Abstract
Petunia hybrida cell suspension cultures were exposed to ultrasonic standing wave fields at 2.43 MHz for 40 min with mean sound pressures (within homogenous sound fields) varying from 0 (control) to ca. 1.1 MPa. Mean (±s.d.; n=6–9) cell viability was reduced to 87±10% at 0.6 MPa and to 59±23% at 1.1 MPa, compared to an initial control value of 92±6% (P<0.05). Mean (n=3) cell alkaline phosphatase concentration increased linearly with sound pressure from a control value of 0.006±0.001 to 0.02±0.01 Sigma-Units μg −1 protein at 1.1 MPa (P<0.05). Similarly, mean cell catalase activity increased from a control value of 0.020±0.003 to 0.026±0.008 arbitrary units at 1.1 MPa. In contrast, mean cellular lactate dehydrogenase concentration was unchanged. These observations indicate that cellular repair processes associated with increased alkaline phosphatase activity might be triggered by physical cell damage caused by ultrasound. The observed increase in catalase activity suggests increasing production of free radicals and other sonochemicals, which warrants further study. The absence of changes in lactate dehydrogenase indicates that there was no major damage to respiratory pathways or to overall cellular integrity.
INTRODUCTION
Ultrasound offers several potential applications for the manipulation of prokaryotic and eukaryotic cells, whether they be of microbial, mammalian or plant origins. In addition to its use both in acoustic cell separation systems for replacing mechanical filters (Citation[1-3] and for the immobilization of cells, such as yeast (Citation[[4]]), ultrasound is currently employed in certain aspects of plant cell technology, including the stimulation of Agrobacterium-mediated genetic transformation (Citation[[5]]). A detailed understanding of the biological effects triggered by ultrasound is thus essential to minimise undesirable non-reversible physiological perturbations, as well as to optimise desirable outcomes utilising this process. A further justification for the present study was to examine cellular responses to ultrasound as a baseline for its wider application in, for example, downstream processing of biotechnologically valuable secondary products (Citation[[6]]). A recent study (Citation[[7]]), using cell suspension cultures of an albino line of Petunia hybrida cv. Comanche exposed to ultrasound at 2.43 MHz, demonstrated the relationship between cell viability and acoustic energy density, wave type, the duration of sonication and cell age. A comparison of different wave types suggested that the local pressure amplitude is the primary variable responsible for decreased cell viability. A similar investigation, involving transmission electron microscopy of yeast cells suspended in water and exposed to ultrasonic standing wave fields (Citation[[8]]), showed significant changes in cell morphology, including rearrangement of organelles and physical damage to vacuoles. It is possible that ultrasound may also cause similar effects on cultured plant cells, such as physical wounding, altered associations of organelles due to a breakdown of internal structure, production of free radicals and the disruption of metabolic pathways. One approach for assessing such physiological changes is to analyse the activities of specific enzymes following sonication of cultured cells. Therefore, in the present investigation, changes in the cellular activity of alkaline phosphatase, catalase and lactate dehydrogenase, which are readily assayable stress-induced enzymes, have been monitored to assess the effects of ultrasound on Petunia hybrida suspension cultured cells.
MATERIALS AND METHODS
Sonication Apparatus
The equipment and experimental treatments were based on those described earlier (Citation[[7]]), except that the sonicator was used only in standing wave field configuration, and a Mylar® polyester film (thickness 0.019 mm; Mylar® A19, DuPont Teijin Films UK, Middlesborough, UK) was used as a separation foil. Treatments were carried out in a sonication chamber of ca. 53 ml capacity, containing 48 ml of cell suspension, in order to provide an optimum homogenous sound field in the suspension. A rigorous, one-dimensional multi-layer model (Citation[[9]]) was used to determine the sound pressure (MPa) generated in the sonication chamber as a function of the voltage supplied (Citation[[7]]). The acoustic parameters of the piezo ceramics, together with those of the glass and water layers required for these calculations, were also taken from previous work (Citation[[7]]); the variables speed of sound (v long) and acoustic quality factor (Q) for the P. hybrida cell suspension were determined as reported previously (Citation[[7]]). The parameters for the Mylar® separation foil were either taken or calculated from the supplier's data sheet, except for Q, which was determined as described previously (Citation[[7]]). The characteristics relating to thickness (d), mass density (ρ), v long and Q for the separation foil and the cell suspension, are shown in .
Table 1. Acoustic Properties of the Separation Foil and Cells of P. hybrida Suspended in UM Culture Medium as Used in the Simulation Model
Sonication of Cell Suspensions and Assessment of Cell Viability
Cell suspensions of an accession of Petunia hybrida cv. Comanche derived from albino sections of variegated leaves of diploid plants, were maintained routinely by sub-culture every 7 d, in liquid UM medium (Citation[[10]]) at 24±2 °C with 25 μmol m −2 s −1 of continuous Daylight fluorescent illumination. To obtain a sufficiently high enzyme activity per sample volume, a cell density of 5×10 5 cells/cell colonies ml −1 medium was used in the current experiments, which was significantly greater than that used previously (Citation[[7]]). This cell density was obtained by using an unfiltered suspension, which contained cell colonies each up to 1 mm in diameter. The cell populations used for sonication were harvested 4 d post-sub-culture and were kept suspended by drawing the suspension into a 10 ml pipette and rapidly expelling the contents back into the chamber every 3 min with the sound field switched off. A control treatment was also performed using cells that were pipetted, but not sonicated, in order to assess that there was no decline in cell viability or density caused by such agitation.
Sonication treatments and viability assessments were repeated 3 times. Cell suspensions were exposed to an ultrasonic standing wave field at a frequency of 2.43 MHz for 40 min. Subsequently, a 3 ml aliquot of cells was harvested and used to determine cell viability by uptake and cleavage of fluorescein diacetate (FDA) (Citation[[11]]). Approximately 45 ml of the residual sonicated cell suspension was pelleted by centrifuging at 50× g for 7 min. The UM medium was removed from the cells using a Pasteur pipette and the medium frozen in 1.5 ml Eppendorf tubes for 2 d at −20 °C. One ml of extraction buffer (50 mM HEPES at pH 7.5, 10 mM EDTA, 5 mM MgCl 2·6H 2O) was added to ca. 1 ml packed volume of cells. The latter were frozen overnight at −20 °C and thawed, and the extraction buffer was removed but retained by storage at room temperature for a maximum of ca. 30 min. Subsequently, cells were lysed at room temperature using a glass homogeniser (10 ml capacity, BDH/Merck, Poole, UK); complete cell lysis was monitored by light microscopy. The lysate was re-suspended in the previously removed extraction buffer and again frozen overnight. Thawed samples were centrifuged at 10,000× g for 10 min at 4 °C, to remove cell wall material from the thawed lysate. Supernatants were aspirated and frozen in 1.5 ml Eppendorf tubes overnight at −20 °C.
Enzyme assays
Alkaline phosphatase activity in thawed supernatants was assessed by use of a commercial test-kit for phosphatases (alkaline, acid, prostatic acid; Procedure Nr 104, Sigma Diagnostics, Poole, UK). Catalase activity was monitored using the assay of Aebi (Citation[[12]]), except that the procedure was modified with a smaller sample concentration of 20 μl of extracted samples per 2 ml sample of reaction buffer for absorbance measurements at 240 nm. Lactate dehydrogenase concentration was assessed routinely using a commercial (LDH/LD) kit (Procedure Nr 500; Sigma Diagnostics). Total protein content was assessed spectrophotometrically at a wavelength of 595 nm following the protocol of Bradford (Citation[[13]]) using a commercial protein assay reagent (Bio-Rad Laboratories, Munich, Germany). Bovine serum albumin (BSA, Sigma), dissolved in reverse-osmosis water, was used as a protein standard solution. Standard solutions at concentrations ranging from 0–200 μg ml −1 of BSA were assayed in order to generate a standard curve which was used, in turn, to determine protein concentrations in the extracted samples of petunia cells. All spectrophotometric absorbance measurements were performed using a Lambda Bio UV/Vis Spectrometer (Perkin Elmer, Warrington, UK).
Statistical analyses
Means and standard deviations (s.d.) were used throughout. Statistical significance between mean cell viability and enzyme activity values was assessed, as appropriate, using conventional ANOVA and Student's t test (Citation[[14]]). A probability of P<0.05 was considered significant.
RESULTS
Typically, individual cells had a diameter of 60–80 μm, with cell colonies being up to 1 mm in diameter. The mean (±s.d.) viability of untreated (control) P. hybrida suspension cells was 92±6% (n=9). Exposure of cells to ultrasound for 40 min induced a progressive decrease in mean cell viability with increasing sound pressure (). Cell viability with 0.6 MPa was reduced to 87±10% (n=6), with further significant (P<0.02) decreases following exposure to 0.8 and 1.1 MPa (). Ultrasound induced a progressive increase in mean cell alkaline phosphatase activity with increasing sound pressure. For example, mean alkaline phosphate activity (40 min) at 1.1 MPa was 0.02±0.01 Sigma Units μg −1 protein (n=3), compared to 0.006±0.001 for untreated cells (P<0.05; ). Similarly, mean cell catalase activity tended to increase with increasing sound pressure, reaching a maximum mean value of 0.024±0.001 arbitrary units (a.u.) following exposure to ultrasound at 1.1 MPa (). In contrast, there was no significant change in cellular lactate dehydrogenase activity with increasing sound pressure ().
Figure 1. Mean viability of P. hybrida cells exposed to ultrasound at different sound pressures for 40 min. Error bars=s.d. (n=6–9).
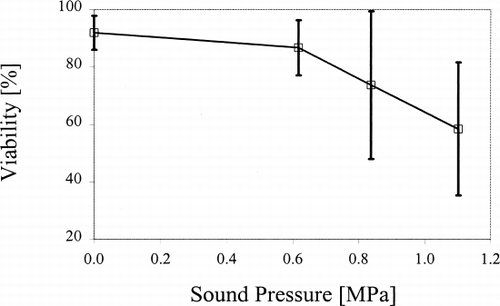
Figure 2. Mean alkaline phosphatase activity in P. hybrida cells exposed to ultrasound (40 min). Error bars=s.d. (n=3).
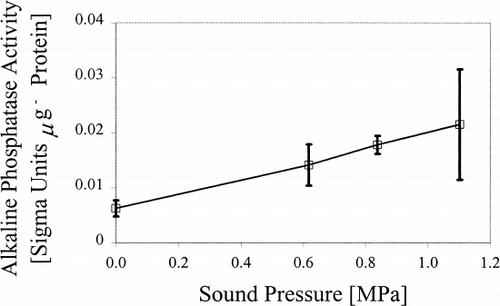
DISCUSSION
The present findings demonstrate that exposure of P. hybrida cell suspension cultures to ultrasound with a sound pressure greater than 0.8 MPa progressively caused a significant decrease in cell viability. The primary aim of the present investigation was to determine the effect of exposure of typical, dedifferentiated cultured plant cells to sound pressures of greater magnitude and duration than those normally used in acoustic cell separation systems for prokaryotic and eukaryotic cells. In this respect, in current separation systems, cells are routinely exposed to ultrasonic standing wave fields with comparatively lower sound pressures (ca. 0.5 MPa) and significantly shorter duration (ca. 2–3 min) than those used in the present study.
During sonication, cells are subjected to ultrasonic radiation pressure, of several orders of magnitude lower than the sound pressure (Citation[[15]]). The resulting primary acoustic radiation force components in the direction of the sound propagation align cell clusters at the pressure-nodal planes. Normally, the primary radiation force components perpendicular to the direction of sound propagation prevent such cell clusters from sedimenting. However, larger cell clusters would not be subjected to sufficiently high ultrasonic radiation forces to overcome sedimentation. Consequently, in the present study, regular agitation of the cell colonies in suspension was used to minimize such sedimentation effects and thus create a more-or-less uniform system for evaluating physiological (enzymatic) responses of plant cells to ultrasound. Agitation did not significantly affect cell viability and cell density (data not shown). An assay of total protein content in the UM medium was carried out to monitor possible cell leakage or damage. Major leakage, however, can be excluded since the total protein concentration was within the minimum resolution of the method, being typically 2 μg ml −1 (data not shown).
The present observation of an increase in cellular alkaline phosphatase activity in P. hybrida cells following exposure to ultrasound may reflect an increasing demand for energy to maintain cellular repair processes. This implies that cells exposed to ultrasound of the magnitude employed in this investigation may be subjected to subtle and, as yet undefined, physical damage. Indeed, Sanatrém et al. (Citation[[5]]) reported micro-wounding in immature cotyledons of soybean when ultrasound was used to stimulate Agrobacterium-mediated genetic transformation. However, sound pressure and wave type were unspecified and a frequency of 55 kHz was used in the latter study. Consequently, the form of any persistence of physical damage might be significantly different from perturbations elicited in P. hybrida cells exposed to a sound field with a frequency of 2.43 MHz, as in the present investigation. Furthermore, it is possible or indeed likely, that the responses to ultrasound will vary between different cell types within tissues even of the same plant and/or of different species.
Catalase promotes breakdown of hydrogen peroxide, normally present in cell microbodies (Citation[[16]], Citation[[17]]), thereby preventing exposure to high concentrations of potentially toxic oxygen radicals. While the changes in catalase activity observed in the present study were marginal, there is, nevertheless, a case for further study, not only to assess the effects of ultrasound on free radical generation, but also on the activity of other free radical detoxifying enzyme systems, such as peroxidases. This is justified further by previous studies showing that ultrasound generates cavitational effects, leading to hydrogen peroxide generation in mammalian cells in vitro (Citation[[18]]). Future work should, however, always take into account possible variations in metabolic-driven responses to ultrasound and sonochemicals between plant and animal cells.
In the present study, ultrasound had no marked effect on cellular lactate dehydrogenase activity. This enzyme activity generally increases with low oxygen levels during glucose metabolism (glycolysis) and occurs, primarily, when cellular respiration is inhibited, or when repair processes demanding high glucose consumption cannot be counterbalanced with adequate oxygen (Citation[[16]]). The present observations suggest that ultrasound does not have a profound effect on the glycolytic pathway, although further work is again needed to determine more precisely the short- and long-term effects that ultrasound may have on metabolic systems and other physiological processes in plant cells and their tissues.
ACKNOWLEDGMENTS
This study was supported by the European Commission TMR Network Contract No. ERBFMRXCT97-0156 (EuroUltraSonoSep).
REFERENCES
- Bierau H., Perani A., Al-Rubeai M., Emery A. N. A comparison of intensive cell culture bioreactors operating with Hybridomas modified for inhibited apoptotic response. J. Biotechnol. 1998; 62: 195–207
- Gröschl M., Burger W., Handl B., Doblhoff-Dier O., Gaida T., Schmatz C. Ultrasonic separation of suspended particles—part III: application in biotechnology. Acust. Acta Acust. 1998; 84: 815–822
- Zhang J., Collins A., Chen M., Knyazev I., Gentz R. High-density perfusion culture of insect cells with a BioSep ultrasonic filter. Biotechnol. Bioeng. 1998; 59: 351–359
- Radel S., Gherardini L., McLoughlin A. J., Doblhoff-Dier O., Benes E. Breakdown of immobilization/separation and morphology changes of yeast suspended in water-rich ethanol mixtures exposed to ultrasonic plane standing waves. Bioseparation 2000; 9: 369–377
- Sanatrém E. R., Trick H. N., Essig J. S., Finer J. J. Sonication-assisted Agrobacterium-mediated transformation of soybean immature cotyledons: optimisation of transient expression. Plant Cell Rep. 1998; 17: 752–759
- Kilby N. J., Hunter C. S. Towards optimisation of the use of 1.02-MHz ultrasound to harvest vacuole-located secondary product from in vitro grown plant cells. Appl. Microbiol. Biotechnol. 1991; 34: 478–480
- Böhm H., Anthony P., Davey M. R., Briarty L. G., Power J. B., Lowe K. C., Benes E., Gröschl M. Viability of plant cell suspensions exposed to homogeneous ultrasonic fields of different energy density and wave type. Ultrasonics 2000; 38: 629–632
- Radel S., McLoughlin A. J., Gherardini L., Doblhoff-Dier O., Benes E. Viability of yeast cells in well controlled propagating and standing ultrasonic plane waves. Ultrasonics 2000; 38: 633–637
- Nowotny H., Benes E. General one-dimensional treatment of the layered piezoelectric resonator with two electrodes. J. Acoust. Soc. Am. 1987; 82: 513–521
- Uchimiya H., Murashige T. Evolution of parameters in the isolation of viable protoplasts from cultured tobacco cells. Physiol. Plant. 1974; 54: 936–944
- Widholm J. The use of FDA and phenosafranine for determining viability of cultured plant cells. Stain Technol. 1972; 47: 189–194
- Aebi H. Catalase in vitro. Methods Enzymol. 1984; 105: 121–126
- Bradford M. M. A rapid and sensitive method for the quantitation of microgram quantities of protein utilizing the principle of protein-dye binding. Anal. Biochem. 1976; 72: 248–254
- Snedecor G. W., Cochran W. G. Statistical Methods 8th Ed. Iowa State Univ. Press, AmesUSA 1989
- Gröschl M. Ultrasonic separation of suspended particles—part I: fundamentals. Acust. Acta Acust. 1998; 84: 432–447
- Hall J. L., Flowers T. J., Roberts R. M. Plant Cell Structure and Metabolism. Longman, New York 1974
- Neumann D., Nover L., Parthier B., Rieger R., Scharf K.-D., Wollgiehn R., Zur Nieden U. Heat shock and other stress response systems of plants. Biol. Zentralbl. 1989; 108: 1–146
- Miller D. L., Thomas R. M., Frazier M. E. Ultrasonic cavitation indirectly induces single strand breaks in DNA of viable cells in vitro by the action of residual hydrogen peroxide. Ultrasound Med. Biol. 1991; 17: 729–735