Abstract
Watermelon α‐galactosidase (EC 3.2.1.22) was immobilized on a natural (chitin) and a synthetic anion‐exchange (Amberlite IRA‐938) support by covalent coupling methods. The procedure entails the activation of supports with 1,1′‐carbonyldiimidazole (CDI), followed by immobilization of the enzyme on to these supports without and with a spacer arm; γ‐aminobutyric acid (GABA). Optimization of activation was performed by changing the CDI concentrations and coupling efficiencies. The comparison of two immobilization techniques for both chitin and Amberlite IRA‐938 was made by comparing different enzyme concentrations against enzyme activity yield. Furthermore, the storage stability of the immobilized enzymes was also investigated and chitin immobilized α‐galactosidase was found to be better. Although the activity yield of immobilized enzymes were the same for both supports, the short storage stability of immobilized enzyme on Amberlite IRA‐938 is currently a drawback to its applications.
Introduction
α‐Galactosidases (α‐D‐galactoside galactohydrolase, EC 3.2.1.22) catalyze hydrolysis of simple and complex oligo‐ and polysaccharides containing α‐1,6‐linked terminal galactosyl groups, which are widely distributed in nature (Kotwall et al., [Citation1999]). They have useful applications in food processing (Mansour and Khalil, [Citation1998]; Shivanna et al., [Citation1989]; Somiari and Balogh, [Citation1993]), the sugar industry (Ohtakara and Mitsutomi, [Citation1987]; Porter et al., [Citation1990]), medicine (Citation[[Balasubramaniam and Mathew, 1986]]; Coppola et al., [Citation1994]; Ito et al., [Citation1993]; Wong et al., [Citation1986]), enzymatic synthesis (Golubev and Neustroev, [Citation1993]; Hashimoto et al., [Citation1993]), and structural analysis and elucidation of the biological functions of complex carbohydrates (Gidley et al., [Citation1992]; Ibatullin et al., [Citation1993]). They have been isolated and purified from various microorganisms (Scigelova and Crout, [Citation2000]; Telefoncu et al., [Citation1998]; Zeilinger et al., [Citation1993]), mammalian tissues (Bishop and Desnick, [Citation1981]; Dhar et al., [Citation1993]) and plants (Haibach et al., [Citation1991]; Mansour and Khalil, [Citation1998]; Pressey, [Citation1984]; Zhu et al., [Citation1995]) using different techniques.
The immobilization of enzymes offers several advantages over the use of soluble enzyme preparations including; easier separation of products from the incubation mixture, the ability to recover and reuse the enzyme catalyst, stabilization of tertiary structure and increased storage stability. These advantages have been of particular value for enzymes and enzyme systems that are difficult to isolate, are unstable and for applications in industry, medical science etc. Therefore, investigation of various supports for enzyme immobilization have broad implications. The immobilization of enzymes on inert, insoluble materials is currently a very active area of research. In this work, we have studied the immobilization of watermelon (Citrullus vulgaris) α‐galactosidase on a natural (chitin) and a synthetic (Amberlite IRA‐938) support using covalent coupling methods. Chitin is a hydrophilic, inert, biocompatible, inexpensive and natural polymer (Illanes, [Citation1994]; Iyengar and Prabhakara, [Citation1979]) and has been frequently used as a solid support for enzyme immobilization (Batra and Gupta, [Citation1994]; Ge et al., [Citation1996]; Mitsutomi et al., [Citation1985]; Spagna et al., [Citation1998]). The utilization of ion‐exchangers as synthetic solid support for enzyme immobilization have also been widely reported (Kurota et al., [Citation1990]; Thompkinson, [Citation1989]). Amberlite IRA‐938 is a macroporous anion‐exchange resin consisting of a polystyrene‐divinylbenzene matrix. It is very stable in acidic and alkali media and also resistant to chemical attacks.
The aim of this study is to optimize and compare the two supports in order to provide an alternative to other methods previously tested for the immobilization of α‐galactosidase. Furthermore, the storage stability of the immobilized enzyme was also investigated by comparing with the stability of free enzyme.
Materials and Methods
Materials
p‐Nitrophenyl‐α‐D‐galactopyranoside (PNPG), 1,1′‐carbonyldiimidazole (CDI), 1‐ethyl‐3(3‐dimethylaminopropyl)carbodiimide (EDC) and chitin were purchased from Sigma Chemical Co. (St. Louis, USA). The anion‐exchange resin (Amberlite IRA‐938) was the product of Rohm and Haas Co. (Darmstadt, FRG) and γ‐aminobutyric acid (GABA) was purchased from Fluka Chemie AG (Buchs, Switzerland). Watermelons (Citrullus vulgaris) were obtained from local markets. All other chemicals and reagents were obtained from local suppliers and were analytical grade or better.
Methods
Extraction of α‐Galactosidase
After the removal of seeds and peel, the watermelon fruit was cut into small pieces and homogenized (Silverson STL‐2, UK). The homogenate was decanted through two layers of cheese‐cloth and centrifuged (Hettich 30 RF, FRG) at 10 000 rpm for 15 min. The pH of the resulting supernatant was adjusted to pH 6.0 and subjected to 85% ammonium sulfate precipitation. The mixture was allowed to stand overnight with continuous stirring. The precipitate was then collected by centrifugation at 10 000 rpm for 30 min, dissolved in distilled water and dialysed overnight against distilled water. In order to remove the insoluble matter, dialysate was centrifuged at 4 000 rpm for 5 min and then subjected to 25–85% ammonium sulfate precipitation overnight with continuous stirring. All steps for the preparation of the partially purified α‐galactosidase were carried out at 4°C. The resultant precipitate was dissolved, dialysed against water and kept at − 20°C until immobilization (Önal, [Citation2000]).
Enzyme Immobilization
Watermelon α‐galactosidase was immobilized on chitin and Amberlite IRA‐938 using two different methods. Following the activation of supports with 1,1′‐carbonyldiimidazole (CDI), α‐galactosidase was immobilized on chitin and Amberlite IRA‐938 without and with a spacer arm, γ‐aminobutyric acid (GABA).
Method A
Chitin (1 g) was washed several times with distilled water and Amberlite IRA‐938 (1 g) was soaked in 25 ml of distilled water for 24 hours at room temperature. The supports were washed sequentially with 25 ml of water, dioxane:water (3:7), dioxane:water (7:3) and suspended in 15 ml of anhydrous dioxane. Different amounts of 1,1′‐carbonyldiimidazole (CDI) (0.15–1.0 mmol CDI/g support) was added to the suspension and then was agitated gently at room temperature for 3 hours. In order to remove liberated imidazole, the supports were washed with anhydrous dioxane and then used for enzyme immobilization. The treated supports were added to α‐galactosidase preparation in 10 ml of distilled water (pH 6.0) with gentle shaking (200 rpm) at 4°C overnight. The resultant preparate was separated by filtration and washed with 25 ml of distilled water, 12.5 ml of 0.5 M NaCl and 25 ml of distilled water, respectively. The washing solutions were kept for enzymatic activity and protein determination.
Method B
The CDI‐activated chitin and Amberlite IRA‐938 were prepared according to Method A. 0.1 g γ‐aminobutyric acid (GABA) in 12.5 ml water (pH 10.0) was added to the activated supports and incubated overnight at 4°C. The GABA bound supports were washed with 25 ml distilled water, 12.5 ml 0.5 M NaCl and 25 ml distilled water, respectively and filtered. The GABA bound support was mixed by 0.08 g of 1‐ethyl‐3(3‐dimethylaminopropyl)carbodiimide (EDC) in 50 ml 0.1 M acetate buffer (pH 5.0) was filtrated and washed with 100 ml of 0.1 M acetate buffer (pH 5.0) and gently stirred at room temperature for one hour. Then, EDC treated matrix was filtrated and washed with 100 ml of 0.1 M acetate buffer (pH 5.0) in order to remove the excess of reagents. α‐Galactosidase was treated with matrix by gently shaking (200 rpm) at 4°C overnight. The immobilized enzyme was separated by filtration, washed twice with 25 ml 0.1 M acetate buffer (pH 5.0) and assayed.
Active Group Determination
In order to determine the active group yield of the supports, CDI‐activated supports were coupled via a spacer arm (GABA) according to Method B. The GABA bound supports, which contained carboxylic groups, were soaked twice in 50 ml of 0.1 M HCl for 30 min and filtered. The active group yield were determined by the titration of the supports with 0.01 M NaOH . The activity yield(%) was calculated by; Activity yield(%) = Active group yield/Reactive concentration × 100 equation.
Enzyme Assay
The α‐galactosidase activity of the native enzyme was assayed in a reaction mixture consisting of 0.25 ml 2.0 mM p‐nitrophenyl‐α‐D galactopyranoside, 0.5 ml 50 mM citrate buffer (pH 6.0) and 0.25 ml enzyme solution. After incubation for 30 min at 37°C, the reaction was stopped by the addition of 3.5 ml of 0.2 M borate buffer (pH 9.8). The quantity of liberated p‐nitrophenol was measured at 400 nm.
Immobilized enzyme activity was determined using (0.05–0.1 g) immobilized enzyme) in a total volume of 1 ml reaction mixture indicated above. The reaction was stopped by the addition of borate buffer (0.2 M, pH 9.8), then the mixture was centrifuged and assayed.
One unit of enzyme activity (Unit) was defined as the amount of enzyme which released one μmol of p‐nitrophenol per min at pH 6.0 and 37°C.
Protein Determination
Protein contents of enzyme preparations and the washings were determined by the method of Lowry et al. ([Citation1951]) using bovine serum albumin as standard.
Storage Stability
The storage stability of the immobilized enzyme was determined in terms of the activity survival ratio after 7 months of storage at 4°C. Aliquots were withdrawn with time and their activities against PNPG assayed and compared with that of free enzyme.
Results and Discussion
For the covalent immobilization of an enzyme on to an inert support, two discrete chemical processes are required. Initially, the activation of support with an appropriate agent and secondly, coupling of enzyme to the activated support. Enzyme can be chemically coupled to an activated support either directly or via a spacer arm. We preferred to use 1,1′‐carbonyldiimidazole (CDI) for the activation step because of its advantages: CDI is a carbonylating reagent and has been shown to be suitable for the activation of free hydroxyl groups on supports (Bethell et al., [Citation1979]; Hearn, [Citation1987]). An important advantage of the CDI method compared to the standard cyanogen bromide method is the absence of any charged groups introduced by the functional groups of the activation reagent during both the activation and ligand coupling steps as assessed over the pH range normally used. Beside this, CDI is a non toxic reagent and reaction could be handled under mild conditions.
The intermediate activated matrix reacts smoothly with N‐nucleophiles such as free amino groups in enzymes. Sometimes coupling a ligand to a CDI activated support via a spacer may have the effect of increasing the efficiency of the ligand. Therefore, we have coupled α‐galactosidase to CDI‐activated supports (chitin and Amberlite IRA‐938) directly and via a spacer arm according to Method A and Method B, respectively. The reaction schemes are given in .
Figure 1. a) CDI activation of the support, b) Enzyme coupling without spacer arm (Method A), c) Enzyme coupling via spacer arm (Method B).
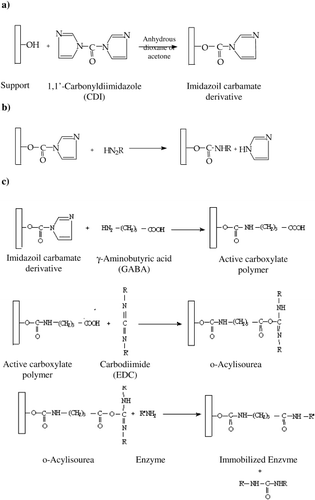
Optimization of α‐Galactosidase Immobilization
Chitin and Amberlite IRA‐938 were activated by the use of various amounts of CDI (0.15, 0.3, 0.6 and 1.0 mmol CDI per gram support) (). As shown in , the highest activation yield (93%) and active group yield (0.14 meq/g chitin) were obtained with 0.15 mmol CDI/g chitin. Although the highest activation yield (88%) was obtained with 0.15 mmol CDI/g Amberlite IRA‐938, the highest active group yield (0.243 meq/g Amberlite IRA‐938) was found to be 0.6 mmol CDI/g Amberlite IRA‐938. Various activation levels ranging from 10 to 4000 μmol active groups per g solid matrix can be rapidly and reproducibly achieved with carbonylating reagents under controlled conditions (Bethell et al., [Citation1979]; Hearn, [Citation1987]). However, the appropriate choice of activation level should be optimized, because highest activation levels of support materials does not provide the best binding yield. For this reason, we have coupled the same amount of enzyme to the activated supports in order to determine the optimum activation and coupling conditions. The highest activity yields were found to be 67% with 1 mmol CDI/g matrix for chitin and 68% with 0.3 mmol CDI/g matrix for Amberlite IRA‐938, repectively (Tables and ).
Table 1. Activation yields of chitin and Amberlite IRA‐938 with various amounts of CDI
Table 2. Coupling parameters for the immobilization of α‐galactosidase on CDI activated chitin (A) and Amberlite IRA‐938 (B)
A comparison of with shows that, when the coupled enzyme amount/active group yield ratio is low, the activity yield is generally medium. However, when the ratio is high, the corresponding yield is low.
When we compared the supports, which have the same active group yield, the higher activity yields were obtained by chitin. However, coupled protein per active group was lower. This may be due to the hydrophilic character and porous structure of chitin, meaning an increase in the protein surface area in contact with the support surface and the probable number of linkages. The data for Amberlite IRA‐938 showed that the specific activity of the immobilized enzyme can be changed according to the activation yield of the support. If the number of reactive groups on the support is very high, the enzyme molecules can be coupled to the support surface via a lot of arms and this may restrict the three‐dimensional structure of the enzyme. The activation levels at 0.3 mmol CDI/g Amberlite IRA‐938 and 0.6 mmol CDI/g Amberlite IRA‐938 were found to be 0.163 meq/g Amberlite IRA‐938 and 0.243 meq/g Amberlite IRA‐938, respectively. Although both resins had different activation levels, they can couple to the same amount of protein (3.48 and 3.52 mg protein/g support) (). The specific activity of the first preparate (0.3 mmol CDI/g) confirm these results. In the case of chitin, 0.15 mmol CDI/g chitin and 1 mmol CDI/g chitin gave 0.140 meq/g chitin and 0.139 meq/g chitin, respectively. However, they coupled to different amounts of protein ().
According to the activation results, 1 mmol CDI/g chitin and 0.3 mmol CDI/g Amberlite IRA‐938 were chosen and then different amounts of protein (0.5–10 mg for chitin and 2.5–20 mg for Amberlite IRA‐938) were tested for the investigation of the high immobilization yield by applying Method A to these supports. The results showed that, 1 mg protein/g chitin and 5 mg protein/g Amberlite IRA‐938 gave 67% and 68% activity yields ().
Table 3. Effect of protein amounts on the coupling efficiency of CDI activated chitin (A) and Amberlite IRA‐938 (B)
Low immobilization yields were obtained by both supports containing GABA as spacer arm (). This may be explained by the increase of undesired hydrophobic interactions caused by spacer arm during sorption of proteins.
Table 4. Effect of protein amounts on the coupling efficiency of CDI activated chitin (1 mmol CDI/ g chitin) [A] and Amberlite IRA‐938 (0.3 mmol CDI/g resin) [B] with spacer arm(GABA)
The coupling of enzyme to chitin without a spacer arm (Method A) had the best storage stability. It retained 59% of its initial activity after 7 months of storage (A). However, the enzyme immobilized on Amberlite IRA‐938 by the same procedure, lost its activity quickly in 8 days (nearly 50%) and thereafter it retained 36% of its initial activity (B). All of these results showed that chitin‐immobilized α‐galactosidase was considerably more stable than the free enzyme and could be stored for extended periods before use. Various reports confirm that the storage stability of enzyme vary depending on the immobilization method applied and on the character of covalent coupling to the support (Krajewska et al., [Citation1990]).
Figure 2. Storage stability of free and immobilized α‐galactosidases at 4°C. (•): free enzyme, (□) immobilized enzyme : on chitin (A), on Amberlite IRA‐938 (B).
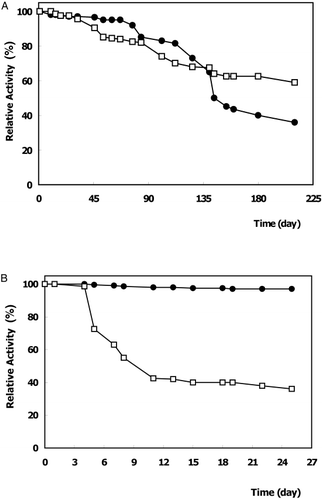
Several results are noncomparable because there is no known references to us about immobilization of the watermelon α‐galactosidase on these supports. However, CDI activated supports are frequently used as biospecific affinity supports in the purification of enzymes and other proteins or in immobilization of enzymes. These results demonstrate that chitin‐enzyme conjugate (activation level: 1 mmol CDI/g chitin, active group yield: 0.139 meq/g chitin, coupling efficiency: 1 mg protein/g chitin, activity yield: 67%) can be utilized with a high degree of activity over a period of many months. The immobilized enzyme also showed good properties and stabilities which are very important parameters especially in industrial applications (data not shown).
Conclusions
In this study we selected watermelon as a source of α‐galactosidase because of its various advantages. It is readily available in Turkey, very cheap and it contains very high α‐galactosidase activity. The contaminant glycosidases can be easily removed by partial purification procedures. Furthermore, the enzyme found in watermelon was very stable.
The results of this study show that α‐galactosidase can be successfully immobilized on CDI activated chitin using a direct coupling procedure which offers certain advantages: Chitin is relatively cheap, inert and suitable for enzyme immobilization. The proposed immobilization procedure is simple to carry out. The activity yield of immobilization and the storage stability of the immobilized enzyme offer potential for use in industrial processes.
Acknowledgment
The authors are grateful for the financial support provided by the Ege University Research Foundation under project FEN 95/025.
References
- Balasubramaniam K., Mathew C. D. Purification of α‐galactosidase from coconut. Phytochemistry 1986; 25(8)1819–1821
- Batra R., Gupta M. N. Non covalent immobilization of potato (Solanum tuberoscum) polyphenoloxidase on chitin. Biotechnol. Appl. Biochem. 1994; 19: 209–215
- Bethell G. S., Ayers J. S., Hancok W. S. A novel method of activation of crosslinked agaroses with 1,1′‐carbonyldiimidazole (CDI) which gives a matrix for affinity chromatography devoid of additional charged groups. J. Biol. Chem. 1979; 254: 2572–2574
- Bishop D. F., Desnick R. J. Affinity purification of α‐galactosidase from human spleen placenta and plasma with elimination of pyrogen contamination. J. Biol. Chem. 1981; 256: 1307–1316
- Coppola G., Yan Y., Hantzopoulos P., Segura E., Stroh J. G., Calhoun D. H. Characterization of glycosylated and catalytically active recombinant human α‐galactosidase A, using a baculovirus vector. Gene 1994; 144: 197–203
- Dhar G. M., Mitsutomi M., Ohtakara A. Purification and characterization of Phaseolus vulgaris α‐galactosidase izozymes. Bull. Fac. Agric. 1993; 74: 59–68
- Ge S., Bai H., Zhang L. Ttyripsin immobilization on shrimp chitin with formaldehyde and its application to continious hydrolysis of casein. Biotechnol. Appl. Biochem. 1996; 24: 1–5
- Gidley M. J., Eggleston G., Morris E. R. Selective removal of α‐galactosidase side chains from Rhizobium capsular poysaccharide by guar α‐galactosidase: effect on conformational stability and gelatin. Carbohydr. Res. 1992; 231: 185–196
- Golubev A. M., Neustroev K. N. Crystallization of α‐galactosidase from Trichoderma reesei. J. Mol. Biol. 1993; 231: 3933–3934
- Haibach F., Hata J., Mitra M., Dhar M., Harmata M., Sun P., Smith D. Purification and characterization of a Coffea canephora α‐galactosidase isozyme. Biochem. Biophys. Res. Commun. 1991; 181: 1564–1571
- Hashimoto H., Katayama C., Goto M., Kitahata S. Purification and some properties of α‐galactosidase from Candida guilliermondii H 404. Biosci. Biotechnol. Biochem. 1993; 57: 372–378
- Hearn M. T.W. Methods in Enzymology, 1,1′‐Carbonyldiimidazole Mediated Immobilization of Enzymes and Affinity Ligands, K. Moshbach. Academic Press, New York 1987; Vol. 135: 102–117, Part 7
- Ibatullin F. M., Golubev A. M., Firsov L. M., Neustroev K. N. Model for cleavage of O‐glycosidic bond in glycoproteins. Glycoconj. J. 1993; 10: 214–218
- Illanes A. Advances in Bioprocess Engineering, Chitin as a Matrix for Enzyme Immobilization, E. Galindo, O. T. Ramirez. Kluwer Academic Publishers, Netherlands 1994; 461–466
- Ito N., Tabata S., Kawahara S., Hirano Y., Nakajima K., Uchida K., Hirota T. Histochemical analysis of blood group antigen in human sublingual glands and pancreas. Histochem. J. 1993; 25: 242–249
- Iyengar L., Prabhakara R. A.V.S. Urease bound to chitin with glutaraldehyde. Biotechnol. Bioeng. 1979; 21: 1333–1343
- Kotwall S. M., Gote M. M., Khan M. I., Khire J. M. Production, purification and characterization of constitutive intracellular α‐galactosidase from the thermophilic fungus Humicola sp. J. Ind. Microbiol. Technol. 1999; 23: 661–667
- Krajewska B., Leszko M., Zaborska E. Urease immobilized on chitosan membrane: preparation and properties. J. Chem. Technol. Biotechnol. 1990; 48: 337–350
- Kurota A., Kamata Y., Yamauchi F. Enzyme immobilization by the formation of enzyme coating on small pore size ion exchangers. Agric. Biol. Chem. 1990; 54: 1557–1558
- Lowry O. H., Rosebrough N. J., Farr A. L., Randall R. J. Protein measurement with the folin phenol reagent. J. Biol. Chem. 1951; 193: 265–275
- Mansour E. H., Khalil A. H. Reduction of raffinose oligosaccharides in chick pea (Cicer arientinium) flour by crude extracellular fungal α‐galactosidase. J. Sci. Food Agric. 1998; 78: 175–181
- Mitsutomi M., Uchida Y., Ohtakara A. Immobilization of thermostable α‐galactosidase from Pycnoporous cinnabarinus on chitin and some properties of the immobilized enzyme. J. Ferment. Technol. 1985; 63: 325–329
- Ohtakara A., Mitsutomi M. Immobilization of thermostable α‐galactosidase from Pycnoporus cinnabarinus on chitosan beads and its application to the hydrolysis of raffinose in beet sugar molasses. J. Ferment. Technol. 1987; 65: 493–498
- Önal S. Immobilization of watermelon (Citrullus vulgaris) α‐galactosidase on natural and synthetic supports. PhD. Thesis. 2000
- Porter J. E., Herrmann K. M., Ladisch M. R. Integral kinetics of α‐galactosidase purified from Glycine max for simultaneous hyrolysis of stachyose and raffinose. Biotechnol. Bioeng. 1990; 35: 15–22
- Pressey R. Tomato α‐galactosidases: conversion of human type B erytrocytes to type O. Phytochemistry 1984; 23: 55–58
- Scigelova M., Crout D. H.G. Purification of α‐galactosidase from Aspergillus niger for application in the synthesis of complex oligosaccharides. J. Mol. Catal., B Enzym. 2000; 8: 175–182
- Shivanna B. D., Ramakrishna M., Ramadoss C. S. Enzymatic hydrolysis of raffinose and stachyose in soybean milk by α‐galactosidase from germinating guar (Cyamopsis tetragonolobus). Process Biochem. 1989; 197–199, December
- Somiari R., Balogh E. Effect of soaking, cooking and crude α‐galactosidase treatment on the oligosaccharide content of cowpea flours. J. Sci. Food Agric. 1993; 61: 339–343
- Spagna G., Andreani F., Salatelli E., Romagnoli D., Pifferi P. G. Immobilization of α‐L‐arabinofuranosidase on chitin and chitosan. Process Biochem. 1998; 33: 57–62
- Telefoncu A., Zihnioðlu F., Tatar Önal S., Dinçkaya E., Denizci A. Purification and characterization of α‐galactosidase from Thermus thermophilus HB8. J. Fac. Sci. (Ýzmir) 1998; 21: 55–67
- Thompkinson D. K. Immobilize enzyme systems for β‐galactosidase. Ind. J. Dairy Sci. 1989; 42: 787–791
- Wong H., Hu C., Yeh H., Su W., Lu H., Lin C. Production, purification and characterization of α‐galactosidase from Monascus pilosus. Appl. and Environ. Microbiol. 1986; 1147–1152, November
- Zeilinger S., Kristufek D., Atac I. A., Hodits R., Kubicek C. P. Conditions of formation, purification and characterization of α‐galactosidase of Trichoderma reesei RUT C‐30. Appl. Environ. Microbiol. 1993; 59: 1347–1353
- Zhu A., Wong Z. K., Goldstein J. Identification of tyrosine 108 in coffea bean α‐galactosidase as an essential residue for the enzyme activity. Biochim. Biophys. Acta 1995; 1247: 260–264