Abstract
Brewers yeast was encapsulated in κ-carrageenan microspheres using an emulsification-thermal gelation approach. Due to heat sensitivity of the yeast at temperatures in excess of 36°C, mixtures of low and high gelation temperature carrageenans were tested to obtain a blend yielding a gelation temperature under 40°C. A 20:80 dispersion of 2% carrageenan sol containing cells, in warm canola oil, produced microspheres upon cooling, with a mean diameter of 450 µm and narrow size dispersion (span of 1.2). Application of a chitosan membrane coat to minimize cell release, increased the mean microsphere diameter to 700 µm, due to the coat thickness and swelling of the microspheres. This diameter was designed so as to minimize mass transfer limitations. Batch fermentations were carried out in a 3 L reactor on a commercial wort medium. Cell loading was 107 cells mL−1 microspheres, and cell “burst” release was observed upon inoculation into fresh medium, whether microspheres were coated or not. The kinetics of intra- and extracapsular cell growth were determined. Increased concentrations of extracapsular free cells could be accounted for by growth in the wort medium, and by ongoing release from the gel microspheres, whether coated or not. Cell release from chitosan-coated carrageenan microspheres was less than that from uncoated microspheres, likely due to retention by the membrane coat. Growth kinetics and α-amino nitrogen consumption of encapsulated yeast were higher than that of free cells, and differences in alcohol and ester profiles were also observed, likely due to modified metabolism of the encapsulated yeast.
Introduction
Immobilized cell technology has been widely applied in a variety of research and industrial applications. Cells are physically confined or localized within a defined region, which facilitates separation from the bulk medium and potential reuse in continuous operations. Immobilization also enhances stability of biological materials which are often less sensitive to environmental changes than free cells (Groboillot et al., Citation[[1994]]). For example, immobilized Saccharomyces cerevisiae is more tolerant of ethanol (Krisch and Szajáni, Citation[[1997]]; Norton et al., Citation[[1995]]) and acetic acid (Krisch and Szajáni, Citation[[1997]]), a by-product of ethanol fermentation, in comparison to free cells.
The brewing industry, in an effort to increase productivity, has considered continuous operations with immobilized cell technology in wort fermentation and maturation (Mensour et al., Citation[[1996]]). Enhanced productivity derives from reduced process time, and high cell densities. Increased yeast stability, reduction of contaminant risk, and facilitated recovery and reuse of cells provide additional benefits. Gel entrapment is widely used to immobilize yeast and other cells due to the biocompatibility of polymers such as agar, agarose, gellan gum, alginate or κ-carrageenan, and the ease of formulation. Cell loadings 100 to 1000 times higher than conventional fermentations, result in high bioreactor productivities. Conventional fermentations with free cells require 5–7 days to convert fermentable sugars into ethanol, CO2 and aromatic compounds. Immobilized yeast decreased fermentation times to 1 day, however mass transfer limitations affected the flavour quality of beer because of low amino acid consumption and modified metabolic activity of entrapped cells (Mensour et al., Citation[[1996]]).
Pilkington (Pilkington et al., Citation[[1999]]) investigated viability, cell density and distribution of brewing yeast immobilised in κ-carrageenan beads within a continuous gas-lift rector. They found that viable cells within the bead decreased less than 50% after 6 months of continuous fermentation. The maximum cell concentration within the bead was 109 cells mL−1 of gel bead.
Cell-loaded microspheres with diameters less then 1 mm are preferred as reduced mass transfer limitations within the gel microbeads better promote desirable flavor profiles in the beer. Two techniques are generally used to produce gel beads: droplet extrusion and emulsification. Extrusion of cell-loaded polymer drop wise through one or several needles into a gelation bath, results in beads ranging from one to several millimeters. Concentric airflow applied to the needle enables a reduction in bead size to a range between 300 µm to 1 mm (microspheres). However, the most suitable way to obtain large quantities of microspheres is to emulsify or disperse the aqueous polymeric phase containing cells into an immiscible organic phase, followed by gelation and microbead separation. This technique is readily scalable for industrial use, and formulation conditions are more easily controlled resulting in reproducible batches. Since high retention of cells within the gel is required, an additional level of protection can be provided by membrane coating as the membrane reduces gel porosity and enhances both the mechanical and chemical stability of the gel (Goosen et al., Citation[[1989]]; McKnight et al., Citation[[1988]]).
The objectives of this study were to develop a process for formulating small and uniform diameter gel microspheres loaded with yeast cells, for application in brewing. κ-carrageenan was chosen as the immobilization matrix, for its biocompatibility and easy of use. Microspheres were formulated by an emulsification technique, and fermentations carried out in a 3 L fermentor using a brewing wort under a controlled atmosphere of air and CO2.
Materials and Methods
Microorganism and Culture Conditions
The yeast strain was a commercial dry preparation of Saccharomyces cerevisiae brewing lager strain identified as London. The culture was kindly donated by Lallemand Inc. (Montreal). Yeast was rehydrated in distilled warm water (40°C), plated on Peptone-Yeast extract-Nutrient (PYN) agar medium for yeasts (Difco Laboratories, Detroit, USA) and incubated at 30°C for 36 h to obtain single colonies. Isolated colonies were inoculated to 20 mL PYN broth and incubated overnight at 30°C with agitation (200 rpm). This preculture was used to inoculate (3% v/v) a 400 mL second preculture and incubated for 9 h under the same conditions. Cells were harvested at the end of exponential growth phase, centrifuged at 5000 rpm for 10 min and resuspended in 20 mL physiological solution (0.85% NaCl). The cell suspension was either used directly in fermentation, or warmed to 40°C prior to encapsulation.
Thermoresistance of Yeast
Cells from a second preculture on PYN liquid medium were collected at the end of exponential growth phase, then incubated in a water bath set at different temperatures. Resistance to heat was evaluated by viable cell count on PYN agar plates incubated for 36 h at 30°C.
Gelation Temperature of Carrageenan Gel
Carrageenan solutions were prepared using mixtures of low gelation temperature Genugel X-0909 donated by Copenhagen pectin (Denmark) and commercial grade κ-carrageenan purchased from Sigma (St Louis, USA), yielding 2 or 3% solutions. Solutions were prepared in 0.85% NaCl and heated to 80°C to dissolve the polymer. Temperature of solutions was monitored with a thermocouple during the cool-down process until gelation occurred. The gelation point was established by visual observation, and by physically touching the sol until the gel formed.
Immobilization
Carrageenan solutions (2% w/v) were prepared in 0.85% (w/v) NaCl. Chitosan solution (0.4% w/v) was dissolved in 1% glacial acetic acid, and the pH adjusted to 6.0 with NaOH. Canola oil was a commercial grade product donated by Canada Packers (Montreal, Canada). A volume of 180 mL of 2% (w/v) carrageenan was cooled to 40°C and mixed aseptically with 20 mL of the yeast cell concentrate (108 cells mL−1 gel). This mixture was added to a reactor containing 1 L canola oil at 40°C and stirred for 15 min at 350 rpm, and a controlled temperature of 40°C. The cell-polymer dispersion was cooled to 25°C by addition of 500 mL cold oil (4°C) with continued mixing to initiate gelation of the emulsified droplets. Microspheres were washed several times in sterile 22 g L−1 KCl solution containing 1% tween 80 to remove residual oil. Coating with chitosan was performed by stirring 200 mL beads in 1 L chitosan solution for 1 h. Coated microspheres were rinsed in sterile 22 g L−1 KCl, stored overnight at 4°C, and washed with sterile physiological saline to remove residual KCl, before fermentation. Size analysis was performed using a particle size analyzer (Malvern Instruments, Southborough, USA) to provide the mean diameter and the Span which corresponds to the size band containing 80% of the microspheres divided by the mean size.
Fermentation
Fermentations were carried out at 30°C in a 3 L jacketed reactor (Bioengineering AG, Switzerland). The reactor was agitated at 100 rpm, and sparged with a 2% air in CO2 mixture at a rate of 0.03 vvm. As the reactor was being filled to 2 L with brewing wort, the gas flow rate was adjusted to 60 mL min−1. Wort was prepared from a commercial concentrate (Brewmart Belgian dark lager, Kirkliston, Scotland, UK), at 75 g L−1 concentrate in water, to which 45 g L−1 dextrose was added. The pH was adjusted to 6.5 prior to autoclaving (30 min, 121°C). After 75 h, the fermentation medium was cooled and transferred at 4°C for maturation, allowing beads and cells to settle.
Cell Concentration
Optical density (OD) was measured at 600 nm on the liquid supernatant to quantify cell release, using a Cary 1 UV-Visible spectrophotometer (Varian, Montreal, Canada). OD was converted to colony forming units (cfu mL−1) according to OD = 1.52 × 105 cfu mL−1, determined by prior calibration. The sample was then centrifuged at 4000 rpm for 5 min, and the supernatant frozen. One mL of microspheres was diluted into 9 mL physiological saline, then broken by a 30 s treatment with a tissumizer. A 1 min treatment was required to break the more resistant chitosan coated beads. Dilutions were plated on PYN agar and incubated at 30°C for 36 h. Plate counts were expressed as cfu mL−1.
Analyses
α-amino nitrogen was determined by the ninhydrin test, with lysine as a standard. OD at 570 nm was measured using a Spectramax 250 (Molecular Devices) spectrophotometer. Diacetyl was analyzed colorimetrically with diacetyl as standard (Westerfeld, Citation[[1945]]). Alcohols and esters were determined using an HP5890 series II gas chromatograph (Hewlett Packard, Toronto, Canada) with Carbowax HP20M 0.3 µm, capillary column equipped with a FID detector. Ethanol, acetaldehyde, ethylacetate, methanol, 1-propanol, isobutanol, isoamylacetate, and isoamylalcohol were measured using internal standards, at the end of the fermentation and maturation periods.
Results
Modification of Carrageenan Gel Temperature
Tolerance of London lager yeast to temperatures encountered during encapsulation is illustrated in . With increasing temperature beyond 36°C, viability decreased in a linear fashion, and no cells survived exposure at 53°C. Encapsulation procedures were subsequently carried out at temperatures below 40°C.
In order to reduce the gelation temperature to a level compatible with high viability, different proportions of low gelation temperature carrageenan were mixed with a commercial grade carrageenan, and gelation temperature determined. Results in show that 3% carrageenan solution gels between 48 and 39°C, whereas a 2% solution gels between 40 and 35°C. In both cases, gelation temperatures decrease with increasing amounts of low gelling temperature carrageenan. A mixture of 2% carrageenan was chosen for further studies, with equal proportions of the two types of carrageenan.
Figure 2. Gelation temperature of 2 (•) and 3% (▪) carrageenan solutions with different proportions of low gelation temperature carrageenan.
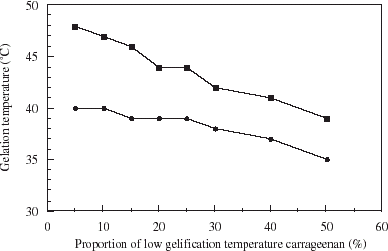
For the dispersion of carrageenan into oil, a water-in-oil phase ratio of 0.2 was chosen to achieve an adequate dispersion of the gel. Higher ratios led to oil droplet inclusions into the gel microspheres.
Microsphere Characteristics
Microsphere size is expressed as a frequency based on the volume of microspheres as seen in . Microsphere diameters exhibited a low size dispersion, with a mean diameter of 450 µm and span of the distribution equal to 1.2. The size distribution for chitosan-coated microspheres was lower than that for non-coated microspheres with a span of 0.9. The mean diameter also increased with coating to 700 µm due to the presence of a membrane coat and possible bead swelling during membrane formation. A diameter of 500 µm has been proposed to be optimal to minimize mass transfer limitations (Kurosawa et al., Citation[[1989]]).
Encapsulated Cell Populations and Growth
The encapsulated cell population was estimated to be 1 × 108 cfu mL−1 of microspheres based on the cell population in the medium prior to encapsulation. The actual encapsulated cell population was determined to be 3 × 107 and 5 × 107 cfu mL−1, for uncoated and coated microspheres respectively, indicating an apparent loss of viable yeast during encapsulation.
Growth of yeast in brewing medium follows a sigmoïdal curve as seen in . The cell doubling time during exponential phase was 3.2 h and the onset of stationary phase occurred after 17 h. The pH decreased from 5.5 to 4.4 in 15 h, remaining unchanged to the end of fermentation. Fermentation with yeast encapsulated within uncoated and chitosan-coated carrageenan microspheres is illustrated in . The cell doubling times were 1.8 and 2.7 h respectively during exponential phase. While cell loadings obtained for free cell culture were two to three times that of encapsulated cell culture, growth rates during the exponential growth phase were higher, though shorter lived. For cells in uncoated microspheres, the pH decreased more rapidly than with free cells, dropping to 4.4 after 10 h. Yeast in chitosan coated beads acidify to pH 4.4 in 12 h, but the initial pH was higher as seen in . In all cases, pH at the end of fermentation stabilized at 4.2.
Figure 5. Growth (squares) and pH profile (circles) during fermentation with yeast encapsulated in uncoated (solid symbols) and chitosan-coated (open symbols) carrageenan microspheres.
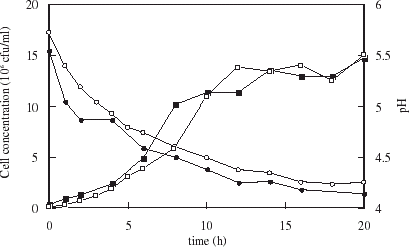
Initially, and possibly during the course of fermentation, cells were released from the microspheres whether membrane coated or not, and appeared to be growing free in the medium. Growth of released cells during encapsulated cell fermentation, is shown in . The initial free cell population was 1 × 105 and 4 × 105 cfu mL−1 for coated and uncoated microspheres respectively, indicating a high level of cell “burst” release at the onset. Initial release from coated microspheres was less than that from uncoated microspheres. The total cell population increased during the fermentation, indicating growth of both free cells as well as those cells encapsulated within the microspheres.
Figure 6. Free cells (circles) and total cell concentration (squares) over the course of fermentation with yeast-loaded uncoated (open symbols) and chitosan-coated (solid symbols) carrageenan microspheres.
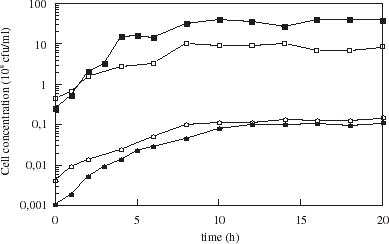
The presence of extracapsular free cells may be accounted for by both free cell growth and continued release of cells over time. For uncoated carrageenan microspheres, initial cell release gave rise to a free cell population of 4 × 105 cfu mL−1. After one doubling time (3.2 h), the free cell concentration would have been 8 × 105 cfu mL−1, assuming no further cell release. The actual free cell population was 20 × 105 cfu mL−1. The difference is a factor of 5, and thus may be accounted for by ongoing release of growing cells from the microspheres. For chitosan-coated beads, the initial free cell concentration was 1 × 105 cfu mL−1. After the first doubling time, the actual free cell population had increased by approximately 9 fold, again too large to be accounted for strictly by free cell growth. It appears that growing cells are being released from the microspheres, whether coated or not, over the course of the fermentation. Release from coated microspheres over the entire process is less than that released from uncoated microspheres, but since the free cell population is initially lower, growth rates in the medium are actually higher, likely due to reduced competition for nutrients. The total cell population in the bioreactor was consistently higher for the coated beads, due to a higher growth rate during exponential growth phase. This would appear to contradict results in , if it were not for the fact that data in the earlier figure did not include the free cell population.
Fermentation Behavior
A decrease of α-amino nitrogen from 100 to 40 mg L−1 was observed during the fermentation as seen in . Small differences were observed between a wort fermented with yeast entrapped in uncoated carrageenan microspheres and those in chitosan-coated microspheres. Nitrogen consumption was observed to be faster with encapsulated yeast than in free cell fermentation. The time to decrease nitrogen content to half the initial level was 5 h with encapsulated cells, whereas fermentations with free yeast required 8 h to reach the same value of 50 mg L−1.
Figure 7. Kinetics of free amino nitrogen uptake in fermentations with free cells (•), and cells entrapped in uncoated (▪) and chitosan-coated carrageenan microspheres (▴).
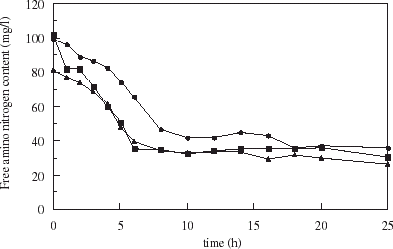
Analysis of alcohols and esters shown in show differences between fermentations involving free cells compared to encapsulated cells in uncoated and chitosan-coated carrageenan beads. Ethanol ranged from 2.8 to 7%, with a decrease observed during maturation for both fermentations with free and chitosan-carrageenan encapsulated cells. Methanol was detected in all cases, with an average concentration of 1.3 g L−1. Propanol was observed in all fermentations in the concentration range observed in typical fermentations (Kronlof et al., Citation[[1989]]; Linko and Kronlof, Citation[[1991]]: 10–20 mg L−1). For fermentations with encapsulated yeast, isobutanol and isoamylacetate levels are around half the amount as with free cells. Isoamylalcohol was detected in all cases. Ethylacetate was not detected, except at the end of maturation for free cell fermentation. Levels obtained were as high as 60 mg L−1. In contrast, concentrations of acetaldehyde were found at high levels for all fermentations.
Table 1. Alcohol and ester content for fermentations with free and carrageenan entrapped cells. Determinations were carried out at the end of fermentation (75 h) and at the end of the maturation process
Discussion
This study describes the encapsulation of viable London yeast in carrageenan and chitosan-coated carrageenan microspheres, formulated by an emulsification/thermal gelation technique. Yeast were active, demonstrating both growth and alcohol formation during fermentation of brewing wort. The formulation methodology yields micron-sized beads, termed “microspheres”, using a dispersion methodology (emulsification) which is well suited to large industrial scale operations such as is encountered in the brewing industry.
London yeast exhibit rapidly declining viability at temperatures in excess of 36°C. As the encapsulation approach involves the use of a thermal set gel, it is important that the yeast are not exposed to higher temperatures during the encapsulation procedure. It was possible to modify the gelation temperature of commercial carrageenan by blending with a low gelation temperature carrageenan. Gelation temperature then depended on the relative proportions of the two carrageenans, and their concentration. Blends containing more than 50% low gelation temperature carrageenan would result in a weak gel, however 2% solutions of carrageenan were able to gel at 37°C, when blended 40–50% with low gelation temperature carrageenan.
The control of the microsphere mean diameter is important for the purpose of minimizing mass transfer limitations, potentially leading to undesirable flavour profiles in the beer (Mensour et al., Citation[[1996]]). A sub-millimeter diameter bead is then preferred, leading to problems in formulation. The emulsification/dispersion approach described in this study has been applied for cell encapsulation systems involving alginate via Ca++ induced internal gelation (Poncelet et al., Citation[[1992]]), and the thermal gelation of carrageenen-locust bean gum (Audet et al., Citation[[1988]]), agarose (Neufeld et al., Citation[[1991]]) and gellan gum (Moslemy et al., Citation[[2002]]). Resulting mean diameter of the carrageenan microspheres formulated in this study was 450 µm with a span of 1.2. The diameter can be controlled by varying the emulsification parameters (Poncelet De Smet et al., Citation[[1989]]), such as the mixing rate or addition of surfactant. A chitosan membrane coat resulted in an increased microsphere diameter. In this case, the diameter increased to 700 µm, which may be due in part to a swelling of the microsphere.
The initial cell loading in the microspheres was approximately 4 × 107 cfu mL−1. Loss of cells into the culture medium was observed through a reduced cell yield (relative to the initial encapsulant cell concentration), and through the measured release of cells from the microspheres during fermentation. Free cell concentrations as high as 4 × 105 cfu mL−1 were measured free in suspension, but were reduced four-fold when microspheres were coated with chitosan. Increase in free cell loading during the fermentation was faster than the growth rate of free cells, suggesting that this increase was due to a combination of free cell growth, and concomitant and on-going release of cells from the microspheres. Thus the microspheres serve as an inoculum to the wort medium, whether microspheres contain a membrane coat or not. Nava Saucedo (Nava Saucedo et al., Citation[[1994]]) showed that high concentrations of free cells may be explained by cell release from the bead surface, promoted by a cell gradient toward the bead periphery. As yeast produced CO2 during fermentation, the gel is subject to deformations and tensions that may weaken the gel and facilitate cell release. Yet cell retention during the course of fermentation was high. The total cell population was 5 times higher for fermentation with chitosan-coated microspheres than for uncoated microspheres. As the final free cell concentration was approximately the same in both cases, it may be concluded that coating is effective to some extent in limiting further cell release. Audet et al., (Citation[[1988]]) reported an increased cell release rate with small beads, and a cell induced weakening of the gel network with carrageenan gels, particularly during the first 2 h of fermentation. It was indicated that gel weakening could be minimized by optimizing the gel composition and entrapment procedure, which may be particularly important when carrageenan blends are used, such as in the present study.
As reported by Nakanishi (Nakanishi et al., Citation[[1986]]), diacetyl formation is closely related to the amount of dissolved oxygen in the wort, with high levels of oxygen conducive to the formation of diacetyl by yeast. In the present study, the wort was sparged with a mixture of CO2 and 2% air according to the protocol outlined by Mensour et al. (Mensour et al., Citation[[1996]]), resulting in minimal dissolved oxygen throughout the fermentation. Under these conditions, levels of diacetyl are minimal, and below the detection limit (data not shown).
The wort used in the present study contained 100 mg L−1 α-amino nitrogen, which is sufficient for growth and flavor development (Krönlof et al., Citation[[1989]]). Levels of 30 mg L−1 were observed at the end of fermentation, indicating no limitation in amino-nitrogen availability. Only total nitrogen consumption was taken into consideration, but metabolic activity may be modified as a result of cell immobilization. Therefore, even if total nitrogen consumption appears normal, amino acid uptake may be unbalanced (Inoue, Citation[[1988]]).
During fermentation, cells convert sugars, amino acids, proteins, and lipids to ethanol, and flavor components including ethylacetate, isoamyacetate, diacetyl, and higher alcohols including propanol, butanol, and isoamyalcohol. Flavor balance in beer is strictly related to the level of yeast development and metabolism. Low levels of isobutanol and isoamylacetate were observed with encapsulated cells, compared to results with free cells. As biosynthesis of higher alcohols is thought to be related to amino acid metabolism and uptake, it may be affected by immobilization, explaining underproduction of higher alcohols. Ester synthesis can be influenced by several factors such as the lipid content, or dissolved oxygen in the wort (Yamauchi et al., Citation[[1995]]). Linko and Kronlöf (Citation[[1991]]) reported that ester formation may be controlled by optimizing oxygen supply in the reactor.
Although cell immobilization may result in the loss of aromas, acetaldehyde and methanol levels were similar to that of the traditional free cell fermentation. As it was expected that mass transfer limitations were largely overcome by the use of small diameter microspheres, encapsulation did have an affect on yeast metabolism, as evidenced through differences in concentration of a number of the flavor and fragrance components tested.
Conclusion
A method of formulating uncoated, and chitosan-coated carrageenan gel microspheres is described, retaining high yeast cell viability through gentle formulation conditions. Immobilization in κ-carrageenan microspheres reduced yeast fermentation time by several hours, due to the high cell loadings in the microspheres, to as high as 109 cfu mL−1. Chitosan coating of carrageenan microspheres reduced cell release, but increases in free cell levels during batch fermentation could only be explained by a combination of cell growth and ongoing cell release from the gel matrix. An unbalanced flavor profile was observed, particularly as high levels of methanol were formed, likely due to a modified metabolic activity of encapsulated yeast.
References
- Audet P., Paquin C., Lacroix C. Immobilized growing lactic acid bacteria with κ-carrageenan-locust bean gum gel. Appl. Microbiol. Biotechnol. 1988; 29: 11–18, [CSA]
- Goosen M. F. A., King G. A., McKnight C. A., Marcotte N. Animal cell culture engineering using alginate polycation microcapsules of controlled membrane molecular weight cut-off. J. Memb. Sci. 1989; 41: 323–343, [CROSSREF]
- Groboillot A., Boadi D. K., Poncelet D., Neufeld R. J. Immobilization of cells for application in the food industry. Crit. Rev. Biotechnol. 1994; 14: 75–107, [PUBMED], [INFOTRIEVE], [CSA]
- Inoue T. Immobilized cell technology-A new possibility for brewing. Amer. Soc. Brewing Chemists J. 1988; 43: 64–66, [CSA]
- Krisch J., Szajáni B. Ethanol and acetic acid tolerance in free and immobilized cells of Saccharomyces cerevisiae and Acetobacter aceti. Biotechnol. Letters 1997; 19: 525–528, [CROSSREF], [CSA]
- Krönlof J., Härkönen T., Hartwall P., Home S., Linko M.. Main fermentation with immobilized yeast. Proceedings of the European Brewery Convention Congress. 1989; 355–362
- Kurosawa H., Matsumura M., Tanaka H. Oxygen diffusivity in gel beads containing viable cells. Biotechnol. Bioeng. 1989; 34: 926–932, [CSA]
- Linko M., Kronlöf J. Main fermentation with immobilized yeasts. Proceedings of the European Brewery Convention Congress. 1991; 353–360
- McKnight C. A., Ku A., Goosen M. F. A., Sun D., Penney C. Synthesis of chitosan-alginate microcapsule membranes. J. Bioactive Biocompat. Polymers 1988; 3: 334–354, [CSA]
- Mensour N. A., Margaritis A., Briens C. L., Pilkington H., Russell I. Application of immobilized yeast cells in the brewing industry. Immobilized Cells: Basics and Applications, R. H. Wijffels, R. M. Buitelaar, C. Bucke, J. Tramper. Elsevier Sci., B.V. 1996; pp. 661–671
- Moslemy P., Guiot S. R., Neufeld R. J. Production of size-controlled gellan gum microbeads encapsulating gasoline-degrading bacteria. Enz. Microb. Technol. 2002; 30: 10–18, [CROSSREF], [CSA]
- Nakanishi K., Onaka T., Inoue T. (1986). A new immobilised yeast reactor system for rapid production of beer. Report of Research Laboratory. Kirin Brewery Company, 29, 13–16
- Nava Saucedo J. E., Audras B., Jan S., Bazinet C. E., Barbotin J.-N. Factors affecting densities, distribution and growth patterns of cells inside immobilization supports. FEMS Microbiology Rev. 1994; 14: 93–98, [CROSSREF], [CSA]
- Neufeld R. J., Peleg Y., Rokem J. S., Pines O., Goldberg I. L-Malic acid formation by immobilized Saccaromyces cerevisiae amplified for fumarase. Enz. Microb. Technol. 1991; 13: 991–996, [CROSSREF]
- Norton S., Watson K., Amore T. D. Ethanol tolerance of immobilized brewers’ yeast cells. Appl. Microbiol. Biotechnol. 1995; 43: 18–24, [PUBMED], [INFOTRIEVE], [CROSSREF], [CSA]
- Pilkington H., Margaritis A., Mensour N., Sobczak J., Hancock I., Russell I. Kappa-carrageenan gel immobilisation of lager brewing yeast. J. Inst. Brew. 1999; 105: 398–403
- Poncelet D., Lencki R., Beaulieu C., Halle J. P., Neufeld R. J., Fournier A. Production of alginate beads by emulsification/internal gelation. I. Methodology. Appl. Microbiol. Biotechnol. 1992; 38: 39–45, [PUBMED], [INFOTRIEVE], [CROSSREF], [CSA]
- Poncelet De Smet B., Poncelet D., Neufeld R. J. Control of mean diameter and size distribution during formulation of microcapsules with cellulose nitrate membranes. Enz. Microb. Technol. 1989; 11: 29–37, [CROSSREF], [CSA]
- Westerfeld W. W. A colorimetric determination of blood acetoin. J. Biol. Chem. 1945; 161: 495–502
- Yamauchi Y., Okamoto T., Murayama H., Nagara A., Kashihara T., Yoshida M., Nakanishi K. Rapid fermentation of beer using an immobilized yeast multistage reactor system: balance control of extract and amino acid uptake. Appl. Biochem. Biotechnol. 1995; 53: 245–259, [CSA]