SOD-CAT solution seems to be able to act as an oxygen carrier and at the same time markedly decreases blood-brain barrier disruption and brain edema in this model.
ACKNOWLEDGMENTS
T.M.S.C. acknowledges the operating grant support from the Canadian Institute of Health Research and the Virage Centre of Excellence award from the Quebec Ministry of Higher Education, Science, and Technology. D.D.P. gratefully acknowledges the FCAR graduate studentship from Fonds pour la Formation de Chercheurs et l'Aide a la Recherche.
REFERENCES
- Heart and Stroke Facts: 1994 Statistical Supplement. American Heart Association, Dallas, Texas 1993
- Juurlink B. H.J., Sweeney M. I. Mechanisms that result in damage during and following cerebral ischemia. Neurosci. Biobehav. Rev. 1997; 21: 121–128
- Chang T. M.S. Red blood cell substitutes. Best Pract. Res. Clin. Haematol. 2000; 13: 651–668
- Gould S. A., Moss G. S. Clinical development of human polymerized hemoglobin as a blood substitute. World J. Surg. 1996; 20: 1200–1207
- Greenburg A. G., Kim H. W. Use of donor blood in the surgical setting potentials for application of blood substitutes. Artif. Cells Blood Substitute Immobilization Biotechnol. 2000; 1(1–2)25–29
- Gould S. A., et al. The first randomized trial of human polymerized hemoglobin as a blood substitute in acute trauma and emergent surgery. J. Am. Coll. Surg. 1998; 187: 113–120
- LaMuraglia G. M., et al. The reduction of the allogenic transfusion requirement in aortic surgery with a hemoglobin-based solution. J. Vasc. Surg. 2000; 31: 299–308
- Alayash A. L. Hemoglobin-based blood substitutes: oxygen carriers, pressor agents, or oxidants?. Nat. Biotechnol. 1999; 17: 545–549
- D'Agnillo F., Chang T. M.S. Polyhemoglobin-superoxide dismutase-catalase as a blood substitute with antioxidant properties. Nat. Biotechnol. 1998; 16: 667–671
- D'Agnillo F., Chang T. M.S. Absence of hemoprotein-associated free radical events following oxidant challenge of crosslinked hemoglobin-superoxide dismutase catalase. Free Radic. Biol. Med. 1998; 24: 906–912
- Kontos H. A. Oxygen radicals in cerebral vascular injury. Circ. Res. 1985; 57: 508–516
- Siesjo B. K., Agardh C. D., Bengtsson F. Free radicals and brain damage. Cerebrovasc. Brain Metab. Rev. 1989; 1: 165–211
- Chan P. H., Fishman R. A., Wesley M. A., Longar S. Pathogenesis of vasgenic edema in focal cerebral ischemia: role of superoxide radicals. Adv. Neurol. 1990; 52: 177–182
- Halliwell B., Gutteridge J. M.C. Oxygen toxicity, oxygen radicals, transition metals and disease. Biochem. J. 1984; 219: 1–14
- Chan P. H. Oxygen radical in focal cerebral ischemia. Brain Pathol. 1994; 4: 59–65
- Chan P. H., et al. Neuroprotective role of CuZn-superoxide dismutase in ischemic brain damage. Adv. Neurol. 1996; 71: 271–280
- Cole D. J., Schell R. M., Przybelski R. J., Drummond J. C., Bradley K. Focal cerebral ischemia in rats: effect of hemodilution with alpha-alpha cross-linked hemoglobin on CBF. J. Cereb. Blood Flow Metab. 1992; 12: 971–976
- Bowes M. P., Burhop K. E., Zivin J. A. Diaspirin cross-linked hemoglobin improves neurological outcome following reversible but not irreversible CNS ischemia in rabbits. Stroke 1994; 25: 2253–2257
- Saxena R., et al. Controlled safety study of a hemoglobin-based oxygen carrier, DCLHb, in acute ischemic stroke. Stroke 1999; 30: 993–996
- Kontos H. A., et al. Appearance of superoxide anion radical in cerebral extracellular space during increased prostaglandin synthesis in cats. Circ. Res. 1985; 57: 142–151
- Hall E. D., Braughler J. M. Central nervous system trauma and stroke. II. Physiological and pharmacological evidence for involvement of oxygen radicals and lipid peroxidation. Free Radic. Biol. Med. 1989; 6: 303–313
- Villacara A., Kempski O., Spatz M. Arachidonic acid and cerebromicrovascular endothelial permeability. Adv. Neurol. 1990; 52: 195–201
- Betz A. L. Identification of hypoxanthine transport and xanthine oxidase activity in brain capillaries. J. Neurochem. 1985; 44: 574–579
- Oury T. D., Piantadosi C. A., Crapo J. D. Cold-induced brain edema in mice: Involvement of extracellular superoxide dismutase and nitric oxide. J. Biol. Chem. 1993; 268(21)15394–15398
- Flothe L., Otting F. Superoxide dismutase assays. Methods Enzymol. 1984; 105: 93–104
- Aebi H. Catalase in vitro. Methods Enzymol. 1984; 105: 121–126
- Smith M. L., et al. Models for studying long-term recovery following forebrain ischemia in the rat. A 2-vessel occlusion model. Acta Neurol. Scand. 1984; 69: 385–401
- Chan P. H., Yang G. Y., Chen S. F., Carlson E., Epstein C. J. Cold-induced brain edema and infarction are reguced in transgenic mice over expressing CuZn-superoxide dismutase. Ann. Neurol. 1991; 29: 482–486