Abstract
Arginase and urease enzymes were immobilized on the surface of pH electrode by using gelatin membrane which is then cross‐linked with glutaraldehyde. Sensor response was maximum when 2.5 mM, Tris‐HCl buffer (pH 8.5) was used at 25°C. The biosensor response depends linearly on arginine concentration between 0.025–0.310 mM with response time 10 min. Furthermore, application of the system for the arginine detection in serum samples was also tested.
Introduction
L‐Arginine is a naturally‐occurring basic amino acid which participates in many important biochemical reactions associated with the normal physiology of the organism. Found in most proteins we consume in our daily diet, L‐arginine can either be metabolized to support glucose synthesis or catabolized to produce energy, depending upon the needs of the body. This amino acid is now known to be the immediate precursor of the endogenous vasodilator substance found in the arterial blood vessels termed “endothelium‐derived relaxing factor”(EDRF). It has now been determined that EDRF is chemically identical to nitric oxide (NO), a free radical gas which was previously known to be present in macrophages and thought to mediate a number of this cell’s cytotoxic actions. Since this finding that NO is identical to EDRF, NO has become the attention of numerous investigations in which it has been determined that this endogenous compound plays critical roles in many diverse physiological processes. It should be emphasized from the outset that NO is not the same entity as the general anesthetic agent nitrous oxide (N2O) also referred to as “laughing gas,” since confusion between the two is common in the lay literature In addition to its important role as a component of most proteins, L‐arginine also undergoes numerous metabolic fates. While much interest has been directed toward its conversion to NO, urea, L‐citrulline, L‐ornithine, L‐proline, L‐glutamate, and polyamines such as putrescine are also formed from L‐arginine (Guoyano and Sidney, [Citation1998]; Maher, [Citation1994]). Moreover, arginine has been administered in many studies pharmacologically to animals and therapeutically to humans. In addition, arginine is being sold in many health food stores where consumers buy these preparations because of a variety of reputed, yet unproven, health benefits (Signorello et al., [Citation2001]; Szende et al., [Citation2001]; Trabelsi et al., [Citation1994]). Since this compound is necessary for protein synthesis and a host of other important biochemical reactions, it is important to detect L‐arginine in physiogical fluids. Biosensors are useful tools for the medical and clinical analysis and enable us to make practical analysis without time consuming (Pearson, [Citation2000]; Zhang et al., [Citation2000]). The aim of the present study is to develop a relatively inexpensive and simple procedure for the arginine determination.
Arginase and urease enzymes were immobilized directly on pH electrode by copolymerization of gelatin, using the bifunctional agent glutaraldehyde. The enzymatic reaction belong to arginase and urease couple is given below;
As the products of the urea hydrolysis are alkaline, an increase of urea concentration related with arginine substrate in the analyzed solution causes an increase of pH inside the biomembrane of the biosensor. The changes could be easily detected by pH electrode.
Material and Methods
Arginase (EC 3.5.3.1), Urease (EC 3.5.1.5), 225 bloom calf skin gelatin and glutaraldehyde were obtained from Sigma Chem. Co. (St. Louis, U.S.A). All other chemicals were purchased from E. Merck (Darmstadt, FRG). Glass electrode (Mettler Toledo Process Analytical Inc., NJ, U.S.A) was used as a transducer.
The blood serum samples were kindly provided by Medical Social Laboratory of Ege University. The samples were diluted 10 times with working buffer solution.
Electrode Preparation
Arginase(23.5 U), urease(270 U) and gelatin(50 mg) were mixed in 1 ml of 50 mM phosphate buffer(pH 7.5) at 38°C in water bath. 0.1 ml of the mixed solution was placed on the surface of pH electrode by dip coating. Then, allowed to dry at 4°C 1 hour. Finally, it was immersed in 2.5 % glutaraldehyde in 50 mM phosphate buffer (pH 7.5) for 5 min and subsequently washed in a stream of distilled water (Ertaş et al., [Citation2000]). The enzyme electrode contained approximately 2.35 U arginase and 27 U urease activity.
Measurement of Biosensor Response
Measurements were carried out at 25°C and varying arginine concentration in steady‐state condition. 2.5 mM of Tris‐HCl buffer was used as a working buffer solution. A change in proton concentration produced by the enzymatic reactions at the glass‐electrode surface and the corresponding change in the output voltage is considered to be a measure of the enzymatic membrane activity. It was followed by pH‐meter.
Results and Discussion
Some preliminary experiments were performed to establish the optimum conditions of pH and buffer concentration to obtain maximum sensor response. The arginine sensor response can be changed substantially by varying the buffer concentration and pH. Maximum sensor response was obtained at 2.5 mM Tris‐HCl buffer (pH 8.5), (). The response was also diminished with increase in buffer concentration which is common property of the potentiometric systems (Ru‐Qin et al., [Citation2000]). These results are also well agree with the previous works (Koncki et al., [Citation1996]). Furthermore, the effect of varying assay temperature (20–45°C) was also examined and 25°C was found to be optimum (). All further measurements were done under these experimental conditions.
Figure 1. The effect of pH on the biosensor response (Tris‐HCl; pH 7.0–8.5, borate; pH 9.0–9.5, arginine conc.; 0.25 mM, 25°C).
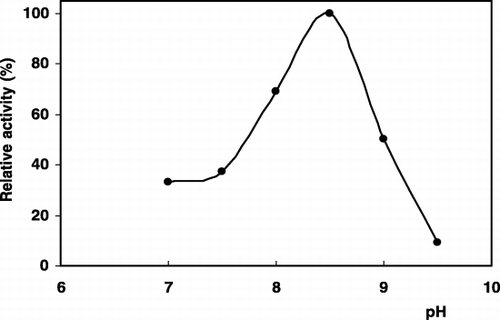
The calibration curves for arginine was obtained in the range of 0.025–0.310 mM. In the high concentration regions, the sensor response was remained steady. The changes in mV values were plotted versus cologarithm of arginine concentration. The calibration graph is defined by the equation y = − 4.449x + 7.168, (R2 = 0.995).
The biosensor was tested for its areproducibility on fourteen standard solutions containing equal amounts of arginine (0.125 mM) and standard deviation was calculated as ± 0.008. On the other hand, it is possible to make approximately 20 measurement with the biosensor during a day. After that, a decrease was observed on the activity. Thus, in order to get reproducible results, freshly prepared enzyme electrode was used each day.
The application of the system for the arginine detection in blood serum samples was also carried out by standard addition method. Before these experiments, urease electrode without arginase enzyme but having the same composition (urease; 270 U and gelatin; 50 mg in 1 ml of 50 mM phosphate buffer, pH 7.5) was prepared and used to test the urea level of the serum samples which is interfere the response of biosensor system based on arginase‐urease enzyme couple. The standard graph for urea was plotted in the range of 0.025–0.310 mM. The changes in mV values were plotted versus cologarithm of urea concentration. The lineer graph is defined by the equation y = − 2.997x + 5.215, (R2 = 0.995).
Arginase‐urease biosensor give the response towards to both urea and arginine. If the serum sample was analysed by both arginase‐urease and urease biosensor, the differences of the signals obtained by these system can give the arginine level. For the measurement, instead of the substrate the diluted sample was added to the reaction cell and change in the output voltage is recorded and concentrations were found from the calibration curves. All the results are summarized in . As is shown in , no signal was observed by urease electrode in serum sample. It means that urea level is lower than the detection limit of the sensor, thus the observed signal caused from the arginine content of the blood serum. It is also clear that, there is no difference in the signal obtained from arginine standard solution prepared in working buffer and in serum samples. It is an evidence that there is no matrix effect to the system.
Table 1. Arginine Detection in Blood Serum Samples
In this work, both arginase and urease were immobilized in gelatin to form a bioactive membrane layer which was deposited on the sensitive area of the pH‐electrode. The immobilization method is easier and faster than standard immobilization techniques namely cross linking with glutaraldehyde on previously silanised surfaces, carbodiimide method, avidin‐biotin linkage, etc. (Lobnik et al., [Citation1998]; Padeste et al., [Citation2000]) The use of this sensor displays great advantages for its simplicity. Furthermore, this sensor is portable and no special skill are required to use it.
References
- Ertaş N., Timur S., Akyılmaz E., Dinçkaya E. Specific determination of hydrogen peroxide with a catalase biosensor on mercury thin film electrode. Tr. J. Chem. 2000; 24: 95–99
- Guoyano W., Sidney M. M. Arginine metabolism:nitric oxide and beyond. Biochem. J. 1998; 336: 1–17
- Koncki R., Walcerz I., Ruckruh F., Glab S. Bienzymatic potentiometric electrodes for creatine and L‐arginine determination. Anal. Chim. Acta. 1996; 333: 215–222
- Lobnik A., Oehme I., Murkovic I., Wolfbeis O. S. pH optical sensors based on sol‐gels: chemical doping versus covalent immobilization. Anal. Chim. Acta 1998; 367: 159–165
- Maher T. Safety concerns regarding supplemental amino acids. Food Components to Enhance Performance, B. M. Marriott. National Academy Press, Washington, DC 1994; 455–460
- Pearson J. E. Analytical aspects of biosensors. Ann. Clin. Biochem. 2000; 37: 119–145
- Padeste C., Grubelnik A., Tiefenauer L. Ferrocene‐avidin conjugates for bioelectrochemical applications. Biosens. Bioelectron. 2000; 15: 431–438
- Ru‐Qin Y., Zong‐Rang Z., Guo‐Li S. Potentiometric sensors:aspects of the recent development. Sensors Actuators B 2000; 65: 150–153
- Signorello M. G., Givovine M., Pascale R., Cataldi L., Bordone C., Benatti U., Leoncini G. Impaired L‐arginine uptake in platelets from type‐2 diabetic patients. Biotechnol. Appl. Biochem. 2001; 34: 19–23
- Szende B., Tyihak E., Trezl L. Role of arginine and its methylated derivatives in cancer biology and treatment. Cancer Cell Int. 2001; 1: 1–5
- Trabelsi F., Helie R., Bergeron R., Lavoie J. M. Effect of inhibition of gluconeogenesis on arginine‐induced insulin secretion. Physiol. Behev. 1994; 57: 797–802
- Zhang S., Wright G., Yang Y. Materials and techniques for electrochemical biosensors design and constructions. Biosens. Bioelectron. 2000; 15: 273–282