Abstract
The purpose of this study was to determine the pharmacodynamics of polyethylene glycol (PEG) conjugation on stroma-free bovine hemoglobin, hemorrhage shock, and exchange transfusion rat models were used. In both rat models, blood pressure increased in 6% PEG-bHb treated animals was significantly higher than dextran 70, even than whole blood (isovolume of hemorrhage) transfusion. Six percent PEG-BHb could ameliorate the micro-circulation of the experimental animals markedly, including reducing the blood viscosity and improving the blood flow. This effect of PEG-bHb was superior to autogenous blood which only recovered 50% blood flow, and there's no effect on blood flow when used isovolumic dextran 70. Tissue oxygenations of rats were evaluated by the oxygen dependent quenching of phosphorescence using an Oxyspot phosphorimeter, and the results showed that capability of oxygen-delivery of PEG-bHb was close to autogenous blood and superior to dextran 70. Based on these effects, the survival rates of animals treated with PEG-bHb were close to that of the whole blood transfusion. And data suggested that half of the hemorrhage transfusion is the reasonable therapeutic dosage. In conclusion, PEG-bHb is an effective blood substitute with powerful tissue oxygenation and blood volume expansion.
Introduction
With the realization that plasma expansion alone was not adequate replacement for blood in the treatment of hemorrhage, the limitations of liquid RBCs such as the source and storage, requirement for cross-matching and transmission of diseases, seeking effective blood substitutes has become an urgent task since the World War II (Ketcham and Cairns, Citation[[1998]]). Bovine erythrocytes are one source of hemoglobin that has shown potential in the development of hemoglobin based oxygen carriers (HBOC). Bovine Hb is cheaper than human Hb, doesn’t require 2,3-diphosphoglycerate to lower its oxygen affinity, and can enhance delivery of oxygen at a lower pH because of Bohr effect (Benesch et al., Citation[[1972]]). However, unmodified stroma-free hemoglobin are rapidly cleared from the body and can cause renal toxicity (Center for Biologics Evaluation and Research, Citation[[1991]]). Structural modification of hemoglobin can attenuate some of these unfavorable attributes while preserving its oxygen carrying ability (Squires, Citation[[2002]]). Proteins pegylation, which is conjugated polyethylene glycol (PEG) to proteins, has been demonstrated to reduce renal filtration dramatically, prolong blood circulation, and reduce any potential immune or allergic reactions (Veronese, Citation[[2001]]). PEG-bHb is developed for intravenous use as an oxygen-carrying solution by Kaizheng Biotech, and its pharmacodynamics will be evaluated by shock resuscitation and exchange transfusion rat models in this contribution.
Materials and Methods
Animals
Sprague-Dawley rats (mixed gender, 180–220 g), provided by Experimental Animals’ Center of the First Military Medical University of China (Certification No.: 2000A059).
Test Solutions
PEG-bHb (6% bovine hemoglobin wt/v solution, methemoglobin <5%, endotoxin <1.0 EU/mL, osmolality 330 ± 30 mOsm, pH 7.4 ± 0.2) was formulated in buffer consisting of 150 mmol/L NaCl, 5 mmol/L NaHCO3, 4 mmol/L Na2HPO4, and 1 mmol/L NaH2PO4 and stored at −35°C. The dextran 70 was purchased from Sigma.
Animal Models
In both models, rats were anesthetized using 13.3% urethane and 0.5% chloralose (0.6 mL/kg, i.p.), and placed on a heating pad with their body temperature maintained at approximately 37°C. In hemorrhagic shock model, MAP of rats was maintained at 40 mmHg for 1 h by blood controlled withdrawal, then transfused with PEG-bHb and other fluids. In the exchange model, rats received a 50% exchange transfusion of their estimated blood volume (weight of animal (kg) × 60 mL/kg × 50%).
Rats of two models were randomized into Seven treatment groups respectively ().
Table 1. The treatment groups of rats
Pharmacodynamics Measurement
Mean arterial blood pressure (MAP) and heart rate (HR) were monitored every 10 min, beginning 2 h before surgery and for 3 h after transfusion. Blood samples were withdrawn to test Cl−, pH, PCO2, PO2, and viscosity. Micro-circulation of rat spinotrapezius muscle was observed (Gray, Citation[[1973]]), including the velocity and volumetric flow rate and the diameter of the microvessels.
Surface tissue oxygen tension was measured by the oxygen dependent queching of phosphorescence using an Oxyspot™ phosphorimeter (Medical Systems Corp, Greenvale, NY). A dosage of 2 mg/100 g b.wt of phosphorescent probe was administered via vein (Conover et al., Citation[[1999]]). Data were recorded and analyzed 60 min before and after shock and 0, 30, 60, 120, 180 min after resustation.
Statistical Analysis
The differences among treatment groups were assessed by one-way ANOVA. Multiple comparisons, when significant differences existed, were determined by least significant differences techniques. Statistical significance was defined as p < 0.05 to reject a null hypothesis. Statistical analysis was conducted with SPSS 10.0 software program.
Results
Survival
PEG-bHb and whole blood-treated groups showed significantly higher survival rate ( p < 0.05) than the no fluid group and dextran group at 24 h in the exchange model. Resuscitation effects were best by half volume hemorrhage PEG-bHb infusion in shock (S4) and exchange (E4) models in rats and infusion isovolume and 1.5-fold volume hemorrhage PEG-bHb showed lower survival rate ( and ).
Cardiovascular Monitoring
Basal conscious MAP and HR were similar among all groups in both models examined and were consistent with the normal values of the rat. MAP decreased with volume controlled hemorrhage in model rats, then increased until infusion of treatment fluids. PEG-bHb could maintain MAP just as autogenous blood throughout the study period and dextran had lower ability to raise MAP after rat shock. HR showed a positive correlation with MAP (showed in , , , ).
Figure 3. The MAP of rats in hemorrhage shock model. Blood pressure increased when treatment fluids infused. (View this art in color at www.dekker.com.)
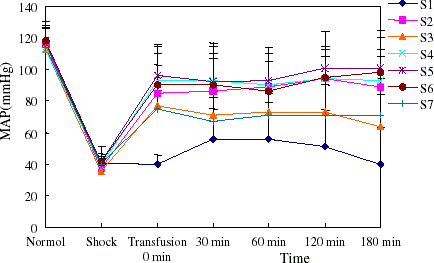
Figure 4. The heart rate of rats in hemorrhage shock model. The heart rate increased with MAP elevation. (View this art in color at www.dekker.com.)
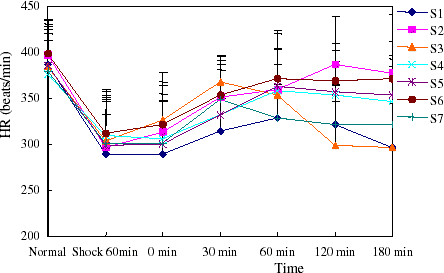
Blood Measurement
Considering the fact that the concentration of Cl− modulates the PEG-bHb capability to release oxygen to the tissues, we detected chloride ion in rat blood. The result did not show any significant [Cl−] difference in rat among groups after fluids infusion and the normal states in two models (Tables and ).
Table 2. Concentration change of Cl− of rats in shock model (mmol/L, x ± s)
Table 3. Concentration change of Cl− of rats in exchange transfusion model (mmol/L, x ± s)
Arterial pH values decreased progressively during hemorrhage in all treatment groups (S1–7, E1–7), and rectified to normal level after transfusion treatment fluids at 30 min, except for the groups of no infusion. The PCO2 and PO2 values varied slightly during the study.
Whole blood viscosity of all groups were evaluated during experiments at different shearing stress of 5 and 200 p/s. Infusion of PEG-bHb could lower the viscosity of experimental rats obviously. Blood viscosity came back to normal through autogenous blood transfusion and decreased mildly by dextran infusion (Tables and ).
Table 4. Changes of blood viscosity of shock model rats at different shearing stress of 5 p/s and 200 p/s (x ± s)
Table 5. Changes of blood viscosity of exchange model rats at different shearing stress of 5 p/s and 200 p/s (x ± s)
Microvascular Variables
Microvascular Diameter
Sixty minutes after shock, a variable narrowing was seen in the arteriolar vessels, which was recovered by transfusion, and the venular vessles diameter didn’t show any change. There's no considerable shrink in arteriolar and venular vessles throughout exchange transfusion in rats.
Blood Flow
Volumetric flow was dramatically reduced in arterioles and venules in the two models. The flow rate recovered nearly to normal by infusion of PEG-bHb, while increased to half of normal with autogenous blood transfusion. Along with the increase of flow rates, blood flow increased compared to hemorrhage. Dextran couldn’t ameliorate microvascular flow rate of the model rats (, , , ).
Figure 7. The flow rate of arteriole in hemorrhage shock model rats. (View this art in color at www.dekker.com.)
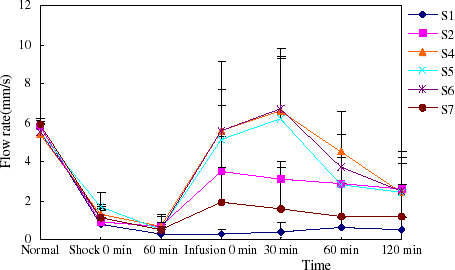
Figure 8. The flow rate of venula in hemorrhage shock model rats. The blood flow rate of venula recovered nearly to normal level when PEG-bHb transfused. (View this art in color at www.dekker.com.)
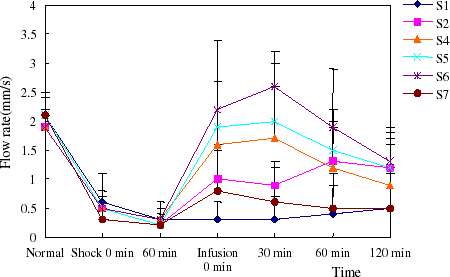
Tissue Oxygenation
To evaluate the oxygen delivery capability of PEG-bHb directly, oxygen tension of liver, muscle, and small intestine was measured by the oxygen dependent quenching of phosphorescence. The results showed that tissue oxygen tension decreased significantly after shock, and could be increased by reinfusion of whole blood and PEG-bHb, while dextran had less capability to raise tissue oxygen tension (p < 0.05, compared to PEG-bHb and whole blood) (Tables , , ).
Table 6. Oxygenation of liver throughout the study in shock model rats (% change from basal, x ± s)
Table 7. Oxygenation of muscle throughout the study in shock model rats (% change from basal, x ± s)
Table 8. Oxygenation of intestine throughout the study in shock model rats (% change from basal, x ± s)
In exchange transfusion model, PEG-bHb had the middle oxygen delivery ability compared with whole blood and dextran ().
Table 9. Oxygenation of tissues throughout the study in exchange model rats (% change from basal, x ± s)
Discussion
To evaluate the pharmacodynamics of PEG-bHb, hemorrhage shock and 50% exchange transfusion models of rats were used in our study. Due to the characteristic of PEG-bHb (e.g., the viscosity and colloid osmolality), its efficiency depends on the dosage used. Three different dose groups were then designed. In two models, the survival rates were higher in 1/2 hemorrhage volume transfusion group (S4, E4) than in groups where isovolume and 1.5-fold volume of PEG-bHb were used. Compared with whole blood and dextran transfusion controls, the results of rats survival showed that the PEG-bHb (when half of hemorrhage volume were used) had the approximate resuscitation effect as the whole blood, and was superior to dextran significantly.
MAP and HR are the indexes to demonstrate the function of cardiovascular system. MAP of rats in two models came back to the normal level nearly when PEG-bHb transfused and even superior to the autogenous blood, which suggests that PEG-bHb has excellent volume expansion function. HR lowered in rats when MAP decreased, maybe lack of functional reflex regulation in the anesthetized rats, and HR recovered with MAP increased to the normal level.
Blood viscosity is an important factor determing microvascular flow, and RBC is the key element to maintain the blood viscosity. Cell-free PEG-bHb has lower viscosity and dilution effect when transfused due to higher colloid osmolality, so PEG-bHb would induce blood viscosity decrease with increased infusion dose. The lower viscosity of blood will reduce the flow resistance to increase tissue blood flow, but there's information argued that moderate viscosity is necessary to maintain blood shearing stress to the vascular wall, which is related with NO synthesis and release, and NO could prevent microvascular contract (de Figueiredo, Citation[[1998]]). This may be one of the reasons why the resuscitation effect was better when 1/2 hemorrhage volume transfused than isovolume and 1.5-fold volume used. Microvascular observation approved that appropriate PEG-bHb infusion could ameliorate the microvascular flow.
Tissue oxygenation measurement provided the direct proof of the oxygen delivery capability of PEG-bHb. The oxygen tension of liver, muscle, and intestine decreased dramatically when rats in shock or 50% exchange transfusion, and raised with PEG-bHb transfusion. Although the recovery effect was limited, it was obviously comparative to whole blood and superior to dextran. In addition, there's another evidence that the survival time of rabbits of 95% exchange transfusion by PEG-bHb was above 25 h, which confirms the oxygen delivery capability of PEG-bHb (data not published in our corporation).
In conclusion, a 6% solution of PEG-bHb can adequately deliver oxygen to tissue and has comparative resuscitation effect with the whole blood in both hemorrhage shock and 50% exchange transfusion rat models. PEG-bHb is an effective blood substitutes.
Acknowledgments
Contract grant sponsor: National High-tech program.
References
- Benesch R., Renthal R., Maeda N. Affinity labeling of the polyphosphate binding site of hemoglobin. Biochem. 1972; 11: 3576–3582
- Center for Biologics Evaluation and Research. Points to consider on safety evaluation of hemoglobin-based oxygen carriers. Transfusion 1991; 31: 369–371
- Conover C. D., Linberg R., Shum K. L., Shorr R. G. L. The ability of polyethylene glycol conjugated bovine hemoglobin (PEG-bHb) to adequately deliver oxygen in both exchange transfusion and top-loaded rat models. Art. Cells, Blood Subs and Immob. Biotech. 1999; 27: 93–107, [CSA]
- de Figueiredo L. F. Vasoactive properties of synthetic blood substitutes. Medicine (B Aires) 1998; 58: 403–410, [CSA]
- Gray S. D. Rat spinotrapezius muscle preparation for microscopic observation of the terminal vascular bed. Microvascular Research 1973; 5: 395–400, [PUBMED], [INFOTRIEVE], [CROSSREF]
- Ketcham E. M., Cairns C. B. Hemoglobin-based oxygen carriers: development and clinical potential. Annals of Emergency Medicine 1998; 33: 326–337, [CSA]
- Squires J. E. Artificial blood. Science 2002; 295: 1002–1005, [PUBMED], [INFOTRIEVE], [CROSSREF]
- Veronese F. M. Peptide and protein PEGylation: a review of problem and solutions. Biomaterials 2001; 22: 405–417, [PUBMED], [INFOTRIEVE], [CROSSREF], [CSA]