Abstract
Ruminococcus gnavus is a Gram positive, nonspore-forming obligate anaerobe normally found in the human alimentary tract. In culture, this organism constitutively produces a 1–3 α-galactosidase. We fractionated and characterized this enzyme demonstrating hydrolysis of the B epitope on erythrocyte membranes and seroconversion to H epitope (blood type O). Since the enzyme yield was low, cell suspension studies could not be performed. Instead, hydrolysis of the B membrane epitope was studied with an ELISA. A highly purified enzyme product was analyzed for characteristics such as pH, ionic strength, and temperature optimum. Activity in red cell preservative solutions and in the presence of type B plasma was also demonstrated. Ruminococcus gnavus a 1–3 α-galactosidase has potential application in the enzymatic conversion of type B to O packed red blood cell units.
Introduction
In the ABO blood group system, the erythrocyte membrane epitopes consist of carbohydrate chains with unique immunodominant terminal sugar residues (KoScielak, Citation[[2001]]; Schenkel-Brunner, Citation[[2001]]). Cleavage of these terminal sugar groups alters antigenicity preventing antibody binding and subsequent complement fixation (Roseman, Citation[[2001]]).
Through the action of certain exoglycosidases, it is possible to convert specific blood group antigens. Several lysosomal exoglycosidases have demonstrated this effect on human erythrocytes (Goldstein et al., Citation[[1982]]; Goldstein, Citation[[1984]]; Haibach et al., Citation[[1991]]). However, these enzymes have a distinct disadvantage, their low pH optima. This requires high enzyme concentration to achieve red cell seroconversion at low pH (Vosnidou et al., Citation[[1998]]). Current technology also requires lowering the conversion pH to 5.6 by washing, then backwashing the treated red cells to neutral pH, resulting in increased red cell loss and cost of seroconversion (Kruskall et al., Citation[[2000]]).
In order to demonstrate the feasibility of erythrocyte antigenic conversion using prokaryotic exoglycosidases, several important outcomes must be assessed. The selected exoglycosidases must exhibit a high activity for unique ABO antigenic sites leaving other red blood cell structure and function unaffected. Ideally, an enzyme used in the alteration of surface antigens would function in the pH range of 6.4 to 7.2 found in red cell units. There is ample evidence that prokaryotic exoglycosidases exhibit pH optima much closer to this pH range than lysosomal enzymes currently used in deantigenation studies (Dybus and Aminoff, Citation[[1983]]; Hoskins and Boulding, Citation[[1976]]; Hoskins, Citation[[1981]]; Hoskins et al., Citation[[1985]]; Larson et al., Citation[[1988]];). An exoglycosidase functional at a neutral pH would also eliminate the need to wash erythrocytes prior to deantigenation (Lublin, Citation[[2000]]). Finally, it would be desirable to have an enzyme active in the presence of plasma. An enzyme with these properties could be directly added to red cell units to achieve enzymatic conversion.
Interestingly, some strains of Ruminococcus gnavus constitutively produce a α-galactosidase that degrades the human blood group B antigen (Hoskins, Citation[[1981]]; Hoskins et al., Citation[[1985]]; Larson et al., Citation[[1988]]). The possibility of converting erythrocyte membrane epitopes in red cell units could help meet the demand for group O units. This paper characterizes some properties of the hydrolytic activity of Ruminococcus gnavus α-galactosidase on the blood type B erythrocyte membranes, and under some conditions found in packed red blood cell units.
Materials and Methods
Materials
Macro-Prep ceramic hydroxyapatite CHT-5, Bio-Gel HPHT HPLC columns, SDS polyacrylamide gels, and protein determination reagent were purchased from Bio-Rad, Hercules, California. Mouse monoclonal anti-H antibody was purchased from Dako Corporation, Carpinteria, California. Ruminococcus gnavus strain number 35913 was purchased from the American Type Culture Collection (ATCC), Rockville, Maryland. Dehydrated cooked meat medium was obtained from Difco, Detroit, Michigan. Monoclonal anti-B antiserum was purchased from Ortho Diagnostic Systems, Raritan, New Jersey. Immulon 4 flat bottom microtiter plates were purchased from Dynatech Laboratories, Chantilly, Virginia. All other chemicals, substrates, and chromatography resins were the highest purity available from Sigma Chemical Company, St. Louis, Missouri, or Fisher Scientific, Pittsburgh, Pennsylvania. Type B packed red blood cells and fresh frozen plasma were obtained from the American Red Cross, St. Louis, Missouri. Red cell preservative solutions were prepared using standard formulas as follows: Citrate-phosphate-dextrose (CPD) containing 26.30 g trisodium citrate, 3.27 g citric acid, 25.50 g glucose and 2.22 g monobasic sodium phosphate per liter deionized water; Citrate-phosphate-dextrose-adenine-one (CPDA-1) contained 26.30 g trisodium citrate, 3.27 g citric acid, 25.50 g glucose, 2.22 g monobasic sodium phosphate and 0.27 g adenine per liter deionized water; Adsol additive solution (AS-1) containing 0.90 g sodium chloride, 0.27 g adenine, 2.20 g glucose and 0.75 g mannitol per liter deionized water. Final pH of all undiluted preservative solutions was 5.6. The diluents, phosphate-buffered-saline (PBS) contained 50 mM monosodium phosphate and 100 mM NaCl, pH 7.0.
Methods
Enzyme Purification and Characterization
Purification was based upon a modification of the method of Larson et al. (Citation[[1988]]) by adding low and high-pressure hydroxyapatite chromatographic and chromatofocusing steps. Protein concentration was determined by the method of Bradford with BSA as a standard (Bradford, Citation[[1976]]). Polyacrylamide gel electrophoresis and analytical molecular sieve chromatography were performed as described by Hata et al. (Citation[[1992]]).
Enzyme Linked Immunosorbent Assays (ELISA)
Erythrocyte membrane preparation, microplate coating techniques, and activity assays for R. gnavus α-galactosidase were based upon the ELISA methodology developed by Hobbs et al. (Citation[[1993]]). Microplate wells were coated with type B erythrocyte membranes, treated with R. gnavus α-galactosidase under varying conditions, probed with murine monoclonal anti-IgM, and developed with μ-chain specific alkaline phosphatase conjugate, followed by p-nitrophenyl phosphate substrate. Absolute values for positive controls and test wells were determined by subtracting the mean of the negative control wells, from the mean of the positive controls or test wells. Calculation of enzymatic activity based upon a 24-h incubation period was as follows: OD415 untreated control well−OD415 enzyme containing well = ΔOD415. One unit of enzyme activity was defined as. a ΔOD415 = 0.5.
Results
Enzyme Purification
The use of hydroxyapatite was useful in last steps of purification. Specific activity increased from 11.3 U mg−1 in the crude preparation to 4937.4 U mg−1 in the final preparation. This resulted in 418-fold purification, and a yield of 0.32% or 30 μg of enzyme. The final preparation was heterogeneous with multiple high molecular weight bands observed by 7.5% SDS-PAGE. Analytical Sephacryl S-300 chromatography approximated native molecular weight of enzymatically active fractions to be 673 and 326 kD.
ELISA Studies
The enzyme concentration curve established a curvilinear relationship between concentration of purified R. gnavus α-galactosidase and ΔOD415, . The change in absorbance at OD415 measured hydrolysis of the terminal galactose 1–3 α-galactosyl residue from the blood group B epitope. As expected, binding of monoclonal anti-B decreased as enzyme concentration increased. An enzyme concentration of 10.7 units mL−1 generated a reproducible ΔOD415 of approximately 0.50. The reaction volume was 90 μL. Thus each reaction contained 0.96 units, or 194 ng of enzyme. Based upon the signal generated, and extremely limited enzyme stocks, an enzyme concentration of 10.7 units mL−1 was chosen for all subsequent R. gnavus α-galactosidase characterization studies. As demonstrated in , R. gnavus α-galactosidase increased expression of the H epitope (blood type O) after exoglycosidase treatment of type B membranes. In this experiment absolute OD415 was used to demonstrate the reciprocal relationships of anti-B and -H binding.
Figure 1. Degradation of the B epitope as a function of enzyme concentration. A purified preparation of R. gnavus α-galactosidase was serially diluted into PBS, pH 7.0. Enzyme treatment of B membrane coated microplates and ELISA assay were performed as previously described. Error bars indicate range of ΔOD415. All data points are the product of three independent duplicate determinations.
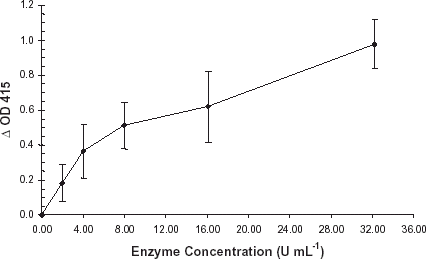
Figure 2. H antigen expression. A purified preparation of enzyme was diluted with PBS, pH 7.0, and applied to a B membrane coated plate. Negative controls consisted of PBS, pH 7.0, incubated under the identical conditions. Enzyme treated wells and negative controls were developed per normal procedure. Primary antibody consisted of monoclonal anti-B (1:100) or monoclonal anti-H (1:10). Error bars indicate range of OD415. All data points are the mean of two independent duplicate determinations.
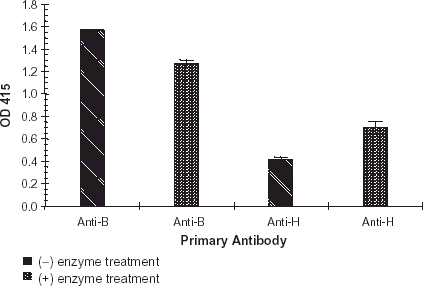
The effect of pH on enzymatic activity investigated the pH range at which the purified R. gnavus α-galactosidase hydrolyzed the terminal galactose from type B membranes. Incubation in pH buffers 5.5–7.5, did not markedly affect the hydrolytic activity of R. gnavus α-galactosidase. Results of the ionic strength study indicated that in 5 mM sodium phosphate, pH 7.0, maximal ΔOD415 was noted at 200 mM NaCl. Interestingly, at 1600 mM NaCl, the ΔOD415 was 62% of the value measured at 200 mM. Lower concentrations of NaCl (140 mM) reduced enzyme activity by 33% compared to the 200 mM optimum.
In an additional experiment, R. gnavus α-galactosidase demonstrated hydrolytic activity with type B membranes over a temperature range of 4 to 37°C. Noteworthy was that hydrolysis at 24 and 37°C was 81% and 57% respectively of that observed at 4°C. The effect of buffer species concentration on the hydrolysis of the terminal galactose from type B membranes by purified R. gnavus α-galactosidase was assessed. Sodium citrate and sodium phosphate concentrations were varied from 10 to 80 mM, pH 7.0, in the presence of 140 to 60 mM NaCl to approximate isotonic conditions. As measured by OD415, variations in buffer concentrations appear to have had little effect on the hydrolysis of the B epitope. In citrate buffers, maximal ΔOD415 was achieved in the solution containing 20 mM Na citrate + 120 mM NaCl, pH 7.0. Minimal ΔOD415 was noted with 40 mM Na citrate + 100 mM NaCl, pH 7.0. When phosphate concentrations were varied, maximal ΔOD415 occurred in 10 mM Na phosphate + 140 mM NaCl, pH 7.0.
ELISA studies were performed to determine effects of diluted red cell preservatives on R. gnavus enzymatic activity. Red cell preservative solutions AS-1, CDPA-1 and CPD were diluted 1:10 with PBS, pH 7.0 containing dilute R. gnavus α-galactosidase. All dilutions were applied to a B membrane coated plate, and incubated at 37°C for 24 h. Plates were developed as described. Results of this study were similar to that of the undiluted red cell preservative solution study. CPDA-1 produced a slightly greater ΔOD415 (0.30) than did AS-1 (0.23) or CPD (0.22), . In addition, enzyme was diluted into CPD, pH 6.25, 6.50, 6.75 and 7.0. In CPD solution, α-galactosidase activity was highest at pH 6.25 (ΔOD415 0.47), .
Figure 3. Diluted red cell preservative study: Adsol, CPDA-1, and CPD. Red cell preservative solutions Adsol, CPDA-1, and CPD were diluted 1:10 with PBS, pH 7.0. A purified preparation of enzyme was diluted with each of these solutions. The enzyme treatment and ELISA plates were developed per normal procedure. Bars indicate change in OD415. Error bars indicate range of ΔOD415. Results are the mean of two independent duplicate determinations.
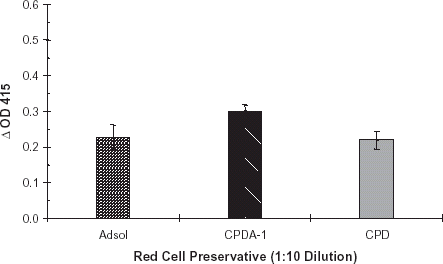
Figure 4. Red cell preservative pH study. Red cell preservative CPD, pH 6.25, 6.50, 6.75 and 7.0 was prepared. A purified preparation of enzyme was diluted with each of these solutions. The dilutions were applied to B membrane coated ELISA plates and developed per normal procedure. Bars indicate change in OD415. Error bars indicate range of ΔOD415. Results are the mean of two independent duplicate determinations.
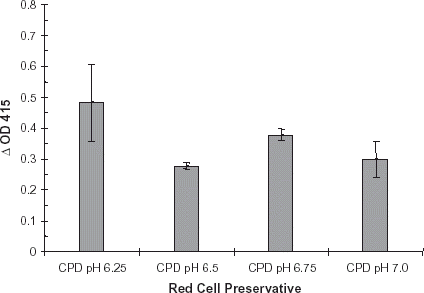
The effect of type B plasma on enzymatic activity was assessed using the ELISA assay. Preservative solution CPD, pH 7.0, was diluted 1:10 with 50 mM NaH2PO4, 100 mM NaCl (PBS), pH 7.0 containing enzyme. This solution was then used to dilute type B plasma to a concentration of 9.2% v/v. Enzymatic activity was also measured in a dilute CPD control and 91.7% v/v type B plasma. Purified R. gnavus α-galactosidase was diluted into each of the plasma solutions and added to B membrane coated microwells. Final plasma concentrations in the microplate wells were 0, 9.2 and 91.7%. Control wells contained similar solutions without enzyme. ELISA plates were incubated for 24 h at 37°C, and developed per normal procedure (see Methods). Based on the results of this study, it was apparent that enzyme retained activity in the presence of B plasma as exhibited by ΔOD415 of 0.383 in a 9.2% B plasma solution. At 0% plasma, the dilute CPD solution containing enzyme, a ΔOD415 of 0.252 was observed, and a 91.7% plasma solution containing enzyme, a ΔOD415 of 0.285 was noted, .
Figure 5. Effect of type B plasma: Red cell preservative solution CPD, pH 7.0, was diluted 1:10 with PBS, pH 7.0. This solution was used to dilute type B plasma to the following concentrations: 0%, 9.2% and 91.7% plasma (see Methods). A purified preparation of enzyme was diluted with each of the plasma solutions. Negative controls consisted of identical plasma dilutions minus enzyme. The ELISA assays were performed per routine. Error bars indicate range of ΔOD415. Results are the mean of two independent duplicate determinations.
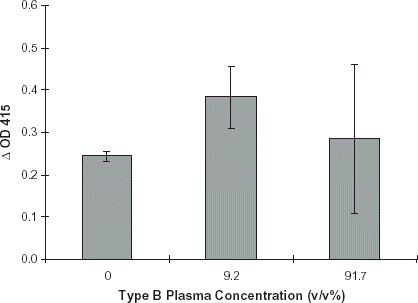
Discussion
Results of these studies confirmed the ability of the R. gnavus α-galactosidase preparations to hydrolyze the terminal galactose from the type B epitope, resulting in a conversion to H epitope (type O antigen). Use of the ELISA assay allowed examination of a wide variety of parameters. Enzymatic conversion of blood type B to O is a promising technology and could significantly alter the practice of transfusion medicine. The expense of enzyme production and man-hours of work involved will be the primary determinants of whether this approach is cost-effective (Lublin, Citation[[2000]]).
The H antigen study demonstrated that R. gnavus α-galactosidase increased expression of the H epitope (type O) after exoglycosidase treatment of type B membranes, . A similar conversion of the type B epitope to type H epitope was also demonstrated by Hobbs et al. with Glycine max α-galactosidase. In this study, detection of seroconversion was accomplished using biotinylated Ulex europaeus lectin (Hobbs et al., Citation[[1996]]) while the current study used monoclonal anti-H.
The pH at which the purified R. gnavus α-galactosidase can effectively cleave the terminal galactose from the type B epitope is important if the enzyme is to be used for seroconversion of red cells in banked units. Other groups have reported successful alteration of the B epitope using α-galactosidases with lower pH optima (Goldstein, Citation[[1984]]; Hobbs et al., Citation[[1996]]; Lenny et al., Citation[[1991]]). However, at these lower pH ranges, perturbations to red cell metabolism may occur (Goldstein et al., Citation[[1982]]). Upon treatment of B membranes with R. gnavus α-galactosidase, maximal ΔOD415 was noted at pH 6.0. It is important to note that at pH 7.0, the ΔOD415 was 77% of the maximum. Overall, it indicated that R. gnavus α-galactosidase exhibited B epitope hydrolytic activity in pH range observed in stored red cell units, pH 6.4–7.2.
The results of the ionic strength study indicated that at pH 7.0 maximal ΔOD415 was noted at 200 mM NaCl. Other purified glycosidases have exhibited similar broad ionic strength optima (Haibach et al., Citation[[1991]]; Hata et al., Citation[[1992]]). The R. gnavus α-galactosidase was active in the ionic strength range found in packed red blood cell units. Variation in buffer concentrations appeared to have had little effect on the overall hydrolysis of the B epitope. Maximal ΔOD415 was achieved in buffers containing 20 mM Na citrate + 120 mM NaCl and 10 mM NaH2PO4 + 140 mM NaCl, both at pH 7.0. Low concentrations of NaCl (<100 mM) appeared to decrease R. gnavus α-galactosidase hydrolytic activity on B membranes.
Red cells are normally collected into preservative solutions resulting in one volume of preservative solution to the eight or nine volumes of whole blood. CPDA-1 solution appeared to enhance R. gnavus α-galactosidase activity as compared to AS-1 and CPD (). Long-term enzymatic stability studies in these preservative solutions could determine the feasibility of collecting red cells directly into blood bags with preservative containing exoglycosidase.
The concentration of plasma in packed red cell units is variable. When whole blood is collected into CPD and CPDA-1, the plasma concentration is reduced by approximately 13%. Because the volume of CPD and CPDA-1 packed red cell units averages 225–250 mL and the hematocrit ranges from 70 to 80%, there is approximately 60 mL of plasma diluted with preservative. In AS-1 units the concentration of plasma is significantly less as the additive further dilutes the plasma. As demonstrated, R. gnavus α-galactosidase was active in concentrated and dilute type B plasma. An enzyme active in concentrated and dilute plasma would be useful for seroconverting erythrocytes in packed red cell units collected in CPD, CPDA-1, or after the addition of AS-1.
This study has demonstrated that an α-galactosidase can be fractionated from Ruminococcus gnavus, which specifically cleaves the terminal galactose from the type B antigenic site on human erythrocyte membranes resulting in the seroconversion of the B antigen to the type H antigen (type O) in some conditions found in packed red blood cell units. A purified exoglycosidase specific for the seroconversion of type B to O erythrocytes would have numerous useful applications. Of immediate importance is the possibility of converting antigenic epitopes of units volumes of red cells. This type of technology would result in the ability to convert erythrocyte type B in order to meet immediate demand for universally transfusable type O red cell units. This would result in an overall increase in the numbers of type O units available for transfusion.
References
- Bradford M. M. A rapid and sensitive method for the quantitation of microgram quantities of protein utilizing the principle of protein-dye binding. Anal. Biochem. 1976; 72: 248–254, [PUBMED], [INFOTRIEVE]
- Dybus S., Aminoff D. Action of α-galactosidase from Clostridium sporogenes and coffee beans on blood group B antigen of erythrocytes. Transfusion 1983; 23: 244–247, [PUBMED], [INFOTRIEVE]
- Goldstein J. Biochemical manipulation of blood groups. Clin. Immunol. Allergy 1984; 4(3)489–502
- Goldstein J. Preparation of Transfusable Red Cells by Enzymatic Conversion. The Red Cell: Sixth Ann Arbor Conference. Alan R. Liss Inc., New York 1984; 139–157
- Goldstein J., Sivglia G., Hurst R., Lenny L., Reich L. Group B erythrocytes enzymatically converted to group O survive normally in A, B, and O recipients. Science 1982; 215: 168–170, [PUBMED], [INFOTRIEVE]
- Haibach F., Hata J., Mitra M., Dhar M., Harmata M., Sun P., Smith D. Purification and characterization of a Coffea canephora α-d-galactosidase enzyme. Biophys. Biochem. Res. Comm. 1991; 181(3)1564–1571, [CROSSREF]
- Hata J., Dhar M., Mitra M., Harmata M., Haibach F., Sun P., Smith D. Purification and characterization of N-acetyl-α-d-galactosaminidase from Gallus domesticus. Biochem. Intl. 1992; 28: 77–86
- Hobbs L., Mitra M., Phillips R., Smith D. The activity of a blood type B specific exoglycosidase from Glycine max. Clinica Chimica Acta 1996; 247: 7–21, [CROSSREF], [CSA]
- Hobbs L., Phillips R., Smith D. An ELISA for blood group specific exoglycosidases. J. Immunol. Meth. 1993; 169: 261–266, [CROSSREF]
- Hoskins L. C. Human enteric population ecology and degradation of gut mucins. Editorial: Digestive Diseases and Sciences 1981; 26(9)769–772
- Hoskins L. C., Agustines M., McKee W. B., Boulding E. T., Kriaris M., Niedermeyer G. Mucin degradation in human colon ecosystems. J. Clin. Invest. 1985; 75: 944–953, [PUBMED], [INFOTRIEVE]
- Hoskins L. C., Boulding E. T. Degradation of blood group antigens in human colon ecosystems: in vitro production of ABH blood group-degrading enzymes by enteric bacteria. J. Clin. Invest. 1976; 57: 63–73, [PUBMED], [INFOTRIEVE]
- KoScielak J. ABH blood group active glycoconjugates from human red cells. Transfusion Medicine 2001; 11(4)267–279, [PUBMED], [INFOTRIEVE], [CROSSREF]
- Kruskall M. S., AuBuchon J. P., Anthony K.Y., Herschel L., Pickard C., Biehl R., Horowitz M., Brambilla D. J., Popovsky M. A. Transfusion to blood group A and O patients of group B RBC's that have been enzymatically converted to group O. Transfusion 2000; 40(11)1290–1298, [PUBMED], [INFOTRIEVE], [CROSSREF], [CSA]
- Larson G., Falk P., Hoskins L. C. Degradation of human intestinal glycosphingolipids by extracellular glycosidases from mucin-degrading bacteria of the human fecal flora. J. Biol. Chem. 1988; 263(22)10790–10798, [PUBMED], [INFOTRIEVE]
- Lenny L. L., Hurst R., Goldstein J., Benjamin L. J., Jones R. L. Single unit transfusions of RBC enzymatically converted from group B to group O in A and O normal volunteers. Blood 1991; 77(6)1383–1388, [PUBMED], [INFOTRIEVE]
- Lublin D. M. Universal RBC's. Transfusion 2000; 40(11)1285–1289, [PUBMED], [INFOTRIEVE], [CROSSREF], [CSA]
- Roseman S. Reflections on glycobiology. J. Biol. Chem. 2001; 276(45)41527–41542, [PUBMED], [INFOTRIEVE], [CROSSREF]
- Schenkel-Brunner H. Biochemical basis of the ABO system subgroups. Wiener Klin. Wschr. 2001; 113(20–21)787–798
- Vosnidou N. C., Johnson S. A., Mitra M., Wells D. C., Li C. Q., Evans M. L., Harmata M. A., Walker J. C., Smith D. S. Seroconversion of type B to O erythrocytes using recombinant Glycine max α-d-galactosidase. Biochem. Mol. Biol. Intl. 1998; 46(1)175–186