Abstract
Hemoglobin raffimer (HEMOLINK™, Hemosol Inc, Mississauga, Canada) is an o‐raffinose cross‐linked, purified human hemoglobin‐based oxygen therapeutic that is currently being evaluated in late stage clinical trials. It is composed of several molecular weight (MW) species, comprising principally of stabilized tetramers (34–42%) and oligomers (54–62%). The objective of this study was to determine the in vivo circulating half‐life (T1/2) of hemoglobin raffimer (Hb raffimer) and of its individual MW components in dogs subjected to a topload infusion of 25% of the estimated blood volume (18 mL/kg). Sampling was done over a 64‐hour period that was expected to be equivalent to approximately two‐and‐half to three half‐lives. Methemoglobin (MetHb) levels were also measured at intervals over the same period. The mean circulating half‐life of Hb raffimer was 25.4 ± 3.9 hours. The T1/2 for the individual MW components (determined by size exclusion chromatography) of Hb raffimer was 11 ± 2 hours for the cross‐linked tetramer and 35 ± 7 hours for the fraction of oligomers. The apparent volume of distribution for Hb raffimer was estimated at 78 mL/kg. There was no difference in the apparent volumes of distribution of the tetrameric and oligomeric components of Hb raffimer. Throughout the course of the experiment (in which MetHb could be measured), plasma MetHb concentration, expressed as a percentage of the total plasma hemoglobin concentration, remained at 10% or less, and the mass concentration of MetHb in plasma remained at about 1 g/L. Thus, in the dog subjected to an estimated 25% topload infusion, the T1/2 of the infused Hb raffimer is approximately one day with the intravascular retention of the individual Hb raffimer components being dependent on the MW. Furthermore, oxidation of Hb raffimer to MetHb is limited under these conditions.
Introduction
Cell‐free preparations of hemoglobin of human or bovine origin are being developed to replace allogeneic red cell transfusions in various clinical indications (Cohn, [Citation2002]). To make these preparations clinically useable, extensive purification and chemical cross‐linking procedures have been developed, to remove red cell stromal fragments which have toxic properties, and to enlarge the molecules to improve their intravascular retention. When large amounts of free hemoglobin are present in the plasma, it spontaneously dissociates from its tetrameric state to alpha‐beta dimers (MW 32 KDa) which are rapidly excreted. Covalent cross‐linking stabilizes the molecule in the tetrameric or oligomeric form, thereby enlarging the molecule and rendering it less likely to be filtered, thus prolonging its intravascular retention and physiological efficacy as an oxygen transporter, or an “oxygen therapeutic”. Other modifications are also required to offset the increase in oxygen affinity.
The high oxygen environment to which the hemoglobin in the red cell is exposed favours the oxidation of the heme iron to the ferric form, resulting in the formation of methemoglobin (MetHb) (Brooks, [Citation1935]). In this form, the pigment is not able to bind oxygen reversibly and is, therefore, physiologically inactive. An important mechanism preventing this loss of functionality is the MetHb reductase activity within the red cell (Scott et al., [Citation1965]), which, with the generation of NADPH through the hexose monophosphate shunt, reduces the heme iron to the ferrous state, restoring its functionality. When the hemoglobin is liberated from the protective environment of the red cell, it is no longer protected from oxidation by this mechanism. Hence when administered in vivo, the maintenance of the physiological efficacy and functionality of the oxygen therapeutics requires that MetHb formation be minimal.
Hemoglobin raffimer (HEMOLINK™, Hemosol, Inc, Mississauga, Ontario, Canada) is a cell‐free human hemoglobin solution under development as an oxygen therapeutic. It is manufactured from outdated human red cell units collected and approved for transfusion, by a method that involves lysis, pasteurization, chromatographic purification, filtration and cross‐linking by o‐raffinose, which is derived from a naturally occurring trisaccharide. The cross‐linking occurs both within the hemoglobin molecule to stabilize it in the tetrameric form, as well as inter‐molecularly, generating oligomers. Hemoglobin raffimer (Hb raffimer) is prepared from purified hemoglobin which contains > 99% hemoglobin A0. After cross‐linking, Hb raffimer is comprised of approximately 34–42% cross‐linked tetramer (MW 64 kDa) and 54–62 % oligomer (MW 64–500 kDa). The intravascular persistence, volume of distribution and rate of heme oxidation in vivo were estimated in an experiment on beagle dogs given Hb raffimer by intravenous infusion.
Methods
The experiments were performed by ITR Laboratories, Montreal Quebec a Clinical Research Organization in compliance with Good Laboratory Practices. Beagle dogs were used, after approval by an Animal Care Committee, and the animals were treated in all respects in compliance with the Guidelines of the Canadian Council on Animal Care. The dogs (8.2–12.7 kg body weight) were housed individually in a controlled environment and fed a standard certified commercial dog chow and given water ad libitum. A clinical veterinarian carried out a detailed physical examination and blood analysis to ensure good health before dogs were included in the study.
Characteristics of the Hb Raffimer Solution
Hb raffimer is supplied at a concentration of 10 g/dL and is dissolved in lactated Ringer's solution. The Hb raffimer supplied for this study was packaged in the de‐oxy form and can be stored refrigerated, and is in use for the current clinical trials. Its physicochemical characteristics include: pH 7.5, kinematic viscosity 1.11 cSt, colloid osmotic pressure 30 mmHg, MetHb content 8.6% and endotoxin < 0.06 EU/mL; these are within acceptable limits for release.
Experimental Procedures
After three days of acclimation to the experimental environment and with the dogs standing in a Pavlov sling, the experiment proper commenced. An indwelling catheter was inserted in the cephalic vein and Hb raffimer, warmed to room temperature (approx. 25°C), was infused by a pump at a rate of 10 mL/min, to a total dose of 18 mL/kg (approx. 25% of the estimated blood volume). Blood samples were collected from the jugular vein immediately prior to the start of the infusion, and at 0.25, 0.5, 0.75, 1, 2, 4, 8, 12, 16, 20, 24, 28, 32, 36, 44, 52, 60 and 64 hours after the end of the infusion. These times were chosen in the anticipation that the intravascular half‐life of Hb raffimer would be about 24 hours, and would thus provide at least three time points in each of three consecutive half‐lives, as well as sufficient additional time points during the early “post‐mixing” period.
Blood Sampling and Analytical Procedures
At each blood collection time point, approximately 1 mL of blood was drawn into each of three tubes (one containing lithium and heparin, and two containing EDTA). The tube containing the heparin was used to determine MetHb concentration in plasma and total hemoglobin concentration in whole blood. The first EDTA tube was used to determine plasma hemoglobin concentration and the second EDTA tube was frozen and sent to Hemosol Inc. for analysis of MW distribution by size exclusion chromatography (S.E.C.). An additional 10 mL sample of “blank” canine plasma and whole blood from a spare dog was also obtained and used by Hemosol, Inc as blank canine hemoglobin in chromatographic analysis.
Following the infusion urine was collected over 12‐hour periods from the cage pans and samples were frozen for the subsequent determination of hemoglobin concentration.
Total hemoglobin and MetHb concentrations in samples of whole blood and separated plasma were determined with an OSM3 Hemoximeter. Samples were retained frozen for subsequent manual analysis (Evelyn‐Malloy method) if total hemoglobin concentration in the plasma was < 10 g/L, at which concentration the automated instrument loses accuracy. Whole blood and plasma MetHb concentrations were not reported when plasma Hb concentration was < 10 g/L, as the measurement is not accurate below this level. Hemoglobin concentration in urine was determined by a Monarch 2000 automated biochemistry analyzer.
Size Exclusion Chromatography
Plasma samples were analyzed by size exclusion chromatography under conditions that dissociate all non‐covalently cross‐linked hemoglobin into αβ dimers (Guidiotti, [Citation1967]). Twenty μL aliquots of the thawed plasma samples were diluted with Milli‐Q water (to a 300 μL final volume), sealed and briefly vortexed. The plasma samples were analyzed using a Beckman System Gold HPLC system. Samples (100 μL) were injected onto a Superdex® 200 Column (Pharmacia) and eluted with 0.5 M MgCl2 in 25 mM Tris‐HCl, pH 7.2 at a flow rate of 0.4 mL/min. Needle washes occurred between all samples to avoid cross‐contamination. Eluant was monitored at 280 and 414 nm to assist in the identification of hemoglobin (absorbance at 414 > 280) versus non‐heme (absorbance at 280 > 414) proteins. Additionally, absorbance surface data from 220–600 nm were collected on the first replicate of each specific sample throughout the chromatographic run.
Pharmacokinetic analysis was performed using WinNonlin software (Pharsight Corp. Mountainview, California) for non‐compartmental analysis for constant intravenous infusion, yielding circulating half‐life for hemoglobin by the least squares method. Additional pharmacokinetic analysis for the individual MW components of Hb raffimer, as determined by size exclusion chromatography (see above), was performed using Grafit software (Erithacus Software, U.K.). The volume of distribution for total Hb raffimer as well as the tetrameric (64 kDa) and oligomeric (> 64 kDa) fraction were calculated using the concentration of Hb raffimer injected and the plasma concentration of total hemoglobin, the 64 kDa fraction and the > 64 kDa fractions, respectively.
Reverse Phase Chromatography
Small but variable amounts of dimeric (32 kDa) hemoglobin were detected at each time point after infusion. Reverse phase chromatographic analysis was performed to identify whether the dimeric hemoglobin was of human or canine origin since canine hemoglobin, originating from small amounts of lysed canine red cells, could have potentially contaminated the samples, whereas dimeric hemoglobin derived from Hb raffimer would be identifiable as human. The post‐infusion sample with the highest measured concentrations of 32kDa “dissociable” hemoglobin was fractionated by size exclusion chromatography on a Superdex® 75 column (Pharmacia) to isolate the 32kDa Hb fraction. The post‐infusion fraction was then exhaustively dialyzed (3 ×) against Milli‐Q water in “Slide‐a‐Lyzer®” dialysis cassettes (0.5–3 mL capacity) having a molecular weight cut‐off (MWCO) of 10,000 and subsequently concentrated using Centricon™ 10 concentrators to reduce the total volume to 200 μL. To serve as a true control for the reverse phase chromatography, a canine hemoglobin solution, free of cellular fragments, was prepared from a blood sample obtained from a control beagle dog (i.e. a dog not administered Hb raffimer). In addition to this control canine hemoglobin sample, additional control samples were also run consisting of the 32 kDa fraction of Hb raffimer as well as a sample of purified human HbA0. All samples were diluted to a total final concentration of 0.08% with Milli‐Q water and run on reverse phase using neat 20 μL injections. Reverse phase chromatography was performed, using a Beckman System Gold HPLC system. Chromatography conditions followed several linear gradients of varying acetonitrile:water ratios (from 39.2% to 52% acetonitrile) at a constant 0.1% trifluoroacetic (TFA) acid concentration. These conditions were chosen as they provide baseline resolution between the α and β globin chains of normal human hemoglobin. A 0.46 × 025 cm Vydac C4 column (The Separations Group, Heperia, California) was used, on the HPLC. Flow rate was 0.9 mL/min and eluant was monitored at 220 nm and 414 nm.
Results
Three dogs were given Hb raffimer infusion. All tolerated the procedures well, were in good clinical condition throughout the duration of the experiment and were returned to the animal colony on completion of the study.
The time course of the disappearance of hemoglobin from plasma is shown in . The intravascular half‐life of Hb raffimer as expressed by the total hemoglobin concentration in the plasma phase was 25.4 ± 3.9 (SD) hours. The WinNonlin software also provided the parameters Cmax and Tmax, but these are of little value in this case, since the initial samples were drawn 15 minutes after the infusion was completed, prior to which time the maximum concentration would have already been reached. Hence, these parameters are not reported.
Figure 1. Plasma clearance and half‐life (T1/2) of total Hb raffimer (THb) administered to dogs subjected to a single topload infusion of 18 mL/kg. Total hemoglobin values are shown by the filled circles (•). Mean plasma methemoglobin (MetHb) levels are given by the (*) symbol. All values are presented as mean ± SD.
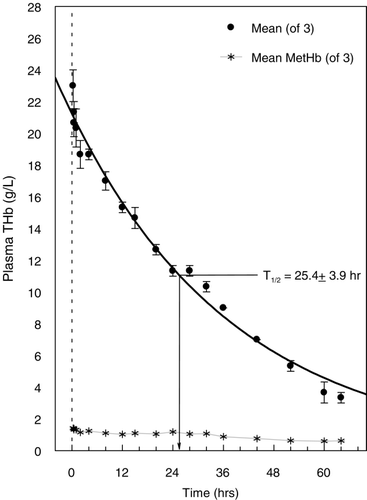
Size exclusion chromatography identified and quantified the various molecular weight species. This yielded intravascular half‐lives for the various molecular weight fractions which is summarized in . The relative percentage of the various hemoglobin moieties in the plasma over time is summarized in . Hemoglobin dimers were present in small but variable amounts. However, as shown in , it was determined by reverse phase chromatography that the dimers were consistent with those of canine, not human hemoglobin.
Figure 2. Plasma clearance and half‐lives (T1/2) of the different hemoglobin fractions that make up Hb raffimer as determined by size exclusion chromatography under dissociating conditions. The disappearance of the 64 kDa fraction (tetramer) is shown in the top panel, the 128 kDa fraction in middle panel, and the fraction composed of all molecular weight species > 128 kDa in the bottom panel. All values are shown as mean ± SD for all animals.
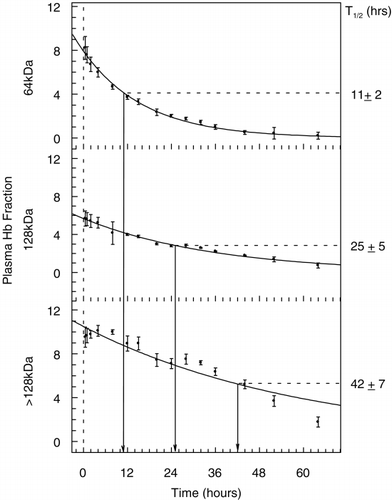
Figure 3. The relative percentage of the different hemoglobin fractions that make up Hb raffimer in the plasma over time. Grey bars indicate the 32 kDa fraction, hatched bars indicate the 64 kDa fraction and open bars indicate > 64 kDa fraction.
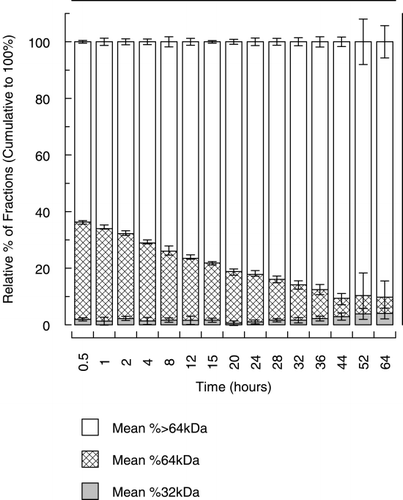
Figure 4. Reverse phase chromatogram showing absorbance at 220 nM. Chromatograms presented are from a control beagle dog, human hemoglobin A0, the 32 kDa fraction of Hb raffimer and a 32 kDa fraction collected from a dog 52 hours after treatment with Hb raffimer. For this sample the scale is expanded 2 ×.
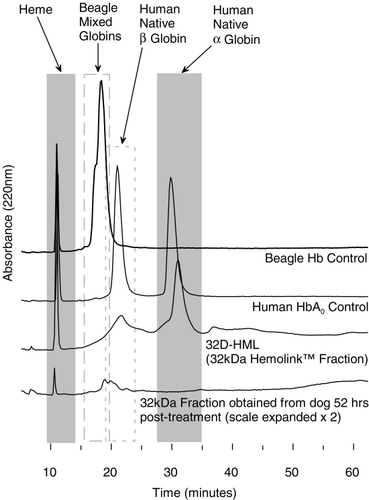
From the maximal concentration of hemoglobin measured in plasma at 15‐min post‐infusion (23 ± 1.7 g/L), the apparent volume of distribution was estimated at 78 mL/kg. This is approximately 24% larger than the “expected” plasma volume, taking into account the enlargement of the plasma volume by the 18 mL/kg topload infusion. The apparent volumes of distribution of the 64‐kDa and > 64‐kDa fractions of Hb raffimer were estimated at 76.6 and 74.6 mL/kg. Hence there appears to be no difference in the apparent volumes of distribution of the tetrameric and oligomeric components of Hb raffimer.
The timecourse of MetHb found in plasma is shown in . During the course of the experiment, representing approximately 2.5 half‐lives, plasma hemoglobin declined from 23 ± 1.7 g/L to approximately 3 g/L, while total whole blood hemoglobin concentration increased from 120 ± 3.1 g/L at 15 min to 132 ± 5.7 g/L at 64 hours. Relative plasma MetHb concentration during the experiment increased from 6.2 ± 0.3 to 10.4 ± 0.2 %, near the end of measurement at 32 hours. The apparent rate of appearance was approximately 0.13 %/hr, when total hemoglobin concentration in plasma declined nearly 2.5 fold over the same time interval. As a result, the calculated MetHb mass‐concentration in plasma remained essentially stable, declining from 1.4 to 1.04 g/L. Expressed as a percentage of the total hemoglobin mass‐concentration in whole blood (), MetHb represented 1.1% of the total at the beginning of the experiment and 0.8% at the end. Throughout the course of the entire experiment whole blood MetHb concentration was about 1 % or less.
Figure 5. Plasma MetHb levels (•), expressed as a percentage of the total plasma hemoglobin concentration (top panel) and also presented as the plasma mass concentration in g/L (bottom panel). Levels are not reported beyond 32 hrs as hemoglobin concentration has fallen below 10 g/L and measurement of MetHb is not accurate at such low levels of total plasma hemoglobin. For comparison, total plasma hemoglobin concentration (○), expressed in g/L is also shown on the right Y‐axis. All values presented are given as mean ± SD.
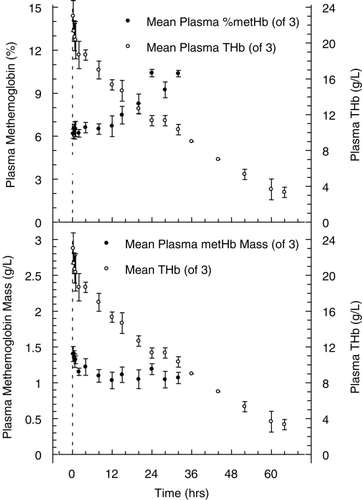
Figure 6. Whole blood methemoglobin levels (•), expressed as a percentage of the total whole blood hemoglobin concentration (top panel) and also presented as the whole blood mass concentration in g/L (bottom panel). For comparison, total whole blood hemoglobin concentration (○), expressed in g/L is also shown on the right Y‐axis. All values presented are given as mean ± SD.
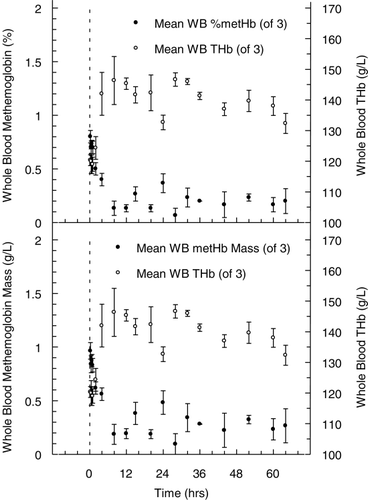
Hemoglobin determinations in urine excreted over 12‐hour periods showed that the concentration was very low (). Since accurate determinations of the volume excreted during this period could not be made, the amount of hemoglobin, either as absolute mass, or the fraction of the injected dose, could not be estimated.
Table 1. Mean urine hemoglobin concentrations (g/L)
Discussion
The results of this study demonstrate that Hb raffimer disappears from plasma with a single‐exponential decay, both for Hb raffimer overall as well as for its constituent molecular weight components. Hb raffimer has an overall intravascular half‐life in the dog in the order of 25 hours, and that the larger molecular weight oligomeric fraction has the longest persistence. This dependence of disappearance rate on molecular weight is consistent with the findings of the Phase I study conducted in healthy human volunteers receiving Hb raffimer (Carmichael et al., [Citation2000]), in whom the oligomers were found to have a longer plasma retention time than the tetramers at each dose studied. Whereas unmodified stroma free hemoglobin solution is known to disappear from plasma rapidly, because of rapid filtration of the small molecular weight dimers, renal loss of Hb raffimer was minimal in the present experiments (). The longer intravascular persistence of the oligomers may be due either to a lesser kinetic likelihood, or reduced affinity of uptake into the principal site of clearance, presumed to be the reticulo‐endothelial system.
The half‐lives determined in dogs of Hb raffimer infused at 18 mL/Kg are substantially longer than the overall half‐life of 14.6 ± 3 hrs, and 7.6 ± 2.8 hrs for the tetrameric species and 19.5 ± 3.2 hrs for the oligomers in human subjects given 4–7 mL/kg (4–7 g/kg) (Carmichael et al., [Citation2000]). The reasons for the longer persistence in dogs than in humans may be related to the larger load in the former, but the experiments, in the absence of comparable loads, do not permit a prediction of the half‐life in humans at this load.
Methemoglobin Formation
The in vivo formation of MetHb would reduce the oxygen transporting capacity of cell‐free hemoglobin based oxygen therapeutics, and would thereby impair their physiological efficacy. Throughout the course of this experiment, plasma MetHb concentration remained at about 10% or less of the total plasma Hb and the mass concentration of MetHb remained at about 1 g/L, suggesting that excessive MetHb formation did not occur. MetHb formation of Hb raffimer in vivo was previously determined in rabbits by Caron et al. ([Citation2000]). In these experiments, Hb raffimer was administered as an exchange transfusion (13 mL/kg, or 1.3 g/kg) replacing 20% of the estimated blood volume, and animals were subsequently followed for three hours. Over the three hour observation period, total blood MetHb levels peaked at 2% in the Hb raffimer group and declined to 0.97 ± 0.37% at the end of the three hour observation period. Given that the whole blood hemoglobin concentration at that time was 123 ± 9 g/L, the MetHb mass‐concentration can be estimated to be 1.2 g/L. If all of this is assumed to be present as oxidized Hb raffimer in plasma, it would represent approximately 5% of the plasma Hb concentration of 22 g/L. MetHb formation in vivo was also studied in rats that had 90% of their blood volume replaced with Hb raffimer (Ning et al., [Citation1998]). Over a 3‐hour period, the plasma MetHb concentration in these rats rose from 6% at baseline, to 8.5% at the peak. Thus, data from three species (rat, rabbit and dog) with varying loads of Hb raffimer (20%, 25%, 90% of the blood volume) suggest that MetHb in vivo formation is limited after Hb raffimer administration.
In experiments with a large blood volume exchange in sheep (i.e. 90% of the estimated blood volume) (Lee et al., [Citation1995]), hemoglobin glutamer—250 [bovine] (Hemopure®, BioPure Corp, Cambridge MA), a bovine hemoglobin‐based oxygen carrier cross‐linked with gluteraldahyde, kept the animals alive and apparently maintained sufficient oxygen transport. However, MetHb accumulated in vivo, to the extent that after 24 hours plasma MetHb concentration exceeded 40%, and remained at that level, as the total plasma hemoglobin concentration declined by its clearance. This was not the case in the experiments on Hb raffimer reported here. MetHb concentration in the plasma of the dogs infused with Hb raffimer remained a small fraction of the total both as a fraction of the total pigment, and as mass‐concentration. While a number of possibilities may be suggested to account for what may be differences in findings between experiments utilizing Hb raffimer and Hb glutamer, no conclusions can be reached from the available evidence.
Interestingly, in a recently published paper, plasma hemoglobin and MetHb concentrations were measured after infusions of large doses of hemoglobin glutamer in bleeding surgical patients (Sprung et al., [Citation2002]). In this study, plasma MetHb concentration rose in a dose dependent manner, with peak concentrations on the third post‐operative day, of 3% or less when the dose was 1.5 g/kg (equivalent to 11.5 mL/kg) or less. The increase was 6–7% when the dose was 2.0 or 2.5 g/kg (representing 15.4 and 19.2 mL/kg), declining thereafter to minimal levels on the seventh post‐operative day. This suggests that adequate reducing mechanisms are present to prevent substantial increases in MetHb in humans.
In a review of the mechanisms promoting and preventing oxidation of free hemoglobin in plasma, Faivre et al. ([Citation1994]) noted the existence of non‐specific reducing agents, including ascorbic acid which is “cycled” between the red cell and plasma (McGown et al., [Citation1990]). In experiments in which dextran‐BTC‐hemoglobin (Faivre et al., [Citation1994]) was infused in guinea pigs, the fully reduced hemoglobin was oxidized to approximately 30–40% MetHb, whereas when fully oxidized MetHb was infused, 40% was reduced within 12 hours. These findings suggest that significant reducing capacity is present in whole blood, which prevents MetHb formation in Hb free in the plasma. This is also suggested by experiments conducted in vitro (Neya et al., [Citation1998]) in which hemoglobin glutamer was exposed to continuous oxygenation in cardiopulmonary bypass equipment for 5 hours in the presence or absence of blood. MetHb formation was quite rapid under these conditions, but the rate was reduced to about half in the presence of fresh human blood. Interestingly, the results of continuous exposure to a high oxygen concentration were reproduced in control experiments in which after an initial 3‐minute exposure, samples were held for 5 hours at 37°C. MetHb formed at similar rates in these samples, and the effect of the presence of fresh whole blood was also one of similar retardation of the oxidation.
The results of this study demonstrate that Hb raffimer does not exhibit a rapid rate of oxidation of the heme iron in vivo in the dog. Supporting literature reflects the same conclusion in rabbits and rats.
Acknowledgment
We gratefully acknowledge the staff of ITR Laboratories for their expert completion of this study.
References
- Brooks J. The oxidation of haemoglobin to methaemoglobin by oxygen II—The relation between the rate of oxidation and the partial pressure of oxygen. Proc. R. Soc. Lond. 1935; 547: 560–577
- Carmichael F. J., Ali A. C., Campbel J. A., Langlois S. F., Biro G. P., Willan A. R., Pierce C. H., Greenburg A. G. A phase I study of oxidized raffinose cross‐linked human hemoglobin. Crit. Care Med. 2000; 28: 2283–2292
- Caron A., Menu P., Faivre‐Fiorina B., Labrude P., Alayash A., Vigneron C. Systemic and renal hemodynamics after moderate hemodilution with HbOCs in anesthetized rabbits. Am. J. Physiol. Heart Circ. Physiol. 2000; 278: H1974–H1983
- Cohn S. M. Oxygen therapeutics in trauma and surgery. J. Trauma 2002, in press
- Faivre B., Menu P., Labrude P., Vigneron C. Do the dissociation of the dex‐BTC‐Hb and its oxidation influence the P50 in vivo in guinea pig?. Art. Cells Blood Substit. Immobil. Biotechnol. 1994; 22: A97
- Guidiotti G. Studies on the chemistry of hemoglobin. J. Biol. Chem. 1967; 242: 3685–3693
- Lee R., Neya K., Svizzero T. A., Vlahakes G. J. Limitations of the efficacy of hemoglobin‐based oxygen‐carrying solutions. J. Appl. Physiol. 1995; 79(1)236–242
- McGown E. L., Lyons M., Zegna A. Reduction of extracellular methemoglobin by erythrocytes. Biochim. Biophys. Acta 1990; 1036: 202–206
- Neya K., Lee R., Vlahakes G. J. Hemoglobin based oxygen carrying solution stability in extracorporeal circulation: an in vitro evaluation and implications for clinical use. ASAIO J. 1998; 44(3)166–170
- Ning J., Er S. S., Wong L. T. In vivo oxygenation of deoxy‐HEMOLINK™ following exchange transfusions of 50% or 90% the blood volume in rats. Blood Substitutes—Present and Future Perspectives, E. Tsuchida. Elsevier Science, New York 1998; 211–223
- Scott E. M., Duncan I. W., Ekstrand V. The reduced pyridine nucleotide dehydrogenases of human erythrocytes. J. Biol. Chem. 1965; 240: 481–485
- Sprung J., Kindscher J., Wahr J. A., Levy J. H., Monk T. G., Moritz M. W., O'Hara P. J. The use of bovine hemoglobin glutamer‐250 (Hemopure®) in surgical patients: results of a multicenter, randomized, single‐blinded trial. Anesth. Analg. 2002; 94: 799–808