Abstract
α‐Galactosidase (α‐D‐galactoside galactohydrolase, EC 3.2.1.22) from watermelon was covalently immobilized on chitin. The immobilized α‐galactosidase exhibited an activity of 0.61 U per g of carrier and an activity yield of 67%. The properties of free and immobilized α‐galactosidase were also searched and compared. The results showed that, optimum conditions for activity were not affected by immobilization. The optimum pH and temperature for free and immobilized enzyme found as pH 6.0 and 65°C, respectively. Compared with the free enzyme, the temperature and pH stabilities of the immobilized enzyme were similar. Both the enzymes were stable between pH 2–10 and below 50°C. The Km values for free and immobilized enzyme were determined using p‐nitrophenyl‐α‐D‐galactopyranoside (PNPG) and raffinose as substrates. Operational stability of the immobilized enzyme was investigated by using both substrates. The operational half‐life (t 1/2) was calculated as 34 h for PNPG and 28 h for raffinose. The immobilized α‐galactosidase was also utilized in the hydrolysis of raffinose. The immobilization procedure on chitin was cheap and also easy to carry out, and the immobilized enzyme had good properties that the potential for practical application is considerable.
Introduction
Immobilization of enzymes on inert, insoluble materials is currently a very active area of research because of their wide range of applications. Immobilized enzymes have been shown to be reusable and more stable than soluble ones that are desired characteristics for enzymes in commercial use. Immobilizing enzymes on solid supports allow the reuse of catalytic activity, permits better control reactions and the design of bioreactors (Siso et al., [Citation1997]; Vaillant et al., [Citation2000]).
Chitin [(1→4)‐2‐acetamido‐2‐deoxy‐β‐D‐glucan] is the most abundant naturally occuring aminopolysaccharide, found in the shell of crustacean, the cuticles of insects and the cell walls of fungi (Illanes, [Citation1994]; Ilyina et al., [Citation1995]). It is an interesting material to produce immobilized enzyme biocatalysts for industrial processes. Utilization of chitin as solid support for enzyme immobilization is a result of their biological activity, biocompability, biodegradability in combination with its low toxicity and cost (Cho et al., [Citation1999]; Ilyina et al., [Citation2000]).
Recent advances in enzymes that catalyze hydrolysis of glycoside bonds have important roles due to their extensive industrial, therapeutic and biochemical applications (Kotwal et al., [Citation1999]). α‐Galactosidase catalyzes hydrolysis of simple and complex oligosaccharides and polysaccharides containing α‐1,6 linked terminal galactosyl group, which are widely distributed among plants (Mansour and Khalil, [Citation1998]; Pressey, [Citation1984]), humans (Beier et al., [Citation1990]; Bishop and Desnick, [Citation1981]) and microorganisms (Kotwal et al., [Citation1999]; Scigelova and Crout, [Citation2000]). This enzyme is of particular interest in view of its applications especially in food processing, the sugar industry, medicine, enzymatic synthesis and structural analysis. It is used in the hydrolysis of raffinose which inhibits crystallization of sucrose from sugar beet molasses (Porter et al., [Citation1992]), in the hydrolysis of galactooligosaccharides present in soybean milk that cause intestinal discomfort and flatulence (Mansour and Khalil, [Citation1998]). Studies on this enzyme are also of interest in medicine as its deficiency leads to Fabry disease (Kase et al., [Citation2000]). It is also investigated in blood group specificity (Lenny et al., [Citation1994]) and is required for ingestion of seed proteins by monogastric animals (Slominski, [Citation1994]).
In this work, α‐galactosidase from watermelon was covalently attached to 1,1′‐carbonyldiimidazole(CDI) activated chitin. We characterized this enzyme in both free and immobilized forms by determining pH, temperature and thermal profiles, storage and operational stabilities. Meanwhile, kinetic parameters (Km, Ea) and reusability were also measured with p‐nitrophenyl‐α‐D‐galactopyranoside (PNPG) and raffinose as substrate. Finally, the practical applicability of immobilized α‐galactosidase for raffinose hydrolysis was also tested in batch and continuous processes.
Materials and Methods
Materials
p‐Nitrophenyl‐α‐D‐galactopyranoside(PNPG), 1,1′‐carbonyldiimidazole (CDI), 1‐ethyl‐3(3‐dimethylaminopropyl)carbodiimide(EDC), raffinose and chitin were purchased from Sigma Chemical Co. (St. Louis, USA). Watermelons (Citrullus vulgaris) were obtained from local markets. All other chemicals and reagents were obtained from local suppliers and were analytical grade or better.
Extraction of α‐Galactosidase
The method previously described (Önal and Telefoncu, [Citation1996]) was followed in a modified form. After the seeds and peel had been removed, the watermelon fruit was cut into small pieces and homogenized. The homogenate was decanted through two layers of cheese‐cloth and centrifuged at 10 000 rpm for15 min. The pH of the resulting supernatant was adjusted to pH 6.0 and subjected to 85% ammonium sulphate precipitation. The mixture was allowed to stand overnight with continuous stirring. The precipitate was collected by centrifugation at 10 000 rpm for 30 min, then dissolved in distilled water and dialysed overnight. In order to remove the insoluble matter, dialysate was centrifuged at 4 000 rpm for 5 min and then subjected to 25–85% ammonium sulphate precipitation overnight with continuous stirring. The resultant precipitate was dissolved in and dialysed against bidistilled water. The obtained α‐galactosidase preparation (3.46 Unit/ml and 3.6 mg protein/ml) was kept at − 20°C until immobilization.
All steps for the preparation of the partially purified α‐galactosidase were carried out at 4°C.
Immobilization of α‐Galactosidase
Chitin (1 g) was first washed several times with distilled water and then washed sequentially with 25 ml of water, dioxane:water (3:7), dioxane:water (7:3) and suspended in 15 ml of anhydrous dioxane. 0.167 g 1,1′‐carbonyldiimidazole(CDI) was added to the suspension and then was agitated gently (200 rpm) at room temperature for 3 hours. In order to remove liberated imidazole, the support was washed with anhydrous dioxane and then used for enzyme immobilization. The CDI activated chitin and watermelon α‐galactosidase preparation (specific activity: 0.96 U/mg) was added to 10 ml of 50 mM sodium citrate buffer (pH 6.0) with gentle shaking at 4°C overnight. The immobilized enzyme conjugate was separated by filtration and then in order to remove the unbound proteins it was washed with 25 ml of distilled water, 12.5 ml of 0.5 M NaCl and 25 ml of distilled water, respectively. The washing solutions were collected and used for protein determination.
Assay of α‐Galactosidase Activity
The α‐galactosidase activity was assayed in a reaction mixture consisting of 0.25 ml of 2.0 mM PNPG, 0.5 ml of 50 mM sodium citrate buffer (pH 6.0) and 0.25 ml enzyme solution (3.46 U/ml). After incubation for 30 min at 37°C, the reaction was stopped by the addition of 0.2 M sodium borate buffer (pH 9.8). The activity of immobilized enzyme was determined using 0.05–0.1 g immobilized enzyme (0.61 U/g) in a total volume of 1 ml reaction mixture indicated above. The reaction was stopped with borate buffer, then the mixture was centrifuged and assayed. The quantity of liberated p‐nitrophenol was measured at 400 nm. One unit of enzyme activity was defined as the amount of enzyme which released one µmol of p‐nitrophenol per min at pH 6.0 and 37°C (Itoh et al., [Citation1986]).
The α‐galactosidase activity against raffinose was determined as follows: The reaction mixture consisting of 0.1 ml of 10 mM raffinose, 0.65 ml of 50 mM citrate buffer (pH 6.0) and 0.25 ml of the free enzyme was incubated at 50°C for 60 min. In the assay of immobilized enzyme, 0.005–0.01 g immobilized enzyme was used instead of the free enzyme in the reaction mixture mentioned above. After incubation, 1 ml of mixture was added to 0.5 ml of alkaline copper reagent and the amount of galactose produced was measured by the method of Smogyi and Nelson (Smogyi, [Citation1952]).
The specific activity is expressed as units per mg of protein (U/mg).
Protein Measurement
Protein concentrations were determined by the method of Lowry et al. ([Citation1951]) by using bovine serum albumin as standard. The amount of bound protein was calculated from the difference between the amount of protein introduced into the reaction mixture and the total protein present in the filtrate and washing solutions after immobilization.
Determination of Some Properties of Free and Immobilized α‐Galactosidase
Effect of pH on Activity
The effect of pH on activity of free and immobilized α‐galactosidase was assayed in 50 mM glycine/HCl (pH 1.5–2.0), citrate (pH 2.0–6.5), phosphate (pH 7.0–8.0) and Tris/HCl (pH 8.0–9.0) buffers by using standard activity assay procedure with PNPG as substrate.
Effect of Temperature on Activity
The effect of temperature on activity of free and immobilized enzymes was studied between 4°C–95°C at the optimum pH of both enzyme preparations and then the activities were assayed as mentioned above.
Km Determination
The Michaelis constant (Km) was calculated for the free and immobilized enzyme by measurements of enzyme activity in increasing PNPG concentrations ranging from 0.05 to 2.25 mM and raffinose concentrations from 2.5 to 45 mM in 50 mM citrate buffer (pH 6.0). The same amount of free and immobilized enzyme (in terms of protein) was used in these assays.
pH Stability
The pH stabilities of the both forms of enzyme were compared in various buffers (pH 1.5–12.0). The enzyme preparations were incubated in these buffers for 6 h at 4°C and then the remaining activity was assayed under the standard assay conditions.
Thermal Stability
The effect of temperature on enzyme stability was checked in temperature range 4°C–80°C. Both enzyme preparations which has the same amount of protein in incubation mixture were incubated in citrate buffer (50 mM, pH 6.0) for 30 min at different temperatures and then cooled. The remaining activity was measured by using PNPG as substrate.
The pre‐incubation time dependent inactivation of the enzyme was also searched. The enzymes were incubated in citrate buffer (50 mM, pH 6.0) at different temperatures (37°C, 50°C and 65°C) for the desired times (between 0 to 90 min) and then their activities were assayed against PNPG.
Operational Stability
The operational stability of the immobilized enzyme was determined for PNPG and raffinose in a batch‐stirred tank reactor. The reaction was carried out for 24 h at 37°C for PNPG and for 50 h at 50°C for raffinose. The activity of samples taken at regular time intervals was measured. The relationship between operation time and the conversion were determined and also the half‐life of the biocatalyst was calculated.
Storage Stability
The free and immobilized enzyme were stored at 4°C in citrate buffer (50 mM, pH 6.0) and the storage stabilities of the enzyme preparations were determined by measurement of the activity of samples taken at regular time intervals and compared.
Reusability
The stability of immobilized enzyme on repeated use was examined by measuring the activity for the hydrolysis of PNPG and raffinose at 37°C and 50°C, respectively. After each activity determination the immobilized enzyme was washed with distilled water and used again.
Hydrolysis of Raffinose
A batch‐stirred tank reactor and a continuous flow‐packed bed reactor were designed for the hydrolysis of raffinose by using chitin immobilized α‐galactosidase.
10 ml of 100 mM raffinose solution prepared in 50 mM citrate buffer (pH 6.0) and 90 ml of citrate buffer were mixed in the batch reactor and then 1 g of immobilized enzyme was added. The reactor was maintained at 50°C and stirred continuously with magnetic stirrer.
As a second reactor, immobilized enzyme was poured into a glass jacket column and the jacket was maintained at 50°C throughout the experiment. 5 ml of 10 mM raffinose solution prepared in 50 mM citrate buffer (pH 6.0) and 45 ml of citrate buffer were mixed in reservoir and stirred magnetically. This feed solution was passed through the column continuously during a period of 11 h with a peristaltic pump. Samples were withdrawn after constant time of incubation and the amount of galactose was determined by the method of Smogyi‐Nelson (Smogyi, [Citation1952]).
Results and Discussion
Immobilization of α‐Galactosidase on Chitin
The watermelon α‐galactosidase was immobilized on CDI‐activated chitin by covalent coupling. To establish the simplified procedure for the preparation of the immobilized α‐galactosidase from an agricultural product, we used watermelon as an enzyme source instead of a commercial α‐galactosidase preparation. After the extraction of α‐galactosidase from watermelon, an enzyme preparation having 3.46 U/ml was obtained and used for the immobilization studies. The immobilization results were given in . A comparison of the values in clearly shows that, immobilization by covalent attachment would result in a larger amounts of active α‐galactosidase coupled to the chitin. As is seen from the table, the yield of α‐galactosidase immobilization varied significantly with the amount of loaded protein. The immobilized enzyme retained 67% of original specific activity with 96% of protein binding capacity. The activity yield of the enzyme was defined as;Prior to the present investigation, Mitsutomi et al. ([Citation1985]) were immobilized Pycnoporus cinnabarinus α‐galactosidase on chitin with the activity yield of 27.5% which was lower than our result. This could be attributed to two kinds of effect: the method used in immobilization and difference in enzyme source.
Table 1. The Immobilization Results of α‐Galactosidase from Watermelon
Properties of the Immobilized α‐Galactosidase
We attempt to examine the properties of the immobilized α‐galactosidase and to compare them with those of the free enzyme.
The pH profile on the activity of the immobilized enzyme compared to the free enzyme is presented in . As is seen from the figure, the optimum pH of the immobilized enzyme was pH 6.0, with no shift from the pH optima for the free enzyme. Similar results have been reported by Ohtakara and Mitsutomi ([Citation1987]) for α‐galactosidase immobilized on chitosan beads. This is an expected result, because chitin is not a charged carrier and also there is no any acidic or basic product as a result of enzymatic reaction (Sun et al., [Citation1998]; Telefoncu, [Citation1983]).
The effect of pH on the stability of the enzyme was determined in various buffers. As shown in , in the range of pH 2.0–11.0 the immobilized enzyme was very stable and more than 80% of the original activity was recovered. Different pH‐activity and pH‐stability profiles of immobilized α‐galactosidase have been obtained depending on a support chosen and on an immobilization method applied (Mitsutomi et al., [Citation1985]; Ohtakara and Mitsutomi, [Citation1987]).
The relative activity expressed in percentage of the maximum activity is presented in as a function of temperature. The maximum activity was observed at 65°C for both forms of enzyme. The Arrhenius plots (log activity versus 1/T) were linear and the calculated values of activation energy (Ea) obtained through the curve were equal to 14 kJ/mol and 20 kJ/mol for the free and immobilized enzyme, respectively. The immobilized enzyme has a little higher activation energy than that of the free enzyme. This increase in Ea for the immobilized enzyme could be attributed to steric hinderances or diffusional limitation. Similar results were reported previously (Krajewska et al., [Citation1990]).
Figure 3. The effect of temperature on α‐galactosidase activity: (•); free enzyme, (□); immobilized enzyme.
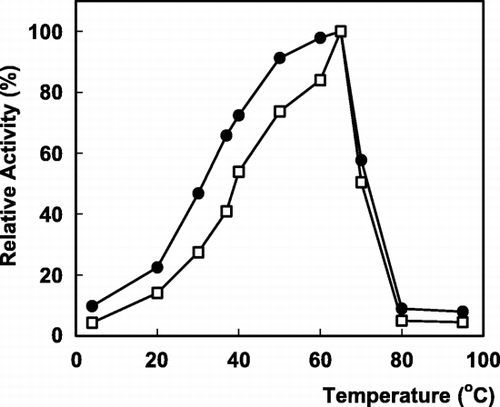
The effect of temperature on the stability of the enzyme is shown in . The immobilized enzyme was as thermostable as the free enzyme. Both the enzymes completely retained nearly about 90% of their original activity below 50°C, but they were destroyed their activities quickly above 65°C.
Thermal stability of α‐galactosidase dependent on the preincubation time was presented in . It shows that the immobilized enzyme was much more stable than free enzyme. At 37°C, the activities of the both forms of enzyme slowly decreased; the free enzyme retained 78% of activity after 90 min and the immobilized enzyme retained 94% of activity under identical conditions. At 65°C, over a period of the same time, the residual activity of the native enzyme was 23% whereas that of the immobilized enzyme was 43%. We could see the thermal stability of the two kinds of enzyme forms has the same decreasing trend at high temperature according to the graph. As the initial stage the activity quickly decreased but then decreased slowly with the time. This might be explained by the enzyme's having some heat‐resisting component. The thermal stability of immobilized enzyme is generally very important for its industrial application. In several studies of α‐galactosidases from different sources, the thermal stability was also increased by immobilization (Mitsutomi et al., [Citation1985]).
Figure 5. Thermal stability of α‐galactosidase dependent on pre‐incubation time: (a); free enzyme, (b); immobilized enzyme [•; 37°C, ▴; 50°C, ▪; 65°C].
![Figure 5. Thermal stability of α‐galactosidase dependent on pre‐incubation time: (a); free enzyme, (b); immobilized enzyme [•; 37°C, ▴; 50°C, ▪; 65°C].](/cms/asset/d94739e0-622e-4c4a-a297-1d35f4495901/ianb19_a_11116863_uf0005_b.gif)
The enzyme obeyed simple Michaelis‐Menten kinetics toward both PNPG and raffinose as demonstrated by straight lines obtained for double reciprocal plots. Lineweaver‐Burk plots for the free and immobilized enzymes were drawn for both substrates and the Km values were calculated from the graphs. The Km of free and immobilized enzyme were 0.298 mM and 0.426 mM, respectively for PNPG. These close Km values show that the immobilization process had an insignificiant effect on the α‐galactosidase affinity for PNPG. The Km of free and immobilized enzyme for raffinose were calculated as 12.6 mM and 42.1 mM, respectively. The Km value is affected by many sorts of complicated factors. Such increase in the Km of the immobilized enzyme may be a lower accessibility of the substrate to the active site of the immobilized enzyme or the possible changes in the enzyme‐substrate interaction (Sun et al., [Citation1998]).
The operational stability of α‐galactosidase with PNPG and raffinose is very good. Figure shows that the immobilized enzyme lost about 10% of its activity within 6 h and then slowly decreased its activity to 66% within 24 h. When raffinose used as substrate, the immobilized enzyme lost 45% of its activity within 50 h (). The operational half life (t1/2) of the enzyme was calculated by using the formula given as;where t is the operation time, kD is decay constant, Ao and A are the enzymatic activities at the beginning and at t time. The half life of chitin bound α‐galactosidase was determined as 34 h for PNPG and 28 h for raffinose.
Figure 6. Operational stability of immobilized α‐galactosidase: (a); PNPG, (b); raffinose as substrate.
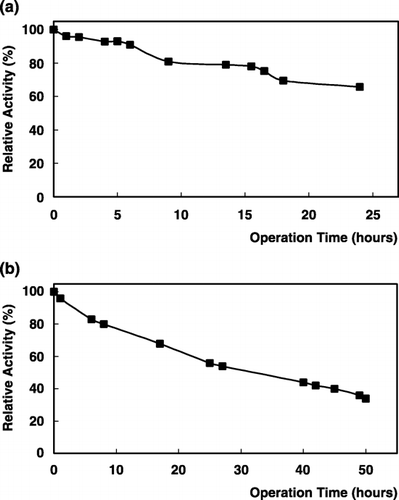
Both the free and immobilized enzyme were stored at 4°C under the same conditions mentioned above. The enzymes retained 97% of their original activities about 30 days, but there after the activities were slowly decreased. The immobilized enzyme lost about 41% of its activity over a period of 7 months, whereas the free enzyme lost about 64% of its original activity over the same period of time. Various reports confirm that the storage stability of immobilized enzyme varies depending on the immobilization method applied and storage conditions (Krajewska et al., [Citation1990]; Vaillant et al., [Citation2000]).
The stability of immobilized α‐galactosidase on repeated use was examined by measuring the activity for the hydrolysis of PNPG and raffinose (). As is seen from the figures, the immobilized enzyme retained 30% of its initial activity after 33 cycles of reuse with PNPG (), and 70% of its initial activity after 19 cycles of reuse with raffinose (). Such reusability is advantageous for the continuous use of this enzyme in biotechnological applications (Mitsutomi et al., [Citation1985]; Ohtakara and Mitsutomi, [Citation1987]).
Laboratory‐Scale Application of Immobilized α‐Galactosidase to Hyrolysis of Raffinose
α‐Galactosidase immobilized on chitin was utilized in the hydrolysis of raffinose. The conversion of raffinose hydrolysis is defined as the proportion of substrate consumed at time t [X = (So − St)/So], where So and St are the concentrations of raffinose in the reservoir at time to and t, respectively and the product concentration corresponds to the difference between So and St (So − St). Thus the variables X and t may be plotted on a conversion curve. Comparison of conversion efficiencies of this immobilized enzyme in a batch‐stirred tank reactor and continuous flow‐packed bed reactor that the packed‐bed reactor has an higher efficiency. As is seen from , the conversion of the raffinose in a batch system was 5.5% within 50 h (). but in a packed‐bed reactor the conversion was 7.5% within 7 h (). This indicated that both the reactors gave similar conversion results but batch reactor requires a reaction time 7 times higher than for the packed‐bed reactor to reach the same productivity. The results related to the hyrolysis of raffinose with α‐galactosidase described that the column reactors are much favourable than batch systems (Reynolds, [Citation1974]; Thanankul et al., [Citation1976]) and α‐galactosidase from watermelon would have a promising application in the hydrolysis of raffinose in molasses.
Conclusion
This study shows that α‐galactosidase prepared from watermelon was immobilized successfully on CDI activated chitin. The system offers various advantages: Chitin is relatively inexpensive and inert material and also easy to get. The immobilization procedure is very simple and easy to carry out. The properties of the immobilized enzyme offer potential for use in production processes. The obtained enzyme has a comparatively high enzymatic activity. The activity, the thermal stability and catalytic characteristics of immobilized enzyme are important factors when selecting an appropriate enzymatic system for applications. Chitin immobilized α‐galactosidase with excellent storage, thermal and operational stabilities and reusability demonstrates the potential of the immobilized enzyme for practical application. A laboratory‐scale application of immobilized enzyme to the hydrolysis of raffinose was compared by using two reactors. The packed‐bed reactor is much favourable than batch system.
Acknowledgment
The authors are grateful for the financial support provided by the Ege University Research Foundation under project FEN 95/025.
References
- Beier E. M., Shono N., Kozlora I., Viedershain G. Y. Relationship of the multiple forms of human α‐galactosidase and α‐D‐fructosidase in the normal and Fabry's disease. Biochim. Biophys. Acta 1990; 1038: 386–389
- Bishop D. F., Desnick R. J. Affinity purification of α‐galactosidase A from human spleen, placenta and plasma with elimination of pyrogen comtamination. J. Biol. Chem. 1981; 256(3)1307–1316
- Cho Y.‐W., Cho Y.‐N., Chung S.‐H., Yoo G., Ko S.‐W. Water‐soluble chitin as a wound healing accelerator. Biomaterials 1999; 20: 2139–2145
- Illanes A. Chitin as a matrix for enzyme immobilization. Advances in Bioprocess Engineering, E. Galindo, O. T. Ramirez. Kluwer Academic Publishers. 1994; 461–466
- Ilyina A. V., Tikhonov V. E., Varlamov V. P., Radigina L. A., Tatarinova N. Y., Yamskov I. A. Preparation of affinity sorbents and isolation of individual chitinases from a crude supernatant produced by Streptomyces kurssanovii by a one step affinity‐chromatographic system. Biotechnol. Appl. Biochem. 1995; 21: 139–148
- Ilyina A., Tikhonov V. E., Albulov A. I., Varlamov V. P. Enzymatic preparation of acid free water soluble chitosan. Process Biochem. 2000; 35: 563–568
- Itoh I., Uda Y., Nakagawa H. Purification and characterization of α‐galactosidase from watermelon. J. Biochem. 1986; 99: 243–250
- Kase R., Bierfreund U., Klein A., Kolter T., Utsutomi K., Itoh K., Sandhoff K., Sakuraba H. Characterization of two α‐galactosidase mutants (Q279E and R301Q) found in an a typical variant of Fabry disease. Biochim. Biophys. Acta 2000; 1501: 227–235
- Kotwal S. M., Gote M. M., Khan M. I., Khire J. M. Production, purification and characterization of a constitutive intracellular α‐galactosidase from the thermophilic fungus Humicola sp. J. Ind. Microbiol. Biotechnol. 1999; 23: 661–667
- Krajewska B., Leszko M., Zaborska W. Urease immobilized on chitosan membrane: preparation and properties. J. Chem. Technol. Biotechnol. 1990; 48: 337–350
- Lenny L. L., Hurst R., Goldstein J., Galbraith R. A. Transfusions to group subjects of 2 units of red cells enzymatically converted from group B to group O. Transfusion 1994; 34: 209–214
- Lowry O. H., Rosebrough N. J., Farr A. L., Randall R. J. Protein measurement with the Folin phenol reagent. J. Biol. Chem. 1951; 193: 265–275
- Mansour E. H., Khalil A. H. Reduction of raffinose oligosaccharides in chick‐pea (Cicer arientinum) flour by crude extracellular fungal α‐galactosidase. J. Sci. Food Agric. 1998; 78: 175–181
- Mitsutomi M., Uchida Y., Ohtakara A. Immobilization of thermostable α‐galactosidase from Pycnoporus cinnabarinus on chitin and some properties of the immobilized enzyme. J. Ferment. Technol. 1985; 63(4)325–329
- Ohtakara A., Mitsutomi M. Immobilization of thermostable α‐galactosidase from Pycnoporus cinnabarinus on chitosan beads and its application to the hydrolysis of raffinose in beet sugar molasses. J. Ferment. Tech. 1987; 65(4)493–498
- Önal S., Telefoncu A. Purification of α‐D‐galactosidase from watermelon (Citrullus vulgaris) by affinity‐ultrafiltration. J. Fac. Sci., Ege Univ. 1996; 19(2)75–88
- Porter J. E., Sarikaya A., Herrmann K. M., Ladisch M. R. Effect of pH on subunit association protection of soybean α‐galactosidase. Enzyme Microb. Technol. 1992; 14: 609–614
- Pressey R. Tomato α‐galactosidases: conversion of human type B erytrocytes to type O. Phytochemicals 1984; 23(1)55–58
- Reynolds J. H. The uses of precipitated nylon as an enzyme support: an α‐galactosidase reactor. Immobilized Enzymes in Food and Microbial Processes, A. C. Olson, L. Cooney. Plenum Press, New York 1974; 63–70
- Scigelova M., Crout D. H.G. Purification of α‐galactosidase from Aspergillus niger for application in the synthesis of complex oligosaccharides. J. Mol. Catal. B: Enzym. 2000; 8: 175–181
- Siso M. I.G., Lang E., Gomez B. J., Becerra M., Espinar F. O., Mendez J. B. Enzyme encapsulation on chitosan microbeads. Process Biochem. 1997; 32: 211–216
- Slominski B. A. Hydrolysis of galactooligosaccharides by commercial preparations of α‐galactosidase and β‐fructofuranosidase: potential for use as dietary additives. J. Sci. Food Agric. 1994; 65: 323–330
- Smogyi M. Notes on sugar determination. J. Biol. Chem. 1952; 95: 19–23
- Sun S., Xuyuan L. S., Nu S., You X. Immobilization and characterization of β‐galactosidase from the plant gram chicken bean (Cicer arietinum). Evolution of its enzymatic actions in the hydrolysis of lactose. J. Agric. Food Chem. 1998; 47: 819–823
- Telefoncu A. Preparation and properties of Trypsin chemically attached to EEDQ activated styrene‐methacrylic acid copolymers. Biotechnol. Bioeng. 1983; 25: 713–724
- Thanankul D., Tanaka M., Chichester C. O., Lee J. Degradation of raffinose and stachyose in soybean milk by α‐galactosidase from Mortierella vinacea. J. Food Sci. 1976; 41: 173–175
- Vaillant F., Millan A., Millan P., Dornier M., Decloux M., Reynes M. Co‐immobilized pectinlyase and endocellulase on chitin and nylon supports. Process Biochem. 2000; 35: 989–996