INTRODUCTION
Since the report by Muirhead and Reid (1948), much work has been done using hemoperfusion over resins to remove exogenous toxins or endogenous metabolites (cf. Schechter et al., 1958; Pallotta and Koppanyi, 1960; Nealon and Ching, 1962; Nealon et al., 1966; Rosenbaum et al., 1970). Unfortunately, there are a number of problems preventing their widespread clinical use. When in direct contract with blood, ion exchange resins reduce blood platelet and leucocyte levels (Rosenbaum et al., 1970), and remove essential elements like calcium ions from perfusing blood (Rosenbaum et al., 1962). Another class of detoxicants which has been widely studied is activated charcoal. It has been known for many years as a useful detoxifying agent. For many years it has been used orally to remove ingested toxins in the gastrointestinal tract. However, activated charcoal administered orally does not act with sufficient speed or efficiency in patients who have already absorbed a large amount of toxin and are deeply intoxicated. In these cases, it has been found that a column of granular activated charcoal can efficiently remove the toxins from perfusing blood. Thus barbiturates (Yatzidas, 1964; Hagstram et al., 1966), Doriden® (DeMyttenaere et al., 1967), and other toxins (Dunea and Kloff, 1965) have been successfully removed from a blood-perfusing activated charcoal column. Unfortunately, we are again faced with a number of problems which prevent the widespread clinical use of hemoperfusion over activated charcoal. Activated charcoal in direct contact with blood adversely affects platelets (Dunea and Kolff, 1965; Hagstam et al., 1966; DeMyttenaere et al., 1967; Dutton et al., 1969). In addition, the charcoal granules, despite careful washing, release free particles into circulation, causing emboli in the lungs, liver, spleen, and kidney (Hagstam et al., 1966).
The principle of artificial cells has been used to circumvent these problems (Chang, 1966) (Fig. 2). Detoxicants enclosed in artificial cells will not come into direct contact with the formed elements of blood. Powder released from the enclosed detoxicant cannot leave the artificial cells to cause embolism. In addition, the enclosing membranes can be made selectively permeable to toxins so that essential elements of blood like calcium can be excluded. On the other hand, external permeant toxins can diffuse freely across the artificial cell membranes to be removed by the enclosed detoxicants. The selective removal of toxin can be accomplished by variations in membrane porosity, lipids, charge, and other properties.
This study has been carried out through a number of stages. Initial work was done (Chang, 1966; Chang et al., 1967b) using a slight modification of the standard procedure (Chang, 1964; Chang et al., 1966) to enclose ionexchange resins and activated charcoal in artificial cells. When this basic research demonstrated the feasibility of this system, modified procedures were used to prepare artificial cells suitable for use in scaled-up systems (Chang et al., 1968, 1970; Chang, 1969e), which is now being tested clinically (Chang, et al., 1970, 1971).
STANDARD ARTIFICIAL CELLS
With slight modification of the standard procedure, nylon artificial cells containing Dowex®-50W-X12 have been produced (). Ion exchange resins are suspended in the aqueous phase containing hemoglobin and alkaline diamines. After adjusting the pH to 10.5, the remaining steps for the preparation of nylon membrane artificial cells were followed. Shunts have been loaded with these artificial cells of up to 120 ml. Over short periods of perfusion, such a shunt is effective in removing most of the ammonia from the perfusing blood of animals whose ammonia levels have been raised by the continous infusion of ammonium sulfate. Some technical difficulties still have to be overcome before this system can be used for prolonged perfusion. In particular, the artificial cells, whose membranes are flexible, tend to become so closely packed when used in such large volume that blood flow diminishes in spite of repeated reversal of flow. Activated charcoal can also be microencapsulated (Chang, 1966). This is done by suspending activated charcoal powder in the aqueous phase, followed by the standard procedure for the preparation of artificial cells with nylon membranes, collodion membranes, or heparin-complexed membranes (Chang, 1964; Chang et al., 1966, 1967b). Other laboratories have also been successful in using our procedures for the enclosure of ion exchange resins or activated charcoal in nylon membrane artificial cells (Levine and LeCourse, 1967; Sparks et al., 1969).
Figure 53. Artificial cells containing enzymes and Dowex-50W-12. (From Chang, 1966. Courtesy of the American Society for Artificial Internal Organs.)
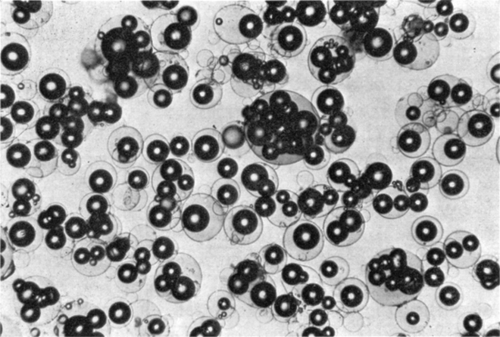
Because of the flexibility of the membranes, this system, though useful for small-scale basic research, cannot be readily used in scaled-up systems suitable for clinical use. For this, further steps of development are required.
BHCAC ARTIFICIAL CELLS
Having demonstrated that polymer coating can prevent the problems of packing and breaking of membranes under a high flow situation, heparin-complexed collodion was used as the enclosing membrane (Chang et al., 1968), since earlier results (Chang et al., 1967b, 1968), indicated that it is the least harmful to the formed elements of blood. Coating of particles can be done in a large variety of ways. For instance, in a typical example (Chang et al., 1968), 100 ml of heparin-benzalkonium collodion solution prepared as described (Chang et al.,1967b) is added to 50 gm of activated charcoal. (Instead of the smaller granules, the 6–14 mesh granules, Fisher Co., are less liable to cause packing.) The suspension is manually stirred until the solvent of the polymer solution has evaporated sufficiently so that the microencapsulated particles are no longer adherent to one another. The artificial cells are then spray-dried at room temperature and suspended in saline over night. Experiments have been carried out to test the effect of microencapsulated activated charcoal on the formed elements of blood. Forty grams of either the uncoated activated charcoal or the microencapsulated activated charcoal formed by coating with heparin-complexed collodion was placed in the extracorporeal shunt chamber described. Blood from dogs was perfused through the shunt at 100 ml/min. The effect on platelet level is shown in . Activated charcoal enclosed in artificial cells did not have any adverse effect on the blood platelet level. On the other hand, free activated charcoal which was not enclosed in artificial cells caused a 50% reduction in the arterial platelet level of heparinized dogs and an even greater decrease in the shunt effluent level. The use of the large mesh activated charcoal granules (6–14 mesh) allowed a large amount of heparin-complex coated activated charcoal to be used (300 gm). In addition, a blood flow rate of 200 ml/min or more could be used without resulting in packing at the exit screen.
Figure 54. Effects of activated charcoal in BHC-artificial cells and of free activated charcoal on the platelet level of arterial and shunt-effluent blood. (From Chang et al., 1968. Courtesy of the American Society for Artificial Internal Organs.)
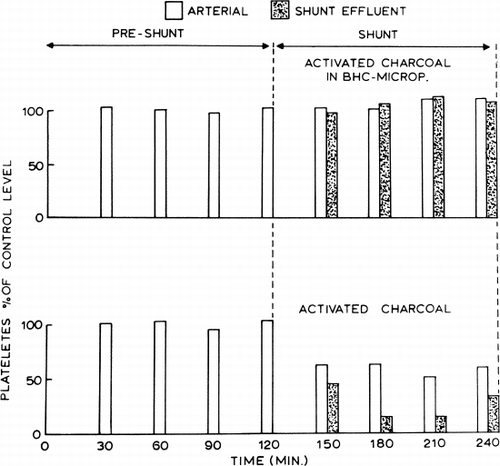
This system does not adversely affect the formed elements of blood and does not result in the release of embolising powder. It is also capable of removing some Doriden, salicylate, Nembutal®, and uremic metabolites. However, the membrane thickness prevents the efficient removal of these toxins. With thinner membranes this preparation is more efficient, but in this form, the membrane contained too little heparin to have a platelet protection and nonthrombogenic effect. This problem is being solved by variation in membrane materials and changes in heparinization procedures. In the meantime, a different approach has led to the preparation of a system for clinical use.
ACAC ARTIFICIAL CELLS
In this approach, instead of using heparin, albumin is complexed into the artificial membrane by the following procedure (Chang, 1969e): 400 gm of activated charcoal granules (6–14 mesh, Fisher Co.) wrapped in sterile cloth is autoclaved at 121°C, 15 pounds per square inch, (psi) for one hour. After this, it is left in the 50°C ventilator for at least 96 hours. A polymer solution is made up by dissolving 20 ml of collodion (U.S.P.) in an organic solvent consisting of 20 ml of alcohol and 400 ml of ether. The 440 ml of polymer solution is poured into a beaker containing the 400 gm of activated charcoal. The suspension is stirred manually with a metal stirring rod until a free-flowing polymer solution is no longer present in the container. The slightly wet polymer-coated activated charcoal is spread out in a 2×1-foot tray. This is then placed in a ventilated oven for five hours at 50°C. After this the polymer-coated activated charcoal granules are placed in 10 liters of pyrogen-free distilled water. The suspension is sieved (No. 20 mesh) continuously with more pyrogen-free water until all fine particles are removed. The 400 gm of polymer-coated activated charcoal is then placed in a silicone-coated high density polypropylene shunt prepared as described (Chang, 1969e). The shunt is then drained, stoppered, wrapped, sealed and placed in the autoclave for 30 minutes at 121°C, 15 psi. It is important not to pass through the stage of “liquid cool”; instead, the autoclave is “vented” at the end of 30 minutes. At the end of this, the shunt is allowed to cool in its sealed, sterilized wrapping. Using sterile technique, the shunt perfused with 4 liters of saline (Baxter, for human use) at 200 ml/min by gravity. A 1 gm/100 ml albumin solution in sterilized saline is prepared from human albumin (Cutter Co.). This albumin solution is used to displace all the saline from the shunt containing the polymer-coated activated charcoal. The shunt is then sealed by sterilized technique and kept at 4°C for 15 hours for the albumin to coat the polymer membrane. Just before use, the albumin solution is displaced with 4 liters of saline (Baxter, for human infusion) without allowing the albumin-polymer-coated activated charcoal to come into contact with air at any time. After injecting 2000 units of heparin into the shunt, the system is now ready for use by attachment to the arteriovenous shunt.
Hematological Findings: Formed Elements of Blood
Detailed studies of different types of microencapsulated activated charcoal on the formed elements of blood have been done (Chang, 1969e; Chang and Malave, 1970). In these experiments 3 mg/kg of heparin was used, 1 mg/kg into each shunt containing 300 gm of ACAC microencapsulated charcoal and 2 mg/kg intravenously. After taking control samples hemoperfusion (120 ml/min) was started; no blood pump was used. The results obtained are briefly summarized in . In hemoperfusion across free activated charcoal granules there was a marked drop in systematic platelets and leucocyte levels. ACAC microencapsulation prevented this adverse effect on platelets or leucocytes. Plasma hemoglobin measurements showed that neither free activated charcoal nor ACAC microencapsulated activated charcoal caused any hemolysis of perfusing blood.
Table 4. Effects of 2-Hour Hemoperfusion on Systemic Platelets and Leucocytes
Histological Findings
After hemoperfusion through free activated charcoal, Hagstam (Hagstam et al., 1966) found charcoal particles in effluent blood and masive charcoal emboli in the lungs, livers, kidneys, and spleens of the test animals. In our experiments using artificial cells containing activated charcoal (ACAC artificial cells), no charcoal particles were found in smears of effluent blood from shunts (Chang, 1969e); however, charcoal power was consistently observed from those shunts containing free activated charcoal (Chang, 1969e). Studies have been done on histological sections of lungs after two hours of hemoperfusion through 300 gm of ACAC microencapsulated activated charcoal (Chang and Malave, 1970). Sections obtained from four dogs were examined carefully and no charcoal emboli could be found in the first three dogs treated with preparations which had not been autoclaved. In the fourth dog treated with autoclaved microencapsulated charcoal, four very small charcoal particles in the precapillary vessels were found. With more careful washing of the autoclaved preparations, no charcoal particles were found in the histological sections of the fifth, sixth, and seventh dogs. Histological sections of liver, spleen, and kidney obtained from four dogs showed no evidence of charcoal embolism. Thus, unlike the massive embolism resulting from hemoperfusion through free activated charcoal, hemoperfusion through carefully washed ACAC artificial cells did not result in any such embolism.
Long-Term Follow-Up Studies
In control experiments, four Nembutal-anesthetized dogs underwent percutaneous femoral artery catheterization (14-gauge catheter). In hemoperfusion experiments, seven Nembutal-anesthetized dogs underwent similar percutaneous arterial catheterization. Each of these animals was then treated with two hours of hemoperfusion through 300 gm of sterile ACAC microencapsulated activated charcoal and then allowed to recover. All animals have been followed for more than one month. There were no adverse effects.
Pentobarbital as a Model Exogenous Toxin
The efficiency of the ACAC artificial cell system for the removal of exogenous toxins has been tested using a barbiturate, pentobarbital, as a model system (Chang 1969e, 1971b; Chang et al., 1970). Barbiturates belong to the group of drugs commonly encountered in accidental or suicidal overdosage. In these studies dogs are given intravenous injections of pentobarbital (50–60 mg/kg) and then supported by artificial respiration. Control studies show that the rate of decrease of the arterial pentobarbital level is slow. On the other hand, hemoperfusion over ACAC artificial cells rapidly lowers the arterial pentobarbital levels. A typical example is shown in . Forty-five minutes after the infusion of pentobarbital, control blood samples are taken at 15-minute intervals for one hour. An extracorporeal shunt containing 300 gm of ACAC artificial cells is then connected to the femoral artery and femoral vein of the heparinized animal. A Travenol blood pump is used to maintain an extracorporeal blood flow of 200 ml/min. As can be seen in , the arterial blood pentobarbital level fell exponentialy and rapidly during the two hours of hemoperfusion. The effectiveness of the hemoperfusion over ACAC artificial cells is shown both by the rate of decrease of arterial pentobarbital levels and also, in some cases, by the animal's waking up from the effects of the pentobarbital.
Figure 55. Arterial pentobarbital level in dogs. Control: dog without treatment. Treated: dog with systematic circulation connected to an extracorporeal shunt containing 300 gm of ACAC artificial cells (blood flow 200 ml/mg) for two hours.
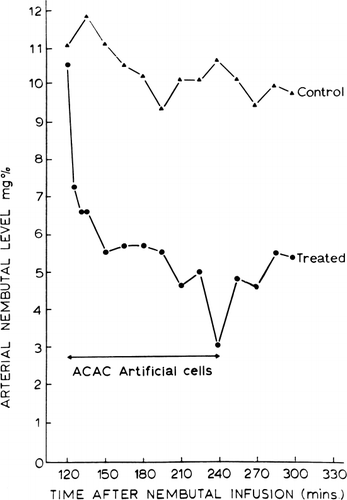
Pentobarbital in the body is distributed into four compartments: blood pool, viscera, lean tissues, and adipose tissues (Dedrick and Bischoff, 1966). The visceral compartment, which includes the central nervous system, receives a high blood flow, whereas the adipose tissue receives a low blood flow. Thus, using this compartmental system, we can say that hemoperfusion over ACAC artificial cells removes pentobarbital from the blood compartment along with any pentobarbital entering the blood compartment along a concentration gradient from the other compartments. From the analysis of Dedrick and Bischoff (1966), it can be seen that the rate of fall of free concentrations of pentobarbital in the visceral compartment (including the central nervous system) falls at the same rate as the pentobarbital concentration in the blood compartment; on the other hand, the concentration in the adipose tissue lags behind the other compartments. Thus it would be expected that hemoperfusion over ACAC artificial cell could efficiently remove pentobarbital from the blood compartment and the central nervous system sufficiently to revive the comatose individual. On the other hand, on termination of hemoperfusion, pentobarbital will continue to diffuse along a concentration gradient from the adipose tissue into the blood comparment, thus resulting in a gradual increase of the blood pentobarbital level, as can be seen in . The degree of increase of blood pentobarbital level will depend on the amount of adipose tissue of any one particular individual.
Salicylates as a Model Exogenous Toxin
Aspirin, a salicylates, is the most important cause of accidental poisoning among children under the age of five years. The efficiency of ACAC artificial cells in the removal of salicylate has been studied in this laboratory using dogs.
A typical example is shown in . In each set of experiments 200 mg/kg of sodium salicylate was given intravenously by infusion to two Nembutal-anesthetized dogs which had previously been nephrectomized to prevent the renal excretion of salicylates. Arterial salicylate levels were followed at 15-minute intervals for one hour. After this, one of the dogs was used as a control. The circulation of the other dog was connected to an extracorporeal shunt containing 300 gm of ACAC artificial cells. Hemoperfusion over the ACAC artificial cells in the extracorporeal shunt was facilitated by the use of a Travenol rotary pump adjusted to a flow rate of 200 ml/min. The arterial salicylate levels in the control animal remained elevated. During this period, the animal exhibited the usual signs of salicylate intoxication. The period of marked hyperpnea was followed by respiratory failure, cardiovascular collapse, and death of the untreated animal. In the case of the animal treated with the ACAC artificial cells, the arterial salicylate levels fell rapidly, and the clinical signs of salicylate intoxication were no longer present soon after the initiation of hemoperfusion. Clearance of salicylate was maintained at 110 ml/min to 120 ml/min for the first 90 minutes.
Figure 56. Arterial salicylate levels in dogs. Control: dog without treatment. Treated: dog with systematic circulation connected to an extracorporeal shunt containing 300 gm of ACAC artificial cells (blood flow 200 ml/min) for two hours.
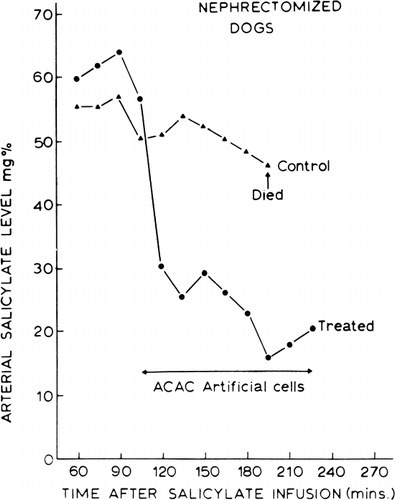
The efficiency of the ACAC artificial cells for the treatment of salicylate intoxication is clearly demonstrated in these experiments. The size of the animals (25–30 kg) and the amount of salicylates administered (4.5–5.5 gm to each animal) in these studies are comparable to what one may encounter in pediatric salicylate intoxication.
Doriden as a Model Exogenous Toxin
Doriden (glutethimide) is another medication commonly encountered in acute intoxication. Preliminary studies in this laboratory using dogs show that Doriden is efficiently removed by the ACAC artificial cells. Dogs were given 150 mg/kg of Doriden. The animal's blood was recirculated at 300 ml/min (rotary pump) through the extracorporeal shunt chamber containing 300 gm of ACAC artificial cells. This way the clearance of Doriden was found to be 150 ml/min for the first hour and 129 ml/min for the second hour. Thus, about 1 gm of Doriden was removed by the 300 gm of ACAC artificial cells within two hours.
GENERAL CONCLUSIONS
This chapter serves to illustrate the feasibility of the general idea of artificial cells. By placing detoxicants in an intracellular environment, the detoxicants can continue to remove permeant toxins without adversely affecting extracellular essential elements like blood cells, protein, and calcium. The examples given in this chapter serve only as model systems to test the efficiency of the artificial cell system. The demonstrated efficiency of these model systems should encourage one to study artificial cells containing other types of detoxicants for the removal of the large numbers of exogenous toxins. The simplicity and the compactness of this system will greatly facilitate the treatment of acute intoxication. Other membrane systems should also be explored. Thus the Utah group (Kolff, 1970; Andrade et al., 1971a and 1971b) is now exploring the microencapsulation of activated charcoal by coating with Hydron, a polymer known for its biological inertness. We are also exploring other membrane systems including cellulose acetate in this laboratory.