Abstract
A functional requirement for all hemoglobin-based oxygen carriers (HBOCs) is the maintenance of the heme-iron in the reduced state. This is necessary for the reversible binding/release of molecular oxygen and minimization of methemoglobin (Fe+3) formation. Acellular hemoglobins are especially susceptible to oxidation and denaturation. In the absence of the intrinsic reducing systems of the red blood cell, the reduced heme-Fe+2 can be oxidized to form increasing levels of methemoglobin that can give rise to free radicals and oxidative cellular damage. If acellular HBOCs are to be utilized as red cell substitutes for oxygen delivery, these carriers must be stabilized in the plasma, the carrier medium. Normal plasma contains reducing components, such as ascorbic acid and glutathione, that can afford protection to these acellular HBOCs through electron-transfer mediated processes. For these components to provide effective reduction to an HBOCs, a favorable reduction potential difference must exist between the reducing agent and the HBOCs. Using a modified thin-layer spectroelectrochemical method, a determination of the formal reduction potential (vs. Ag/AgCl) of several oxygen carriers, including monomeric myoglobin, tetrameric HbA and HbS, chemically cross-linked HbXL99α, polymerized oxyglobin (FDA approved for canine anemia), and the natural cross-linked polymeric Lumbricus hemoglobin, have been determined. In contrast to the negative formal reduction potentials (−155 to −50 mV) obtained for Mb, HbA, HbS, HbXL99α, and oxyglobin, Lumbricus hemoglobin exhibited a positive formal reduction potential (~100 mV). These results may help explain the greater effectiveness of the tested reducing agents to reduce met Lumbricus hemoglobin, compared to the other HBOCs, back to the required reduced form necessary for physiological binding/release of oxygen. Each reducing agent was capable of reducing met Lumbricus hemoglobin to the fully reduced state, although the kinetics of these reactions were different. HbA, HbXL99α, and oxyglobin were only partially reduced (10 to 37%) by glutathione, β-NADH, and ascorbic acid under similar conditions.
INTRODUCTION
An essential physiological requirement for all therapeutic hemoglobin-based oxygen carriers (HBOCs) is the maintenance of the heme-iron in the reduced state (Fe+2). This is necessary for the reversible binding-release of molecular oxygen. Methemoglobin (heme-Fe+3), which can be generated either through autoxidation or the interaction with exogenous oxidizing compounds, is unable to bind oxygen and may lead to hemichrome formation coupled with the formation of oxidative free radicals in vivo Citation[1-3].
The human erythrocytic internal environment is advantageous because it contains enzymatic and nonenzymatic components for controlling the functional redox state of the hemoglobin molecule inside the red cell. The recent design and use of several different acellular HBOCs in the absence of such intrinsic red cell reducing systems have been shown to undergo rapid oxidation under in vivo conditions Citation[4-6]. Since human plasma lacks the enzymatic protective mechanisms to effectively maintain these hemoglobins in the reduced form, it is crucial that any acellular HBOCs circulating in the plasma be resistant to oxidative stress and minimize methemoglobin levels.
Human plasma does, however, contain low concentrations of certain nonenzymatic reducing compounds, such as glutathione and ascorbic acid. These have the potential for maintaining the necessary reduced form of the HBOCs being utilized as an oxygen carrier. Previous in vitro studies, evaluating the effectiveness of these agents in reducing methemoglobin, clearly demonstrated that not all HBOCs were equally protected from oxidation. Interestingly, when the metHb form of HbA, HbXL99α, oxyglobin, and the naturally polymerized and cross-linked giant hemoglobin of the earthworm Lumbricus terrestris (LtHb), were reacted with glutathione, ascorbic acid, and β-NADH, only the LtHb showed significant reduction to the oxyHb form. Differences in the kinetics of reduction by these compounds were observed (Citation[[7]], Citation[[8]]).
In an attempt to elucidate the redox events between the different HBOCs and the specific human plasma reducing compounds, the formal reduction potential of each HBOCs has been determined using a modified form of thin-layer spectroelectrochemistry. These experiments have been analyzed in conjunction with the kinetics of metHb reduction of each HBOCs by these reducing compounds. The combined results clearly indicate that any proposed acellular hemoglobin-based oxygen carrier designed should have a favorable (positive) redox potential in order to maintain the HBOCs in the functionally important reduced state for oxygen-binding and delivery.
MATERIALS AND METHODS
Isolation and Purification of Hemoglobins
HbA-Blood samples from normal individuals (AA) were made available from the Comprehensive Sickle Cell Center at the University of South Alabama Medical Center. Each sample of normal adult blood was centrifuged to separate blood cells and plasma components. The packed red cells were washed three times in an isotonic saline solution (0.9%). The cells were lysed with cold deionized water. Membrane components were removed after high-speed centrifugation. The soluble hemoglobin was centrifuged at least two additional times to remove any precipitated material. The total lysate was equilibrated to pH 8.4 on a Sephadex G-25 column. Isolation and purification of HbA were carried out by anion-exchange chromatography (DEAE-52) eluted with a 50 mM Tris-HCl, pH 8.4 buffer.
Lumbricus terrestris Hb-Live earthworms (10–20 cm) were obtained from Ward's Biological Company, Rochester, NY. The coelomic fluid of each worm was drained into a cold (~1°C) 100 mM potassium phosphate buffer, pH 7.0. After the initial centrifugation at 10,000 rpm for 30 minutes to remove any cellular debris, the supernatant was removed and centrifuged at 160,000 g for 3 hours at 4°C. The red pellet was redissolved in buffer and the ultracentrifugation step was repeated. This red pellet was redissolved in a minimum amount of buffer to maintain a high stock concentration of this hemoglobin. The purified hemoglobin was used immediately according to the experimental situation or stored in pellet form under liquid nitrogen until required for use. Storage under liquid nitrogen prevents autoxidation. No changes in the properties of this hemoglobin were evident with these stored preparations over at least one year as determined by spectroscopic and light scattering measurements Citation[[9]].
Highly purified HbXL99α was made available for our work through a generous gift of the U.S. Army/MMC (Dr. Victor MacDonald). The Oxyglobin sample was purchased directly from Biopure, Cambridge, MA, and used as received.
Reduction Studies-Direct Spectroscopic Analysis
The rate and extent of all hemoglobin reduction reactions were carried out in sodium phosphate (100 mM, pH 7.0) buffer solution. All HBOCs were completely oxidized (100% metHb) by reacting each sample with an excess of K3Fe(CN)6. Each oxidized sample was eluted on a Sephadex G-25 column to remove the unreacted potassium ferricyanide. The reduction reactions were initiated by the direct addition of either glutathione, ascorbic acid, or β-NADH to give the required concentration of reducing agent. Using direct absorption analysis, spectra were recorded in an overlay mode within the visible region (700–490 nm) as a function of time. The rate and extent of hemoglobin reduction was determined either by the increase in the A576/A540 ratio or by the decrease in the charge-transfer band at 630 nm. Spectral and kinetic measurements were obtained using either a Varian 2290 or Shimadzu 2501 recording spectrophotometer equipped with a thermostatically controlled multicell compartment.
Redox Studies-Spectroelectrochemical Analysis
An optically transparent thin-layer electrochemical cell similar to that described by Faulkner and coworkers (Citation[[10]], Citation[[11]]) was used. Since the heme-iron center in the hemoglobin is not accessible for direct electron transfer from the working electrode due to the unique secondary and tertiary structure of hemoglobin, a mediated electron transfer process must be utilized for these reactions. This small mediator molecule is reduced or oxidized at the electrode surface and undergoes diffusion to the heme site where it in turn reduces or oxidizes the heme-iron center. Upon rediffusion back to the electrode surface, the mediator can be reoxidixed or re-reduced and the cycle is continued. In our experiments, the mediator, Ru(NH3)6Cl3, was used in order to compare our results with other studies (Citation[[12]], Citation[[13]]).
The advantage of using thin-layer electrochemistry is that the electroactive species quickly conform to the Nernst equation:
After the cell was assembled and placed into the spectrophotomer, a series of potentials at specified intervals were applied to the platinum minigrid electron. An absorbance spectrum was obtained at each potential after 20 minutes equilibration. The ratio [Ox]/[Red] was determined by measuring [A−Ared]/[Aox−A] where Ared and Aox are the absorbances of the totally reduced and oxidized forms respectively, and A is the absorbance of the intermediate forms.
RESULTS
The use of thin-layer spectroelectrochemistry has permitted a determination of the importance of the formal reduction potential of several hemoglobin-based oxygen carriers (HBOCs). In the reduction potential of myoglobin, a monomeric heme oxygen binding and storage protein, was measured in order to determine the reliability of our method. The formal reduction potential (E°′) as measured against the a reference Ag/AgCl electrode was determined to have an E°′=−155 mV comparable to a previously reported value of −160 mV (Citation[[10]], Citation[[11]]). The corresponding Nernst plot for myoglobin exhibited excellent linearity with the slope of 0.95, the expected value given the monomeric and noncooperative oxygen binding characteristic of this protein. In turn, the formal reduction potentials of other hemoglobin-based oxygen carriers such as tetrameric HbA and HbS, chemically cross-linked HbXL99α, polymerized oxyglobin (FDA approved for treatment of canine anemia), and the naturally available multimeric Lumbricus hemoglobin were also measured under identical experimental conditions. presents a summary of these results. In contrast to the negative formal reduction potentials (−155 to −23 mV) obtained for Mb, HbA, HbS, HbXL99α, and oxyglobin, Lumbricus hemoglobin exhibited a positive formal reduction potential (112 mV).
Figure 1. (Top) Absorbance spectra of myoglobin at differing applied potentials (0.2 V to −0.6 V). Fully oxidized Mb is indicated by the maximum at 408 nm and the fully reduced Mb is indicated by the maximum at 434 nm. Redox measurements were carried out in 100 mM MOPS buffer, pH 7.0, 0.5 mM mediator, and 200 mM NaCl at 20°C. (Bottom) Nernst plot for myoglobin. All potentials were measured against Ag/AgCl reference electrode.
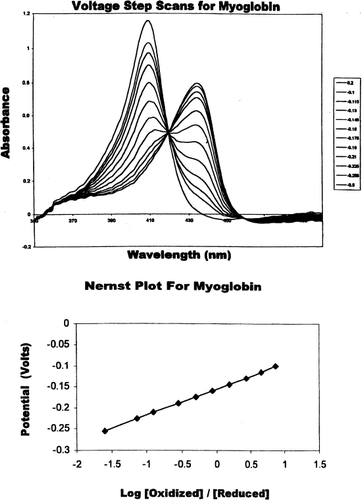
Table 1. Formal Redox Potentials of Hemoglobin-Based Oxygen Carriers by Thin-Layer Spectroelectrochemistry (T=20°C)Footnotea
In contrast to myoglobin, tetrameric and multimeric hemoglobins have complex redox systems associated with the heme-heme interactions involved in cooperative oxygen binding. This was particularly evident in wherein the experimentally altered potential step spectra and Nernst plot for Lumbricus hemoglobin are presented. The formal reduction potential, determined to be E°′=+112 mV, was coupled with a nonlinear Nernst plot wherein n is determined to greater than 1.0, implying cooperativity at the electron transfer level as well.
The formal reduction potentials for tetrameric HbA and HbS (β6Glu→Val) were almost identical (−52 and −50 mV, respectively). The single-point mutation of a surface residue did not appear to affect the inherent reduction potential of HbS. No structural differences within the heme environment are evident from crystallographic studies of these two hemoglobin molecules. On the other hand, the chemically cross-linked form of adult human hemoglobin defined as HbXL99α (linkage between the Lysine 99 residues of the α chain) exhibited a slightly more positive reduction potential (−23 mV). Unfortunately, this sample was quite unstable during the electrochemistry run and resulted in slightly turbid solutions during each run. Oxyglobin (a glutaldehyde cross-linked polyermized form of bovine hemoglobin) was found to have a similar reduction potential (−48 mV) to that of HbA and HbS (). This HBOCs can be fractionated on a gel filtration column into a discrete polymeric hemoglobin and a tetrameric fraction. The polymeric hemoglobin exhibited a reduction potential of −47 mV, similar to the unfractionated mixture of oxyglobin.
Figure 2. (Top) Absorbance spectra of Lumbricus hemoglobin at differing potentials (0.2V to −0.6 V). Fully oxidized LtHb is indicated by the maximum at 396 nm and the fully reduced LtHb is indicated by the maximum at 430 nm. Solutions conditions were similar to myoglobin. (Bottom) Nernst plot of Lumbricus hemoglobin. All potentials were measured against Ag/AgCl reference electrode.
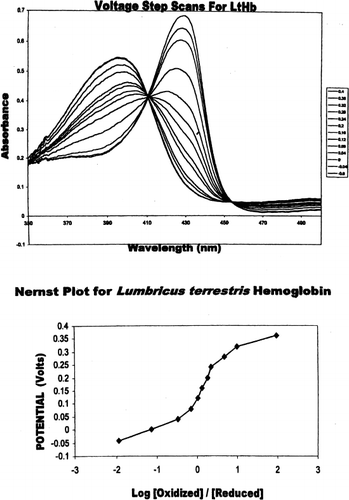
Although it has been previously demonstrated that glutathione and β-NADH can reduce metLHb slowly Citation[[7]], ascorbic acid was rapid and effective in the reduction of the multimeric metLtHb as evident from and . In contrast to HbA, HbXL99α, and oxyglobin, Lumbricus Hb was completely reduced to oxyhemoglobin within a short time period (~90 minutes). HbXL99α and HbA were reduced to about 45% within 270 and 240 minutes, respectively. No other HBOCs used in this study was completely reduced to oxyhemoglobin within similar periods of time investigated.
Figure 3. The reduction of the metHb forms of HbXL99α (A) Lumbricus Hb (B), and HbA (C) by 1 mM ascorbic acid at 20°C. Continuous spectra were recorded at specified time intervals (minutes). The rate of appearance of the 540 and 576 nm peaks reflect the conversion of Fe+3-heme→Fe+2-heme for each of hemoglobin sample.
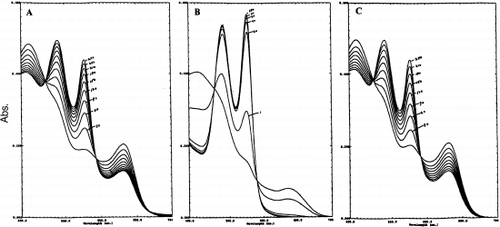
Table 2. Role of Ascorbic Acid in the Reduction (heme-Fe+3→heme-Fe+2) of Hemoglobin-Based Oxygen Carriers at T=20°C, pH 7.0 (% reduction)
DISCUSSION
From hemoglobinopathies and site-specific mutational studies of myoglobin and hemoglobin variants, it is evident the unique molecular modifications associated with amino acid sequence differences are at the center of hemoglobin's ability to function within a wide range of organisms and under different physiological conditions Citation[14-16]. These unique differences within the globin sequences, and especially within the heme pocket, have produced alterations in the oxygen binding properties, allosteric behavior, and, for many hemoglobins, in redox stabilities. This latter alteration is of major concern for any acellular HBOCs design proposed or utilized as a substitute for the delivery of oxygen in place of red blood cells.
Acellular hemoglobins are particularly susceptible to oxidation and denaturation. Human plasma, the carrier medium, lacks the enzymatic protective mechanisms to effectively maintain hemoglobin in the required reduced state (heme-Fe+2). In the absence of any intrinsic enzymatic reducing systems, the reduced heme-Fe+2 autoxidizes to form increasing levels of methemoglobin that give rise to free radicals and oxidative cellular damage in vivo. Plasma does, however, contain levels of ascorbic acid and glutathione that may serve as reducing agents when an acellular HBOCs is used in plasma either under transfusion or anemic conditions.
Over the years much effort has gone into the elucidation of the nature of the cooperative interactions that are associated with the regulation of molecular oxygen binding to tetrameric hemoglobin. Several comprehensive reviews are available which describe in detail the molecular events associated with the binding of oxygen to the hemoglobin molecule Citation[17-19]. In contrast, little is known about the linkages between this binding process, the inherent tendency of acellular hemoglobin (HBOCs) electron loss (heme oxidation) coupled to its loss of oxygen binding capacity, and the role of electron donating agents of the plasma to maintain the necessary redox state of the heme-iron for biological viability. Thermodynamically for an endogenous plasma reducing component to provide effective reduction protection to a specific HBOCs, a favorable reduction potential difference must exist between the reducing agent and the HBOCs. The assumption that all HBOCs presently in clinical trials, or in the developmental stage, will be afforded equivalent reducing protection within human plasma by these endogenous reducing compounds has not been investigated.
A modified thin-layer spectroelectrochemical method Citation[10-12] has permitted the determination of the formal reduction potentials for several HBOCs, assessing their resistance to oxidation (heme-Fe+2→heme-Fe+3) or capacity for reduction (heme-Fe+3→heme-Fe+2). In order to verify the intrinsic validity of this approach, the reduction potential of myoglobin, a single-chain heme oxygen binding and carrier protein, was measured (). Its formal reduction potential (E°′) as measured against a reference Ag/AgCl electrode was determined to have an E°′=−155 mV comparable to a previously reported value of −160 mV (Citation[[10]], Citation[[11]]). As expected, the corresponding Nernst plot for myoglobin exhibited linearity (slope, n=0.95), the expected n value for a single-chain and noncooperative oxygen binding protein ().
This behavior was in marked contrast to the formal reduction potentials of the other hemoglobin-based oxygen carriers investigated in this study which included tetrameric HbA and HbS, chemically cross-linked HBXL99α, polymerized oxyglobin (approved for canine anemia treatment), and the natural cross-linked polymeric giant Lumbricus terrestris hemoglobin (a dodecamer, 4×106 Da molecular weight, 144 hemes). In contrast to the negative formal reduction potentials (−155 to −23 mV) as seen for Mb, HbA, HbS, HbXL99α, and oxyglobin (bovine Hb based polymer), Lumbricus hemoglobin exhibited a positive reduction potential (+112 mV). This formal reduction potential was coupled to a nonlinear Nernst plot with an n value greater than 1.0 (). The large number of heme-heme interactions evident during oxygen binding (Hill coefficient, n=5.6–7.8) for Lumbricus Hemoglobin Citation[[20]] are reflected in this nonlinear plot () and may be a reflection of a high level of cooperativity between these heme moieties during the electron transfer process as well. Unfortunately, the complex nature of the multicentered redox system does not allow for a determination of electron transfer stoichiometry. The well defined isosbestic points at 412 nm and 454 nm LtHb () clearly indicated the presence of only two discrete molecular species in equilibrium during the redox events. This has permitted a reasonable estimate of the formal reduction potential under these solution conditions.
The positive reduction potential for LtHb may be indicative of significant differences in the nature of the heme environment and/or the extent of the heme moiety's solvent-surface exposure. In an earlier systematic study of several heme proteins, Stellwagen Citation[[21]] had determined that the exposure of the heme moiety, rather than the apolarity or nature of the heme environment was the principal factor in determining the magnitude of the reduction potential for a particular heme protein. Experimental work to establish the overall three-dimensional structure of Lumbricus hemoglobin has been underway in several laboratories for the past two decades. Recently, the x-ray studies of Royer and coworkers Citation[[22]] have provided a 5.5 A resolution of the hierarchical structural organization of this large multimeric acellular hemoglobin molecule. Using this hierarchical model, the structure of the dodecameric subunit organization of the heme-containing chains has been further refined by computational and modeling analysis down to a 3.0 A resolution Citation[[23]]. A determination of the extent of the heme solvent-surface exposure (four different heme-containing chains) within this functional dodecameric subunit is presently being investigated.
The positive reduction potential exhibited by LtHb is also consistent with its resistance to oxidation compared to other HBOCs investigated in this study. Previously it was demonstrated that oxy LtHb (160 μM in heme) when mixed directly in human plasma remained in the reduced state up to 6 hours at 37°C as indicated by the constancy of the A576/A540 ratio and the absence of a charge-transfer band at 630 nm Citation[[7]]. Likewise, in a previous investigation from our laboratory, the protective reducing properties of compounds usually associated with hemoglobin redox stability were also shown to be more effective in reducing metLtHb than the met forms of other HBOCs. Non-mediated electron transfer (absence of coupling agent) was found to occur in vitro for metLtHb in the presence of glutathione, β-NADH (a cofactor required for reduction by cytochrome b5 reductase within the red blood cell), and ascorbic acid Citation[[7]]. Reduction by ascorbic acid was rapid and complete compared to the slower rates of reduction observed with either glutathione or β-NADH ( and ). The role of ascorbic acid in reducing LtHb in contrast to the other HBOCs is very unique in light of previous studies indicating that ascorbic acid converts to a pro-oxidative agent for other hemoglobins within a short period of time Citation[[24]]. After 270 minutes only partial reduction (45%) of HbXL99α and HbA was observed (). Continued reaction with ascorbic acid for longer periods of time (8–10 hours) resulted in conversion back to methemoglobin for each of these hemoglobins. The nature of this effective interaction of ascorbic acid in reducing Lumbricus hemoglobin is presently being investigated in our laboratory.
In summary, these studies suggest that: 1) the reduction potential of an HBOCs is important indicator of the redox stability for blood substitute considerations; 2) the naturally polymerized acellular Lumbricus hemoglobin exhibited a formal reduction potential that may explain resistance to oxidation and efficacy in being reduced by endogenous reducing components of human plasma; 3) an effective role for ascorbic acid is evident in its ability to reduce the acellular Lumbricus hemoglobin; and 4) further exploration of other naturally available acellular hemoglobins should be given serious consideration for the continued improvement of our understanding of the continued use of acellular hemoglobin-based oxygen carriers as a substitute for red blood cells Citation[[25]].
ACKNOWLEDGMENTS
This work was supported in part by the American Heart Association—Southeast Consortium (JPH) and Heritage Affiliate and NIH (REH).
REFERENCES
- Rachmilewitz E. A. Semin. Hematol. 1974; 11: 441–462
- Alayash A. I., Cashon R. E. Mol. Med. Today 1995; 1: 122–127
- Faivre B., Menu P., Labrude P., Vigneron C. Art. Cells, Blood Subs., and Immob. Biotech. 1998; 26: 17–26
- MacDonald V. W. Methods Enzymol 1994; 231: 480–490
- Lee R., Neya K., Svizzero T. A., Vlahahas G. T. J. Appl. Physiol. 1995; 78: 236–246
- Alayash A. I. Nature Biotechnol 1999; 17: 545–549
- Harrington J. P., Gonzalez Y., Hirsch R. E. Art. Cells, Blood Subs. and Immob. Biotech. 2000; 28: 477–492
- McGown E. T., Lyons M. F., Marini M. A., Zegna A. Biochim. Biophys. Acta 1990; 1036: 202–206
- Harrington J. P., Pandolfelli E. R., Herskovits T. T. Biochim. Biophys. Acta 1973; 328: 71–83
- Faulkner K. M., Bonaventura C., Crumbliss A. L. Inorg. Chim. Acta 1994; 226: 187–194
- Faulkner K. M., Bonaventura C., Crumbliss A. L. J. Biol. Chem. 1995; 270: 13604–13612
- Kenny C. F., Dorman S. C., Harrington J. P. Biophys. J. 1998; 76: 419
- Dorman S. C., Kenny C. F., Hirsch R. E., Harrington J. P. 8th Intern. Sym. Blood Subst. 2000; 69
- Scott E. E., Gibson Q. H., Olson J. S. J. Biol. Chem. 2001; 276: 5177–5188
- Manning J. M. Blood Substitutes: Physiological Basis of Efficacy, Winslow, Vandegriff, Intaglietta. Birkhauser, Boston 1995; 76–89
- Vandegriff K. D. Blood Substitutes: Physiological Basis of Efficacy, Winslow, Vandegriff, Intaglietta. Birkhauser, Boston 1995; 105–131
- Perutz M. F. Quart. Rev. Biophys. 1989; 22: 139–236
- Ackers G. K., Hazzard J. H. Trends Biochem. Sci. 1993; 18: 385–390
- Perutz M. F., Wilkinson A. J., Paoli M., Dodson G. G. Annu. Rev. Biophys. Biomol. Struct. 1998; 27: 1–34
- Fushitani K., Imai K., Riggs A. F. J. Biol. Chem. 1986; 261: 8414–8423
- Stellwagen E. Nature 1978; 275: 73–74
- Royer W. E., Strand K., Van Heel M., Hendrickson W. A. Proc. Natl. Acad. Sci. 2000; 97: 7107–7111
- Wierzbicki A., Salter E. A., Madura J. D., Sheff S. R., Harrington J. P. Biophys. J. 2001; 80: 291
- Sampath V., Caughey W. S. J. Am. Chem. Soc. 1985; 107: 4076–4077
- Hirsch R. E., Jelicks L. A., Wittenberg B. A., Kaul D. K., Shear H. L., Harrington J. P. Art. Cells, Blood Subs., and Immob. Biotech. 1997; 25: 429–444