Abstract
The bone marrow contains mesenchymal cells that can be divided into two categories: cells of hemopoietic lineage and stromal cells. The stromal cells are adhesive to the surface of culture dish, and could be differentiated into cells with bone‐forming capability when stimulated with osteogenic supplements. In this study, we have employed collagen to immobilize cells with osteogenic potential from bone marrow. A more than two‐fold increase in cell density was obtained on the collagen‐coated substratum as compared to the uncoated ones. The selected marrow cells exhibited elevated alkaline phosphatase activity in parallel with the proliferation of the cells attached to the collagen surface. The osteoblastic expression of the selected cells was further confirmed by the histological stains of alkaline phosphatase and mineral deposit. This method provides a simple and fast screening technique to isolate osteoprogenitor‐enriched population from the bone marrow stromal cells. It has a great potential for future biological and clinical applications.
Introduction
The bone marrow contains mesenchymal cells that can be differentiated into a variety of cells including the osteoprogenitor cells (Owen, [Citation1985]; Weiss, [Citation1976]). Previous studies have demonstrated that the bone marrow formed an osteogenic tissue after implanted (Ashton et al., [Citation1980]; Bab et al., [Citation1986]; Mardon et al., [Citation1987]). The in vitro experimental results of cell culturing also confirmed that the bone marrow cells could be induced into the osteogenic lineage (Benayahu et al., [Citation1989]; Maniatopoulos et al., [Citation1988]). These findings suggest that bone marrow contains osteoprogenitor cells.
Type I collagen is the most abundant protein existing in the extracellular matrix (ECM). The nucleation of the newly formed minerals of the bone is believed to occur in the matrix of collagen fibers (Mundy [Citation1998]). This is supported by the findings that extensive mineralization was formed by osteoblastic cells when grown on collagen substrata (Andrianarivo et al., [Citation1992]; Lynch et al., [Citation1995]; Mizuno et al., [Citation2000]). Furthermore, collagen contains at least two binding sites with different sequence of amino acid for binding to cell surface receptors. Therefore, collagen appears to be a potential candidate as the coating material to selectively isolate osteoprogenitor cells from bone marrow.
In this study, two collagen matrices—reconstituted hydrated collagen matrix and air‐dried collagen matrix were used to immobilize the osteoprogenitor cells from bone marrow. By elution of the loosely attached cells from the surface, we could select a cell population enriched in osteoprogenitor from the bone marrow. This process allows us to obtain cells with osteogenic activity in an effective way as compared with that used in the conventional method. This method will be invaluable for biological and clinical applications.
Materials and Methods
The Dulbecco's modified Eagle's medium (DMEM) and fetal bovine serum were purchased from GIBCO (Grand Island, NY). The alkaline phosphatase activity staining kit was purchased from Sigma (St. Louis, MO). All of the chemicals used were of reagent grade.
Preparation of Collagen Substratum
Type I collagen was prepared from the lipid‐depleted bovine skin with 0.5 M acetic acid and pepsin treatment as described in the previous paper (Hsu et al., [Citation2000]). The collagen was stored in 0.5 M acetic acid at 4°C.
The coating of collagen on culture dish was conducted as follows. The culture dish surface was covered with collagen solution (300 μl, 100 μg/ml) and incubated at 37°C for 2 hrs. By this method, a layer of hydrated collagen consisting of reconstituted collagen fibers was formed on the dish surface (Grinnell and Bennett, [Citation1982]). This collagen‐coated dish was used for the adhesion of bone marrow stromal cells. Alternatively, the hydrated collagen was dried under laminar flow to obtain an air‐dried film of collagen before being used for cell adhesion experiment.
Preparation and Culturing of Bone Marrow Cells
Rat bone marrow cells were prepared and harvested according to the procedure described by Maniatopoulus et al. ([Citation1988]). Briefly, the femurs of SD rats (male, age of 6 week old) were dissected aseptically and the inside bone marrow cells were flushed out with a buffer using a 5‐ml syringe into a dish.
Approximately 2 ml of bone marrow cells (8 × 106 cells/ml) were seeded onto the 6‐well culture dishes coated with or without collagen. The cells were incubated in DMEM (supplemented with 10% fetal bovine serum, 50 μg/ml ascorbic acid, 10 mM β‐glycerophosphate, 10− 8 M dexamethasone and 50 μg/ml gentamycin) for 3 hr at 37°C. The unattached cells were then removed from the dish surface by flushing with PBS several times. The remaining attached cells were cultured in DMEM supplemented with the same components as mentioned above at 37°C in a CO2 incubator. At various times, cells were detached from the matrix by trypsinization. The viable cells were determined under a microscope by the method of trypan blue exclusion.
Alkaline Phosphatase Activity Assay
The alkaline phosphatase activity was determined according to the method of Citation[[Lowry (1955)]. The activity was expressed by the amount of p‐nitrophenol liberated was determined by measuring the light absorbance at 405 nm.
Histological Stain of Alkaline Phosphatase and Mineral‐Deposit
The adherent cells were fixed with citrate‐buffered acetone for 45 seconds and rinsed with de‐ionized water (Kaplow, [Citation1963]). The cells were then incubated for 30 minutes in dark with a mixture containing nathphol AS‐MX phosphate alkaline solution and fast red violet LB salt. After washing in running tap water, the specimens were left to air dry.
The von Kossa staining technique (McGee‐Russel, [Citation1958]) was used to detect the mineral deposited in the ECM of the cells. The cells were fixed with 2% glutaraldehyde in PBS and rinsed with deionozed water. They were then treated with 1% silver nitrate solution and kept for 1 hour under UV light. The fixed specimen was then rinsed with water and reacted with 5% sodium thiosulphate for 5 min, washed with water, and air‐dried.
Results and Discussion
The adhesion of rat femur bone marrow cells on both hydrated and air‐dried collagen matrices were tested and compared to that on the uncoated culture dish. shows that after moderate PBS washing, the number of bone marrow cells adhered to the collagen substratum is about twice that attached to the culture dish without collagen coating. There is no statistical difference between the numbers of the cell attached to the two different collagen matrices. For the growth profile, the attached cell proliferated at slow rate initially with cell density maintaining at about 4 × 104 cells/cm2. However, the cell population increases substantially after 3 days of culturing to approximate 8 × 104 cells/cm2 on the 7th day. On the other hand, the cell density on the uncoated dish increased from 0.8 × 104 cells/cm2 to 4 × 104 cells/cm2, only half of the cell density on the collagen‐coated surface, after 7 days of culturing.
Figure 1. The cell numbers were counted during the culture periods. Collagen‐coated dishes are reconstituted in incubator at 37°C (•) or let dried under laminar flow (♦).
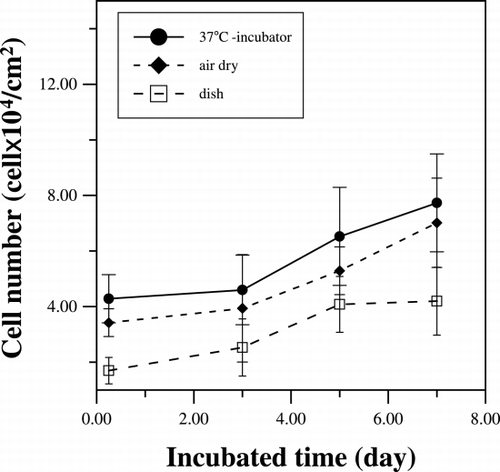
The expression of the activity of alkaline phosphatase (ALP) of the attached cells also had a lag time of three days and then increased to a high level after 3 days of culturing. Because ALP is believed to involve in the bio‐mineralization process of bone matrix, the same period of time lag of ALP expression and cell population indicates that the attached cells are enriched in osteogenitor cells. Corresponding to their proliferation profile, the cells grown on the untreated dishes showed much lower activity of 0.17 mM/hr as compared to the 0.4 mM/hr detected for the cells attached on the collagen matrix (). The histological evidence of the expression of ALP activity of the attached cells is shown in . As demonstrated, almost all of the cells were positive for alkaline phosphatase activity staining after 7 days of culture. This result indicated that the selectively adhered cells could be induced to express the marker protein of osteogenic cells when grown on a collagen based surface.
Figure 2. The alkaline phosphatase activities were measured during the culture periods. Collagen‐coated dishes are reconstituted in incubator at 37°C (•) or let dried under laminar flow (♦).
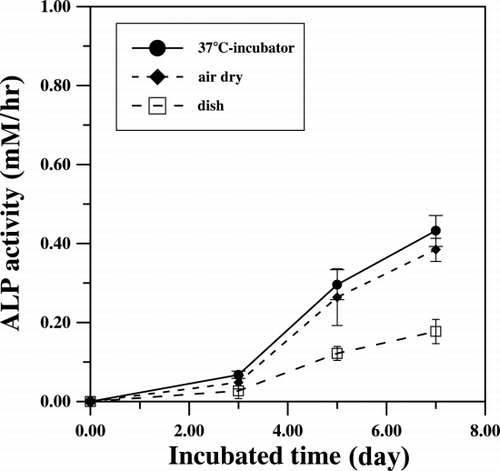
Figure 3. Alkaline phosphatase staining of stromal cells that grown on collagen‐coated glass plate for 7‐days. The presence of alkaline phosphatase in the cytoplasm is displayed as violet red color.
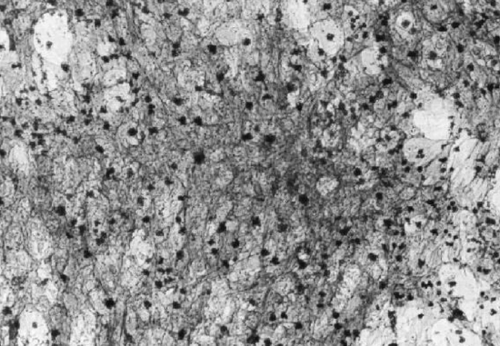
A significant number of morphologically distinctive nodules appeared after 10 days of culturing on the collagen‐coated glasses. Cells associated with these nodules were more cuboidal than the dispersed cells grown on the uncoated dishes. The most prominent phenotypic expression of the osteogenic cells is the deposit of mineral onto the collagenous matrix, which can be detected by von Kossa staining. shows the results of the von Kossa staining on the cells that adhered and grown on the untreated‐ as well as the collagen‐coated dishes. No apparent mineral deposit was found on the ECM of the cells grown in conventional untreated dishes after 2 weeks of culture (A). On the contrary, cells formed nodules and appeared opaque when grown on the collagen‐coated surface (B). These nodules could be stained intensively with von Kossa staining; clearly demonstrated the accumulation of mineral in the ECM of these cells.
Figure 4. The von Kossa staining of the cells on conventional dish (A) and collagen‐coated dish (B) after 2 weeks culture.
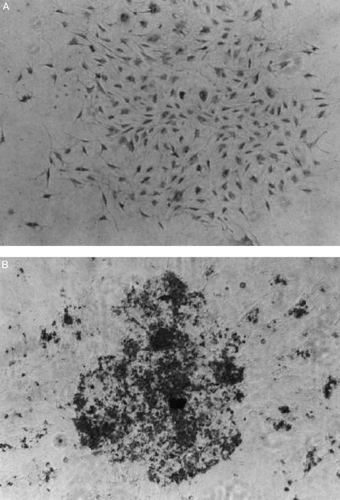
The bone marrow contains cells of hemopoietic lineage and stromal cells. Because the pluripotent stromal cells can be differentiated into various cells such as fibroblasts, chondrocytes and adipocytes (Bruder et al., [Citation1994]), an extensive culturing with conditions to suppress the growth and differentiation of other types of cells is required to enrich the osteoblastic cells in the adherent cell population (Benayahu et al., [Citation1989]; Maniatopoulos et al., [Citation1988]). In this study, we have found that both the hydrated collagen and collapsed air‐dried collagen exhibited about the same capability of immobilize the osteoprogenitor cells from bone marrow. Thus we have developed a method of using collagen‐coated surfaces to selectively enrich the osteogenic cells from bone marrow. This method takes about two weeks from bone marrow stromal cells to osteoblastic phenotype expression. This is much shorter period as compared with the culturing time (about 3 weeks) attained by the conventional method. The current method of is useful to purify the osteogenic cells form bone marrow for biological and clinical applications.
Acknowledgment
This work was supported in part by Grants from National Science Council (NSC), Taiwan, P.R. China.
References
- Andrianarivo A. G., Robinson J. A., Mann K. G., Tracy R. P. Growth on type I collagen promotes expression of the osteoblastic phenotype in human osteosarcoma MG‐63 Cells. J. Cell Pysiol. 1992; 153: 256–265
- Ashton B. A., Allen T. D., Howlett C. R., Eaglesom C. C., Hattori A., Owen M. Formation of bone and cartilage by marrow stromal cells in diffusion chambers in vivo. Clin. Orthop. Relat. Res. 1980; 151: 294–307
- Bab I., Ashton B. A., Gazit D., Marx G., Williamson M. C., Owen M. E. Kinetic and differentiation of marrow stromal cells in diffusion chambers in vivo. J. Cell Sci. 1986; 84: 139–151
- Benayahu D., Kletter Y., Zipori D., Wientroub S. Bone marrow‐derived stromal cell line expressing osteoblastic phenotype in vitro and osteogenic capacity in vivo. J. Cell Physiol. 1989; 140: 1–7
- Bruder S. P., Fink D. J., Caplan A. I. Mesenchymal stem cells in bone development, bone repair, and skeletal regeneration. J. Cell Biochem. 1994; 56: 529–539
- Grinnell F., Bennett M. H. Ultrastructural studies of cell‐collagen interactions. Method. Enzymol. 1982; 82: 535–544
- Hsu F. Y., Tsai S. W., Wang F. F., Wang Y. J. The collagen‐containing alginate/poly(L‐lysine)/alginate microcapsules. Art. Cells, Blood Subs., and Immob. Biotech. 2000; 28: 147–154
- Kaplow L. S. Cytochemistry of leukocyte alkaline phosphatase. Use of complex naphthol as phosphates in azo dye couple technic. Am. J. Clin. Pathol. 1963; 39: 439–449
- Lowry O. H. Micromethods for the assay of enzyme II specific procdures. Alkaline phosphatase. Method. Enzymol. 1955; 4: 371–372
- Lynch M. P., Stein J. L., Stein G. S., Lian J. B. The influence of type I collagen on the development and maintenance of the osteoblast phenotype in primary and passaged rat calvarial osteoblast: modification of expression of genes supporting cell growth, adhesion, and extracellular matrix mineralization. Exp. Cell Res. 1995; 216: 35–45
- Maniatopoulos C., Sodek J., Melcher A. H. Bone formation in vitro by stromal cells obtained from bone marrow of young adult rats. Cell Tissue Res. 1988; 254: 317–330
- Mardon H. J., Bee J., Von Der Mark K., Owen M. E. Development of osteogenic tissue in diffusion chamber from early precursor cells in bone marrow of adult rats. Cell Tissue Res. 1987; 250: 157–165
- McGee‐Russel S. M. Histochemical methods for calcium. J. Histochem. Cytochem. 1958; 6: 22–42
- Mizuno M., Fujisawa R., Kuboki Y. Type I collagen‐induced osteoblastic differentiation of bone‐marrow cells mediated by collagen‐α2β1 integrin interaction. J. Cell Physiol. 2000; 184: 207–213
- Mundy G. R. Osteoblasts, bone formation and mineralization. Bone Remodeling and its Disorders, I. Fogelman. Martin Dunitz, London 1998; Vol. 3: 17–38, Ch. 3
- Owen M. Lineage of osteogenic cells and their relationship to the stromal system. Bone and Mineral, W. A. Peck. Elsevier, Amsterdam 1985; Vol. 3: 1–25
- Weiss L. The hemapoietic microenvironment of the bone marrow: an ultrastructural study of the stroma in rats. Anat. Rec. 1976; 189: 161–184