Abstract
Development of hemoglobin-based blood substitutes requires production of highly purified hemoglobin. Process of hemoglobin purification by ion exchange chromatography in flow-through mode was researched and optimized. Three kinds of media including, QMA Spherosil LS (Biosepra, France) and Q Sepharose Big Beads (Amersham Bioscience, Sweden), and an anion exchange membrane column, Mustang Q (PALL, USA) were investigated and compared. Adding polyethylene glycol (PEG) as an escort in ion exchange chromatography improved the purity and recovery, and the recovery in the chromatography was increased from 75 to 95%. The mechanism of PEG effects on chromatography was discussed. The optimal chromatography step, in combination with hypotonic dilution hemolyzing and membrane separation, formed an integrated hemoglobin purification process. The total recovery in the process was 87.6%. The activity of hemoglobin was well preserved: P50 23.2 mmHg, and Hill coefficient 2.31. The product appeared as a single band in SDS-PAGE, and GF-HPLC showed only one peak. The purity of the prepared hemoglobin was more than 99.9%. The optimized process is time saving and suitable for large-scale preparation of hemoglobin to provide materials for further preparation of blood substitutes.
Introduction
Development of hemoglobin-based blood substitutes requires production of highly purified hemoglobin for further modification of cross-linking, conjugation, polymerization, and encapsulation (Chang, Citation[[2000]]; Winslow, Citation[[2000]]). Hemoglobin (Hb) resides in red blood cells that can be easily separated from the blood through simple filtration or centrifugation. A careful washing of the cells would remove plasma proteins adsorbed to the cells, thus simplifying further purification of hemoglobin. The content of hemoglobin in the cells is reported to be more than 90% of the total proteins (Riggs, Citation[[1981]]). Release of the hemoglobin from the cells is straightforward either by chemical methods such as toluene and pH treatments or by physical methods such as homogenization, sonication, and osmosis regulation. Hypertonic salt solutions may be employed to swell the membrane of red blood cells and allow the hemoglobin to leak out gradually, while keeping the cell membrane intact (Sheffield et al., Citation[[1987]]).
The challenge, however, is to purify hemoglobin to extremely high purity with low cost. The concentration of hemoglobin in the substitute can be as high as 10 g per 100 mL and the dosage of a blood substitute can be more than 1000 mL. Trace amount of stroma components and other proteins may be doubtful for any adverse effects associated with blood substitutes, such as anaphylactic reactions, immune reactions, renal mal-function and vascular coagulation. Furthermore, methemoglobin without activity will form slowly after the hemoglobin bonds to oxygen, even under low temperature (Dorman et al., Citation[[2002]]; Nedjar-Arroume et al., Citation[[1995]]). The cost of hemoglobin is no doubt the large part of the total cost of a blood substitute, which, at present, is more than human blood.
A number of purification schemes have been developed to prepare hemoglobin, which include at least some lytic step to liberate the hemoglobin from the cellular matrix, a low-resolution fractionation step to remove soluble and insoluble contaminants, followed by some form of chromatographic purification steps. Membrane separation can be used for hemoglobin preparation. The process is simple and easy to scale-up, but it lacks of selectivity. Sheffield et al. (Citation[[1987]]) prepared lipid-free human hemoglobin by dialysis and ultrafiltration with an average 90% hemoglobin recovery and 99% purity. Chromatography can produce high product purity. It is also a step reducing the product recovery. Adsorption chromatography has been widely studied with purity more than 99%. Hsia developed affinity chromatography with ATP-agarose, which increased the purity of hemoglobin significantly (Hsia, Citation[[1996]]). Xue et al. reported another affinity chromatography with ppi and Toyopearl DEAE-650 M, which performed well (Xue et al., Citation[[1998]]), but the preparation was only in lab scale, obtaining grams of product. Christensen and Cheung applied ion exchange chromatography to adsorb hemoglobin with QMA-Acell (Christensen et al., Citation[[1988]]) and DEAE-cellulose (Cheung et al., Citation[[1984]]), which proved that they were practical for preparation of hemoglobin in large scale. Other methods such as metal chelating chromatography (Demmer and Nussbaumer, Citation[[1999]]) and membrane adsorption chromatography (Mehgnin et al., Citation[[2000]]) were studied.
Despite many efforts, it is still difficult to obtain high purity and high recovery of hemoglobin simultaneously. The chromatography methods, including ion exchange and affinity, are operated in adsorption mode, i.e., adsorption of hemoglobin by the solid media. Although the saturation adsorption capacity can be as high as 120 mg per mL medium, the dynamic loading capacity is usually less than 50 mg mL−1 in order to assure high adsorption efficiency. Shorr et al. (Citation[[1993]]) developed a flow-through mode of chromatography, which did not adsorbed hemoglobin but adsorbed impurities. In doing so, it omitted the adsorption and elution steps, obtaining loading capacity as high as 200–250 mg bovine hemoglobin per mL medium with a recovery of 90%. However, the disadvantage was that the purity of hemoglobin was only 95%.
Chromatography in a flow-through mode surely possesses advantages over the adsorption mode in term of process speed and ease of operation. If the process is further optimized with respect to the chromatographic media used, the flowing conditions, and the solution environment, it is possible to achieve high purity and high recovery of hemoglobin simultaneously. We therefore developed a flow-through mode of chromatography with polyethylene glycol (PEG) as an escort. The process was simple and efficient to increase the purity and recovery of hemoglobin. This flow-through chromatography, together with hypotonic release of hemoglobin and membrane filtration of the hemolysate, formed a novel integrated process to obtain highly purified hemoglobin with high product recovery in a few steps.
Materials and Methods
Release of Hemoglobin from the Red Blood Cells
Bovine blood was collected from cattle slaughterhouse with 0.3% citrate sodium as anticoagulan and centrifuged for 15 min at 5,000g, 4°C. Red blood cells (RBC) were washed with 1.2% NaCl for three times under the same condition (Doczi, Citation[[1976]]). Hemoglobin was prepared by a hypotonic dilution technique (Doczi, Citation[[1976]]). Clean packed cells were swelled and hemolyzed after being diluted with ice-cold 20 mmol L−1 phosphate buffer solution (PBS) containing 0.6% NaCl (pH 7.4), and shaken for 1h at 4°C. Then double RBC volume of PBS was pumped at the rate of 10% per minute to keep the osmotic pressure of solution between 160 and 180 mOsm, then continued to shake for 1 h to obtain the hemolysate. The osmotic pressure of the lysate was adjusted to 300 mOsm by adding NaCl.
Pretreatment of Hemolysate
The hemolysate was processed through Millipore M12 membrane system (Millipore, Inc) to remove the ghost and cellular debris. All filtrations were conducted at 4°C. Microfiltration membranes with mean pore size 0.1, 0.22, 0.45, and 0.65 μm were tested. The circulation rate was maintained below 0.3 m/s. The filtration rate was controlled by adjusting the valve on the retentate line. In order to reduce concentration polarization and membrane fouling, the pressure on the retentate was controlled not to exceed 0.05 MPa.
The filtrate was then put through ultrafiltration to concentrate the hemoglobin and to remove low molecular weight impurities. Ultrafiltration membranes with MWCO 5, 10, 30, and 50 K were tested. Millipore M12 membrane system was used with the ultrafiltration modules.
Anion Exchange Chromatography (IEC) in Flow-Through Mode
Chromatography was performed on Waters 650E Protein Purification System. Commercial anion exchange media including QMA Spherosil LS (Biosepra, France) and Q Sepharose Big Beads (Amersham Bioscience, Sweden), and an anion exchange membrane column, Mustang Q (PALL, USA) were used for comparison purpose. The media were packed with a volume of 10 mL into an XK16/20 (20 × 1.6 cm) column from Amersham Biosciences (Sweden) except the membrane column that had been prepacked by the manufacturer (V = 10 mL). All chromatographic runs were monitored by on-line measurement of conductivity and UV absorption at 280 nm.
The chromatographic operation, automatically controlled by the system, comprised basically the following steps. First, the columns were equilibrated with 5 column volumes of 10 mmol L−1 PBS at a flow rate of 75 cm h−1. The pH value of loading and rinsing buffer and hemoglobin sample was adjusted to 6.4, 6.8, 7.4, and 7.8 respectively with QMA Spherosil LS, Q Sepharose Big Beads and a Mustang Q membrane column.
Fractions directly flowing through during loading were collected for further analysis of hemoglobin content. Impurities were eluted by 10 mmol L−1 PBS containing 1 mol L−1 NaCl at a flow rate of 150 cm h−1.
In polyethylene glycol escorting ion exchange chromatography, PEG600 and PEG4000 were added into the equilibrium and rinsing buffer with concentrations of PEG varying from 0.75 to 5%, pH 7.8.
Analytical Methods
Osmotic pressure was measured in the process of RBC swelling and lysating by Fiske ONE-TEN™ Osmometer (Fiske Associates, USA). The concentration of protein was determined according to the Bradford (Citation[[1976]]) method. The activity of hemoglobin, in terms of P50 and Hill coefficient, was measured by Hemox™ Analyzer (Bruno and Gino, Citation[[1981]]) (TCS Scientific Corp., PA).
Hemoglobin samples were subjected to sodium dodecylsulfate-polyacrylamide gel electrophoresis (SDS-PAGE). A 4% stacking gel with a 15% running gel was used with 10 μL of hemoglobin solution on a minivertical slab gel apparatus (Bio-Rad, USA) at 200 v for approximately 1 h. A discontinuous Tris-glycine-SDS buffer system was used. After electrophoresis, the gel was then stained with Coomassie blue R250 and analyzed by Bio-Rad Gel Documentation Systems (Gel Doc 2000™).
HPLC analysis was conducted on the purified hemoglobin with an instrument equipped with a variable wavelength spectrophotometric detector (BECKMAN 126) on TSK 3000SW column at 280 nm. Lipid was detected by high performance thin-layer chromatography (HPTLC) (Donald, Citation[[1997]]).
Results and Discussion
Release of Hemoglobin from the Red Blood Cells
In this process, we found that cell wash and osmotic regulation were two key steps. At least three times of wash were necessary to get rid of leukocytes, platelets, lipids and plasma proteins that adsorbed to the cells. Extensive wash can remove trace contaminants from the plasma, but the rupture of the cells is a problem. Liquid shear could be the cause of cell breakage, which appears in the re-suspension of the cell paste. Gentle shaking was found to alleviate the problem.
For release of hemoglobin, we adopted the method of osmotic regulation (Deloach et al., Citation[[1986]]; Dodge et al., Citation[[1963]]). Hypertonic salt solution was employed to raise the osmotic pressure to 160–180 mOsm. Hemoglobin was released from the cells while the cell membranes were intact. The hemolysate, e.g., the suspension after hemoglobin release, was then adjusted to 300 mOsm to preserve the activity of hemoglobin, the latter being the osmotic pressure at which hemoglobin existed in its original environment.
The removal of the intact cell membranes, known as the ghost, can be done either by high speed centrifugation or by membrane filtration. In this experiment, we used two membrane steps. The first one was microfiltration with membranes of mean pore size 0.1, 0.22, 0.45, and 0.65 μm. The second membrane step was ultrafiltration with ultrafiltration membranes of MWCO 5, 10, 30, and 50 K. gives the results of two combinations of the membrane process. The second combination, i.e., filtration with a 0.45 μm membrane followed by ultrafiltration with a 10 kD membrane gave a better performance on the process speed, recovery and bioactivity. Larger membrane pore as 0.65 μm would result in passage of some small debris of the membrane. For ultrafiltration, 10 and 30 kD produced similar results in purity, while the recovery was higher with 10 kD membrane. demonstrates the SDS-PAGE result of the hemolysate after the two membrane steps. The main band of MW 16 kD is the subunit of hemoglobin. There were carbonic anhydrase with a MW of 31 kD and other small amount of impurities with molecular weights of 64 and 97 kD, which indicates the necessity of further purification.
Figure 1. SDS-PAGE Electrophoretogram of hemoglobin in improved pretreatment process. 1. Bovine hemoglobin standard (Sigma), 2. MW marker, 3. lysate, 4. filtrate of 0.45 µm membranes, 5. retentate of 30 KD membranes.

Table 1. Comparison of two pretreatment methods
Optimization of Ion Exchange Chromatography in Flow-Through Mode
According to the different characteristics between hemoglobin and the main impurities, as well as the requirement of fast preparation of hemoglobin in large scale, three kinds of anion exchange media, QMA Spherosil LS and Q Sepharose Big Beads, and Mustang Q were selected to give comparison. The pH is one of most important parameters in determining protein binding, as it determines the change on both the protein and the ion exchanger. Other parameters, such as concentration of ions, the flow rate, sample loading capacity and temperature are related to the resolution of chromatography and bioactivity of hemoglobin. So the pH was first optimized respectively in the chromatography with the three different media for comparison, and made the other conditions be the same. Our previous researches showed that the formation of methemoglobin was decreased at low temperature and low concentration of buffer (Lu et al., Citation[[2003]]). Therefore, the chromatography was performed at 4°C and 10 mmol L−1 PBS was chosen as balance and rinsing buffer. Low sample loading and low flow rate were applied for better resolution, which was 20 mg protein and 75 cm h−1 respectively.
Among the three media tested in this article, the ion exchange membrane module Mustang Q was a novel chromatographic support. It was a stack of flat sheet membranes having a nominal 0.8 µ pore size. According to the manufacturer, the membranes were polyethersulfone membranes chemically modified with charged, hydrophilic monomers, which were cross-linked to produce quaternary amine surface. This open pore structure provided a large, accessible surface area for protein binding without the problem of diffusional limitation that often occurs in the media of porous beads.
Our experiments proved that this membrane module could be operated at very high flow rate without sacrifice of its resolution. The pressure drop was small. With this membrane column, 99% of hemoglobin was recovered. However, the purity of the product was a problem. is a picture of SDS-PAGE of the hemoglobin fraction from the membrane module. Lane 1, 2, and 3 showed three samples from three runs with varied pH. All indicates the presence of impurity bands, which might attribute to the big pore structure of the membranes. The nominal 0.8 µ pore size is more than one hundred times the size of most protein molecules. As the feed flowing through the membrane, impurities through the central part of the pore might not be adsorbed by the ion groups on the walls of the membrane. As a consequence, they were carried away with the hemoglobin fraction flowing though the column.
Figure 2. SDS-PAGE electrophoretogram of hemoglobin chromatography with Q Mustang at various pH values. 1. pH 7.8; 2. pH 7.6; 3. pH 7.4; 4. MW marker; 5. Bovine hemoglobin standard (Sigma).
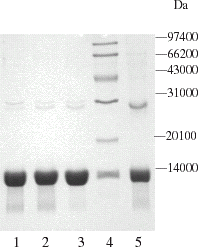
The second medium was QMA Spherosil LS, a composite chromatographic medium with porous silica as the backbones and hydrophilic polymer as the coating layer. Ion exchange groups were conjugated to the polymer surface. This sorbent provides both ionic and slightly hydrophobic properties. This unique mix mode chromatography allows the purification of proteins difficult to isolate on classical media. Experimental results proved that it was efficient in removing impurities such as carbonic anhydrase and the purity of hemoglobin was more than 99% ( lane 7). However, hemoglobin adsorption was a problem. It was found that a portion of hemoglobin was retained by the adsorbent possibly due to hydrophobic interaction. As a result, the recovery of hemoglobin was as low as 50%.
Figure 3. SDS-PAGE electrophoretogram of hemoglobin chromatography with QMA Spherosil LS and Q Sepharose Big Beads at various pH values. 1. Q Big Beads pH 6.4; 2. Q Big Beads pH 6.8; 3. Q Big Beads pH 7.4; 4. Q Big Beads pH 7.8; 5. lysate; 6. ultrafiltrated with 30 KD membrane; 7. QMA pH 7.4; 8. QMA pH 6.8; 9. Marker.
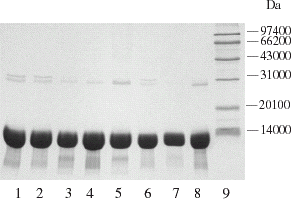
Q Sepharose Big Beads, a kind of quaternary ammonium strong anion exchanger, is based on 100–300 μm agarose beads. Due to its excellent physical stability and large bead size, Q Sepharose Big Beads can be run at high flow rates even with viscous feeds. The experiment results showed that at pH 7.8, most impurities adsorbed onto the medium while hemoglobin flowed through the column without being retained. The purity of hemoglobin was over 99% ( lane 4). The recovery of hemoglobin was 75%. We also tested DEAE Sepharose Fast Flow. It did not give a better result than Q Sepharose Big Beads. Its flow rate was lower and pressure drop was higher. Among the chromatographic media tested, Q Sepharose Big Beads appeared to be the best choice.
In hemoglobin purification, albumin and carbonic anhydrase (CA) were the two main contaminants. Albumin came from plasma and was due to incomplete washing of the red blood cells. If careful and extensive wash was carried out, the albumin contaminant could be minimal. CA, on the other hand, is difficult to remove. In red blood cells, CA bonds to hemoglobin tightly to assist hemoglobin in oxygen liberation and carbon oxygen delivery. When hemoglobin was released under osmotic pressure, CA was released too. The isoionic point (pI) of bovine CA is 6.0 and the pI of albumin is 4.9, which were lower than that of hemoglobin. Therefore, removal of CA and albumin could be possible through ion exchange selection.
The pI of bovine hemoglobin (Hb) is 6.9. Theoretically, if pH is selected between 6.1 and 6.8, Hb would flow through an anion exchange column due to its positive change, while albumin and CA would be retained due to their negative changes. However, varied amount of CA appeared in the fraction of flow through with the Hb in the pH range of 6.1–6.8. Increasing pH to the value above 7.0 could further decrease the contamination of CA in Hb fraction. This can be seen from and where higher pH than the pI of Hb as 7.4–7.8 improved the purity, but the recovery of Hb decreased too. In the case of QMA Spherosil, Hb recovery was as low as 50% at pH 7.4. It is understandable that at such a pH Hb was negatively changed, and was adsorbed by the medium through ion exchange interaction. We also tried to control the ionic strength of the buffer in order to minimize Hb adsorption at high pH while allowing CA, which has a stronger negative change than Hb at the pH far from its pI, had a high tendency to be adsorbed. Phosphate buffer containing 15, 30, and 50 mmol L−1 NaCl (pH 7.8) was applied to chromatography with Q Sepharose Big Beads. There was an improvement in recovery, up to 90%, as the ionic strength increased, but the contents of CA increased too.
It seems that the selection of a pH not far from a protein's pI cannot produce a clear cut to produce a complete separation of the protein in this flow-through chromatography. There was a trade-off between the purity and the recovery. As we had considered both the pH and ionic strength, the next possible optimization strategy should be the influence of nonionic and noncharge factors. For this aspect, we investigated the addition of a cosolvent, polyethylene glycol, to improve the purity and the recovery.
Ion Exchange Chromatography in the Presence of PEG
Polyethylene glycol (PEG) has been widely used in protein fractionation, crystallization and two-aqueous- phase extraction, especially for chemical modification (Inada, Citation[[1995]]), e.g., connecting with proteins. PEG, which is slightly hydrophobic, can inhibit protein aggregation, and help protein folding and refolding (Cleland and Randolph, Citation[[1992]]), thus serving as a protein stabilization reagent. In gel filtration chromatography and hydrophobic interaction chromatography, PEG can improve the partition coefficient (Yan et al., Citation[[1984]]; Zhao et al., Citation[[2002]]). Recent research showed that PEG in ion exchange chromatography could change the polarity of solution and the partition coefficient (Cagnon et al., Citation[[1996]]; Timasheff, Citation[[1992]]).
PEG600 and PEG4000 were applied at concentrations varying from 0.75 to 5% for the flow through chromatographic purification of hemoglobin. is the result. The electrophoretogram showed that the purity of hemoglobin was generally improved, especially when 0.75%PEG600 (pH 7.8) was added. There was only a single band in SDS-PAGE. The recovery was improved to 95% as shown in . Furthermore, the activity of hemoglobin was well preserved during chromatography with P50 of 23.2 mm Hg, and Hill coefficient of 2.31, as compared to the control of P50 of 24.26 mm Hg, and Hill coefficient of 2.48 before chromatography (). In the scanning profile of SDS-PAGE electrophoretogram, the purified hemoglobin appeared only as one peak after purification (), the same as the result in GF-HPLC (). No phosphatidylethanol (PE) and phosphatidylserine (PS) was detected by HPTLC. The purity was analyzed to be more than 99.9%. Results were the same when the sample loading was improved to 300 mg hemoglobin per mL media and the flow rate raised to 150 cm h−1.
Figure 5. Profile of hemoglobin with PEG600 escorting chromatography. Chromatographic column was packed with 10 mL Q Sepharose Big Beads; equilibrium buffer: 10 mmol L−1 PBS, pH 7.8; loading quantity: 100 μL protein with a concentration of 200 mg mL−1; loading rate: 25 cm h−1, 5 min later the flow rate was raised to 75 cm h−1, rinsed until hemoglobin flow through completely (45 min later), absorbed hemoglobin and impurities were eluted by 10 mmol L−1 PBS containing 1 mol L−1 NaCl at a flow rate of 150 cm h−1.
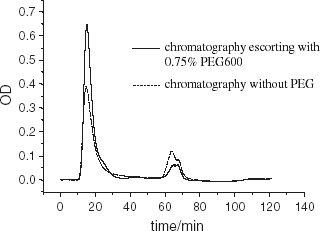
Figure 7. Scanning profile of SDS-PAGE electrophoretogram for purified hemoglobin. (View this art in color at www.dekker.com.)
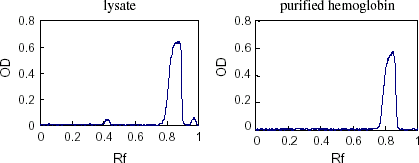
Figure 8. High performance gel filtration liquid chromatography analysis of purified hemoglobin. Chromatography column: TSK 3000SW, 7 μm, 250 × 4.5 mm; mobile phase: 50 mmol L−1 PBS containing 100 mmol L−1 NaCl, pH 7.2: loading quantity: 20 μL, flow rate: 1.0 mL min−1, detected at 280 nm.
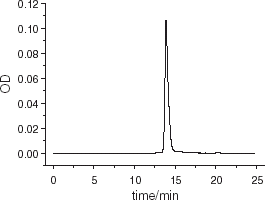
Gagnon et al. (Citation[[1996]]) observed that the addition of PEG to the mobile phase of ion exchange chromatography could alter the retention behavior of a number of proteins. The experiments were conducted with purchased pure proteins. Feng et al. (Citation[[1998]]) carried out investigations with real purification of recombinant human tumor necrosis factor-α and found that when PEG 200, 600, or 4000 were added to the buffer, nearly one-fold increase in the purification and about 100% recovery was obtained during anion-exchange chromatography. However, the mechanism of PEG effect on chromatography has not been well understood. According to Timasheff 's (Citation[[1992]]) preferential hydration hypothesis, when PEG is present in a protein solution, PEG molecule did not surround the protein molecule to form a so-called “PEG protecting layer” or cover the structure domain of protein. Instead, PEG and protein compete for water molecules to form their individual hydration layer. The hydration layer is composed of ordered water molecules. Consequently, the whole system assumed asymmetry thermodynamically, and protein molecule kept compacting to reduce its size and apparent area. As a result, the surface property of the protein, including its interaction ability with solid ion exchange media, may be changed. Such a change would exert different effects on different proteins, so that their ion exchange behaviors could be changed.
Protection of Hemoglobin Bioactivity in Lyophilizing
Lyophilization is a good way for protein preservation. Lyophilized hemoglobin can be kept at room temperature for half a year or stored at 4°C for two years without losing activity (Janson and Ryden, Citation[[1997]]). Before lyophilization, salt and PEG600 should be removed by ultrafiltration with 30 kD membranes. No PEG was detected by titration method in the hemoglobin solution after the ultrafiltration (Ingham and Busby, Citation[[1980]]). In the process of lyophilization, the structure of protein will be affected to some extent. The protection effects of various content of glucose, sucrose and mycose are compared in (the concentration of hemoglobin is 300–400 mg/mL). It has been known that protein activity can be preserved in lyophilization by adding sugar (Janson and Ryden, Citation[[1997]]). demonstrated that 3% sucrose and 3% mycose were the two good protectants. Taking the cost into consideration, 3% sucrose is finally chosen as the protection reagent for lyophilizing hemoglobin.
Table 2. Protection effect of various sugar on hemoglobin activity in lyophilizing
Conclusion
A flow-through mode of ion exchange chromatography, in combination with hypotonic dilution hemolysing and membrane separation steps, formed an integrated hemoglobin purification process. Hemolysate of red blood was pretreated with a 0.45 μm membrane followed by an ultrafiltration membrane having a cut-off molecular size of 10 kD. In comparison of the three media, including QMA Spherosil LS, Q Sepharose Big Beads, and Mustang Q, the purity of hemoglobin was the highest when Q Sepharose Big Beads was used with 10 mmol L−1 phosphate buffer solution (pH 7.8), but the recovery was only 75%. This problem was well solved by using 0.75% PEG600 as an escort in the chromatography. The recovery was increased to 95%. The purity of the hemoglobin prepared was more than 99.9%. The activity (P50) of hemoglobin was well preserved. The loading capacity reached 300 mg Hb per mL medium in the flow-through mode of chromatography. After chromatography, the hemoglobin solution was de-salted and the PEG was removed by ultrafiltration, and then lyophilized with 3% sucrose as a protection reagent. High activity of hemoglobin powder (P50 22.4 mm Hg, Hill coefficient 2.22) was obtained and the total recovery reached 87.6%. The bioactivity, purity and recovery of hemoglobin in an optimized process are shown in .
Table 3. Bioactivity, purity and recovery of hemoglobin in the optimal process
The optimized process needs less time to produce highly purified hemoglobin with high recovery, and it is suitable for large-scale preparation of hemoglobin to provide materials for blood substitutes.
Acknowledgments
The authors acknowledge the financial support received from the National Natural Science Foundation of China (No. 20136020).
References
- Bradford M. M. A rapid and sensitive method for the quantification of microgramme quantities of protein utilizing the principle of protein-dye binding. Analytical Biochemistry 1976; 72: 248–254, [PUBMED], [INFOTRIEVE]
- Bruno G., Gino A. Measurement of binding of gaseous and nongaseous ligands to hemoglobin by conventional spectrophotometric procedure. Methods in Enzymology 1981; 76: 417–427
- Cagnon P., Godfrey B., Ladd D. Method for obtaining unique selectivities in ion-exchange chromatography by addition of organic polymers to the mobile phase. J. Chromatography 1996; 743: 51–55, [CROSSREF]
- Chang T. M. S. Red blood cell substitutes. Best Pract. Res. Clin. Haematol. 2000; 13: 651–668, [CROSSREF], [CSA]
- Cheung L. C., Storm C. B., Babriel B. W. The preparation of stroma-free hemoglobin by DEAE-cellulose absorption. Anal. Biochem. 1984; 137: 481–484, [PUBMED], [INFOTRIEVE], [CROSSREF]
- Christensen S. M., Media F., Winslow R. M., Snell S. M., Zegn A., Marini M. A. Preparation of human hemoglobin A0 for possible use as a blood substitute. J. Biochem. Biophys. Methods 1988; 17: 143–154, [PUBMED], [INFOTRIEVE], [CROSSREF], [CSA]
- Cleland J. L., Randolph T. W. Mechanism of polyetheylene glycol interaction with the molten globule intermediate on the carbonic anhydrase folding pathway. J. Biol. Chem. 1992; 267: 3147–3153, [PUBMED], [INFOTRIEVE]
- Deloach J. R., Sheffield C. L., Spates G. E. A continuous-flow high-yield process for preparation of lipid-free hemoglobin. Anal. Biochem. 1986; 157: 191–198, [PUBMED], [INFOTRIEVE], [CROSSREF]
- Demmer W., Nussbaumer D. Large-scale membrane adsorbers. Journal of Chromatography A 1999; 852: 73–81, [PUBMED], [INFOTRIEVE], [CROSSREF]
- Doczi J.. Injectable Stroma Free Hemoglobin and Its Method of Manufacture. US Patent 3,991,181, 1976
- Dodge J. T., Mitchell C., Hanahan D. The preparation and characteristics of hemoglobin-free ghosts of human erythrocytes. Arch. Biochem. Biophys. 1963; 100: 119–130, [PUBMED], [INFOTRIEVE], [CROSSREF]
- Donald J. H. A Guide to Phosphalipid Chemistry. Oxford University Press, New York 1997
- Dorman S. C., Kenny C. F., Miller L., Hirsch R. E., Harrington J. P. Role of redox potential of hemoglobin-based oxygen carriers on methemoglobin reduction by plasma component. Art. Cells, Blood Subs., and Immob. Biotech. 2002; 30(1)39–51, [CROSSREF]
- Feng X. L., Gu Z. Y., Jin Y. T., Su Z. G. Polyethylene glycol improves the purification of recombinant human tumor necrosis factor during ion exchange chromatography. Biotechnology Techniques 1998; 12(4)293–298, [CROSSREF]
- Hsia J. C. Purification of hemoglobin and modified hemoglobin by ATP-agarose affinity chromatography. J. Chromatogr. 1996; 374: 143–148
- Inada Y. Biomedical and biotechnological applications of PEG- and PM-modified proteins. Trends in Biotechnology 1995; 13: 86–91, [PUBMED], [INFOTRIEVE], [CROSSREF], [CSA]
- Ingham K. C., Busby T. F. Methods of removing polyethylene glycol from plasma fractions. Chem. Eng. Commun. 1980; 7: 315–326
- Janson J. C., Ryden L. Protein Purification: Principles, High Resolution Methods, and Applications. 2nd ed. John Wiley & Sons, Inc. Publication, New York 1997; 3–41
- Lu X. L., Zhao D. X., Jin Y. T., Su Z. G. Efficient separation and purification of bovine hemoglobin using expanded bed adsorption. Journal of Chemical Industry and Engineering 2003; 54(9)1257–1263
- Mehgnin E. A., Plajura D. H., Ehshraf S.. Method of Commercial-scale Purification of Hemoglobin. RU Patent 2,145,873, February 27, 2000
- Nedjar-Arroume N., Castellane A., Guillocbon D. Stabilizing effect of water/alcohol solvents towards autoxidation of glutaraldehyde-modified haemoglobin. Biotechnol. Appl. Biochem. 1995; 21: 173, [PUBMED], [INFOTRIEVE], [CSA]
- Riggs A. Methods in Enzymology, E. Antonini, L. Rossi-Beradi, E. Chiancone. Academic Press, Orlando, FL 1981; Vol. 76: 5–29
- Sheffield C. L., Spates G. E., Droleskey R. E., Green R., Deloach J. R. Preparation of lipid-free human hemoglobin by dialysis and ultrafiltration. Biotechnol. Appl. Biochem. 1987; 9: 230–238, [PUBMED], [INFOTRIEVE], [CSA]
- Shorr R. G., Nho K., Cho M. O.. Process for Hemoglobin Extraction and Purification. US Patent 5,264,555, November 23, 1993
- Timasheff S. N. Stabilization of protein structure by solvent additives. Stability of Protein Phamarceuticals (Part B), T. J. Ahern, M. C. Manning. Plenum Press, New York 1992
- Winslow R. M. Blood substitutes. Advanced Drug Delivery Reviews 2000; 40: 131–142, [PUBMED], [INFOTRIEVE], [CROSSREF], [CSA]
- Xue H., Zheng H., Wu X. F. Preparation of highly purified hemoglobin by affinity elution. Artif. Cells Blood Substit. Immobil. Biotechnol. 1998; 26(3)317–327, [PUBMED], [INFOTRIEVE], [CSA]
- Yan S. C. B., Tuason D. N., Tuason V. B. Polyethylene glycol interferes with protein molecular weight determination by gel filtration. Anal. Biochem. 1984; 138: 137–140, [PUBMED], [INFOTRIEVE], [CROSSREF]
- Zhao D. X., Wei X. G., Gu Z. Y., Zhang G. F., Su Z. G. Preparation of bovine lipid-free hemoglobin. Chinese Journal of Biotechnology 2002; 18(5)609–613, [PUBMED], [INFOTRIEVE]