Abstract
Alginate is a polymer of guluronic acid and mannuronic acid residues and is an inexpensive, nontoxic polysaccharide of marine origin. Trypsin was immobilized noncovalently on alginate with 100% retention of activity. The enzyme did not leach off the polymer even in the presence of 0.01 M HCl and Triton X-100 (0.2% vv−1). The Vmax/Km values did not change significantly on immobilization. There was 22% loss of activity in first cycle of pH change and after that the conjugate could be reused upto 4 precipitation cycles without any further loss of activity. This smart bioconjugate was also found to have better operational stability in the presence of casein than free enzyme. Fluorescence studies were carried out to probe structural changes upon immobilization.
1. Introduction
Conventional immobilization of enzymes or proteins on solid supports is generally carried out by covalent coupling to the matrix rather than adsorption (Blanco and Guisan, Citation[[1989]]; Gupta and Mattiasson, Citation[[1992]]; Williams and Blanch, Citation[[1994]]). This preference is based upon (quite often justified) the premise that an adsorbed enzyme eventually leaks off the support during prolonged use and recycling (Williams and Blanch, Citation[[1994]]). However, in a large number of cases, adsorption has worked well (Gupta and Mattiasson, Citation[[1992]]; Sardar et al., Citation[[1997]]; Vaidya et al., Citation[[1987]]). Whenever it gives acceptable level of stability for the immobilized enzyme, it is a preferable technique since it is economical and generally gives a more active biocatalyst (Gupta and Mattiasson, Citation[[1992]]).
In recent years, quite a few bioconjugates of proteins/enzymes on smart polymers have been described (Arsaratnam et al., Citation[[2000]]; Cong et al., Citation[[1995]]; Fujii and Taniguchi, Citation[[1991]]; Sardar et al., Citation[[2000]]; Taniguchi et al., Citation[[1992]]). A number of these have considerable applications in biomedical sciences (e.g., design of sensors, actuators and valves) (Hoffmann, Citation[[2000]]). These reusable soluble–insoluble biocatalysts are especially valuable for bioconversion of insoluble substrates like cellulose (Taniguchi et al., Citation[[1992]]) or macromolecular substrates like proteins (Fujimora et al., Citation[[1987]]). In the case of such substrates, enzymes immobilized on solid supports are quite inefficient in view of the mass transfer constraints. This had led to the use of water soluble polymers as immobilization supports for designing biocatalysts for such substrates (Van Leemputten and Horisberger, Citation[[1976]]). However such biocatalysts were not easily reusable since their recovery after the reaction was not conveniently possible. In this respect, smart polymers which are reversibly soluble insoluble constitute ideal immobilization matrix (Roy et al., Citation[[2003]]). The catalysis can be done in free solution and the biocatalyst recovered for reuse by precipitation.
Most of the smart biocatalysts described so far have been prepared by covalent coupling (Dominguez et al., Citation[[1988]]; Fujimora et al., Citation[[1987]]; Nguyen and Luong, Citation[[1989]]; Roy et al., Citation[[2003]]; Van Leemputten and Horisberger, Citation[[1976]]). An exception is a recently described immobilization of xylanase on Eudragit in which adsorption has worked rather well (Sardar et al., Citation[[2000]]). In the present work, we describe the immobilization of trypsin on alginate by adsorption. Alginate is a polymer of guluronic acid and mannuronic acid residues and is an inexpensive, nontoxic polysaccharide of marine origin (Smidsrod and Skjak-Braek, Citation[[1990]]). It is a reversibly soluble insoluble polymer which precipitates in the presence of Ca++ and can be dissolved back in the presence of EDTA (Gupta et al., Citation[[1993]]). A large number of attempts for immobilization of trypsin on solid supports have been reported as such (Blanco and Guisan, Citation[[1989]]; Petro et al., Citation[[1996]]). Reusable bioconjugates are extremely useful for protein hydrolysis (Fujimora et al., Citation[[1987]]) and peptide synthesis (Sears and Clark, Citation[[1993]]). Many biomedical applications of trypsin have also been reported e.g., to remove dead tissues from wounds, burns, ulcers etc., so as to speed the growth of new tissue and to inhibit the growth of some contaminant organisms, in emergency kit for snake bite etc. (Cheetham, Citation[[1995]]; Xiong et al., Citation[[1997]]).
2. Materials and Methods
2.1. Materials
Alginate (low viscosity sodium salt, Cat. No. A2158), trypsin (from bovine pancreas, Type III) and haemoglobin were purchased from Sigma Chemical Co., St. Louis, MO, USA. Casein was supplied by Sisco Research Laboratory, Mumbai, India. All other chemicals used were of analytical grade.
2.2. Methods
2.2.1. Measurement of Enzyme Activity
Trypsin activity was measured using casein and urea denatured-haemoglobin as substrates. The activity with casein and haemoglobin was estimated by following the increase in the amount of TCA-soluble peptides (Fujimora et al., Citation[[1987]]). The amount of TCA-soluble peptides was measured by Lowry's method (Lowry et al., Citation[[1951]]). Since the polymer bound enzyme was soluble under assay conditions, the activity of immobilized preparation was estimated in the same way as above.
2.2.2. Estimation of Protein
Protein content was estimated by measuring the absorbance at 280 nm (Stoscheck, Citation[[1990]]).
2.2.3. Preparation of Alginate Solution
Alginate (2%, wv−1) was dissolved in distilled water (Gupta et al., Citation[[1993]]) the pH of which had been adjusted to 7.0.
2.2.4. Immobilization of Trypsin on Alginate
Varying volumes of trypsin (stock solution of 180 µg mL−1) were added to 0.25 mL of alginate (2%, wv−1) and the final volume was made up to 5.0 mL using morpholinoethane-sulphonic acid (MES) buffer (0.05 M, pH 7.0). After incubation for 30 min at 25°C, the polymer-bound enzyme was precipitated by lowering the pH below 2.0 with 1 N HCl followed by centrifugation of the suspension after 10 min (10,000 g× 10 min). The immobilized enzyme was washed with 0.01 M HCl and Triton X-100 (0.2% vv−1). The precipitate was dissolved in 1 mL of MES buffer (0.05 M, pH 7.0). The yield of enzyme activity was calculated in terms of expected and observed activities ().
Figure 1. Calculation of effectiveness factor for the immobilized enzyme using casein (X) and hemoglobin (▵) as substrates. The effectiveness factor (BA−1) was calculated in terms of expected (A) and expressed activities (B). The expected activity is the difference of total initial activity of trypsin in solution form and the activity in supernatant after precipitation of immobilized enzyme. The activity observed after dissolving the immobilized enzyme is plotted as expressed activity.
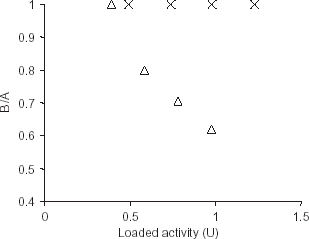
2.2.5. Determination of Km and Vmax
Km and Vmax values of free and immobilized trypsin were determined by measurement of enzyme activity at various concentrations of casein using Leonara software programme (Cornish-Bowden, Citation[[1995]]). This software uses the Hanes-Woolf equation to calculate the kinetic parameters ().
Figure 2. Determination of kinetic constants for the free (○) and immobilized enzyme (•) using casein as substrate. Hanes-Woolf plots for the determination of Km values for free and immobilized trypsin were plotted by measurement of enzyme activity with various concentration of substrate (casein) at 37°C.
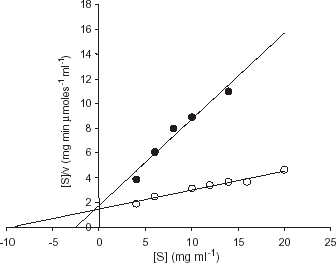
2.2.6. pH Cycling
The stability of immobilized trypsin towards pH cycling was assessed by lowering the pH to 1.8 to precipitate out the immobilized trypsin and redissolving in 0.05 M MES buffer pH 7.0. An appropriate aliquot of the dissolved precipitate was used to measure the enzyme activity. The step was repeated for subsequent precipitation cycles. Free trypsin was treated in a similar manner and concentration of protein was the same as in the immobilized preparation. ()
Figure 3. Effect of pH cycling on the activity of enzyme. The activity of the immobilized preparation (•) was assessed by lowering the pH to precipitate the immobilized enzyme, dissolving it again by increasing the pH and assaying it. The initial activities of both free enzyme as well as immobilized enzyme were taken as 100%. Free enzyme (○) was also passed through same pH changes. This cycle was repeated 3 times.
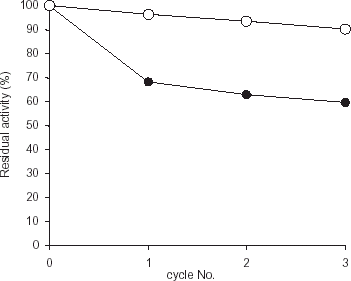
2.2.7. Operational Stability of the Immobilized Enzyme
The operational stability of the immobilized preparation was assessed at 25°C by carrying out the hydrolysis of casein and monitoring the amount of product liberated after each one hour cycle (Lowry et al., Citation[[1951]]). By changing the pH of the reaction mixture to 1.8, both the residual substrate and the immobilized enzyme were precipitated and then separated from the product by centrifugation at 10,000 g for 10 min. The pH of the supernatant was adjusted to 7.0 and the amount of product released was estimated as before (Lowry et al., Citation[[1951]]). Immobilized enzyme and residual substrate were dissolved again in fresh buffer by increasing the pH to initiate hydrolysis reaction. The same cycle was repeated again and again ().
Figure 4. Operational stability of the enzyme preparation. The amount of product released in the supernatant was determined at one hour intervals after removing immobilized enzyme (•) and casein by lowering the pH followed by centrifugation. The activity of the immobilized enzyme was again assessed by adding fresh buffer to the immobilized enzyme and residual casein. The reusability of the free enzyme (○) was assayed in the same way.
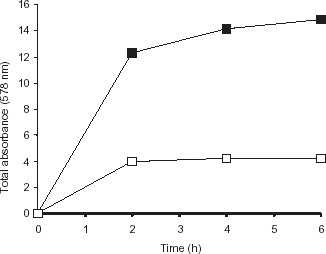
2.2.8. Fluorescence Spectroscopy
The fluorescence emisson spectra were recorded on a Shimadzu RF-5000 spectrofluorometer with a band width of 5 nm and 1 cm path length. The emission spectra were recorded using an excitation wavelength of 284 nm. The concentrations of protein in both cases are matched. ().
Figure 5. Fluorescence spectra of immobilized trypsin. Emission spectrum was recorded on Shimadzu RF-5000 spectrofluorometer with a band width of 5 nm and 1 cm path length (at an excitation wavelength of 284 nm) for free and immobilized preparation. The amount of protein was same in both the cases. (a) Immobilized trypsin (b) Free trypsin. Control ruled out the quenching activity of the polymer.
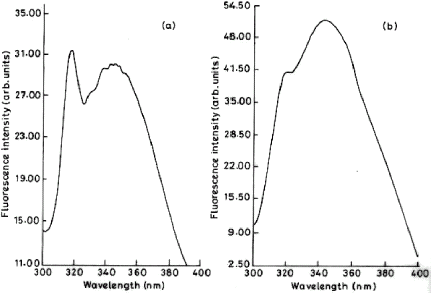
3. Results and Discussion
Protein immobilization on reversibly soluble insoluble polymers is an emerging alternative to the more conventional method involving solid supports for use in clinical applications (Roy et al., Citation[[2003]]). Recently, Arasaratnam et al. (Citation[[2000]]) have shown that trypsin linked to a reversibly soluble insoluble methacrylate polymer is more accessible in free solution to soybean trypsin inhibitor as compared to when the trypsin bioconjugate exists in the insoluble form. This experimental evidence supports the expected and widely held notion that active sites of enzymes linked to soluble matrices are more accessible to substrates/substrate analogs. (Arsaratnam et al., Citation[[2000]]; Roy et al., Citation[[2003]]; Sardar et al., Citation[[2000]]). In the above case (Arsaratnam et al., Citation[[2000]]), immobilization of trypsin was carried out by covalent coupling to the water soluble polymer.
In fact, trypsin was one of the early enzymes to be immobilized on a water soluble polymer (Van Leemputten and Horisberger, Citation[[1976]]). This early work did not make it clear whether conjugation of the enzymes with acrolein-acrylic acid copolymer matrix was via covalent coupling or mere adsorption. In any case, this bioconjugate showed poor proteolytic activity and led the workers to conclude, “The application of soluble-insoluble enzyme complexes with charged polymers may be limited by the charge of the substrate.” If true, this is a serious constraint in the design of smart biocatalysts since most of the reversibly soluble-insoluble polymers carry substantial amount of charges (Arsaratnam et al., Citation[[2000]]; Gupta et al., Citation[[1993]]).
Our results, obtained with a bioconjugate prepared by adsorption of trypsin on alginate (a highly reversibly charged polymer), show that such bioconjugates, even for proteolytic purposes, can be quite effective. It was found that alginate-trypsin bioconjugate showed full activity of the adsorbed enzyme with casein as substrate (). On the other hand, the expressed activity of the adsorbed enzyme molecules was much lower in the case of haemoglobin as the substrate when the bioconjugate with higher amounts of adsorbed trypsin was used. As the pI of haemoglobin is around 7.0 (Righetti et al., Citation[[1981]]), its electrostatic repulsion by alginate is unlikely to be the cause of poor proteolytic activity. The reasons for this significant difference in the proteolytic activities towards the two proteins are likely to be more complex. , however also shows that at the lowest load of trypsin activity, the “effectiveness factor” (ratio of expressed activity of the bioconjugate to the activity expected of the enzyme after immobilization) was equally good for haemoglobin.
It is this immobilized preparation, i.e., with an effectiveness factor of 1 for both substrates, which was characterized further. Further work was limited to working with casein as the substrate.
shows the Hanes-Woolf plots for calculation of kinetic constants. The Vmax/Km values of free and immobilized trypsin were calculated using Leonara software programme (Cornish-Bowden, Citation[[1995]]) and were found to be 0.57 µmol mL min−1 mg−1 and 0.67 µmol mL min−1 mg−1 for casein respectively. These values are in agreement with the effectiveness factor of 1.0 which was obtained for the immobilized enzyme.
The reusability of the preparation was assessed by lowering the pH to 1.8 and assaying it. shows that after the initial loss of 22% activity in the first cycle, the activity does not change up to 4 precipitation cycles. It is interesting to note that the free enzyme, in fact, does not lose any significant amount of activity during the pH recycling. The initial loss in the first cycle is thus, not due to enzyme inactivation. In fact, it was observed that at the precipitation stage after first use, 30% of the bound protein was found in the supernatant. These protein molecules, presumably, were loosely held and came off the matrix during precipitation.
shows the data concerning hydrolysis of casein. The greater hydrolysis of casein by immobilized enzyme reflects the enhanced stability of the enzyme preparation under the reaction conditions. Thus, at the end of 6 h, five times product was obtained with immobilized enzyme as compared to free enzyme.
The use of soluble matrix allows one to probe the structural changes in the enzyme upon immobilization by spectral methods. shows the fluorescence emission spectra of free and immobilized enzymes. The fluorescence emission spectrum of free trypsin showed a broad λmax at 340 nm and a shoulder around 318 nm. This spectrum was obtained by excitation at 284 nm. As pointed out by Schmid (Citation[[1985]]), this allows one to observe emission of both tyrosine and tryptophan residues. The corresponding emission spectrum of the immobilized enzyme showed some interesting changes. The tryptophan λmax shifted by only 4 nm indicating that tryptophan environment has not changed drastically. However, a prominent λmax at 318 nm was now observed. This kind of splitting of λmax region in the emission spectra is unusual but not without precedent. It has been reported for zinc-substituted hemoglobins (Sudhakar et al., Citation[[1998]]). More relevent, Ibrahim et al. (Citation[[1985]]) report that epoxide hydrolase, after immobilization on dextran (another soluble matrix), showed two maxima around 340–358 nm. The contribution of tyrosine residues towards fluorescence spectra of trypsin has been extensively discussed by Turoverov and Kuznetsova (Citation[[1986]]). According to them, absence of tyrosine component in emission spectra is mostly due to the high effeciency of energy transfer from tyrosine residues to tryptophan. It looks likely that immobilization of the enzyme on alginate had resulted in interference with the energy transfer and the immobilized enzyme showed emission due to both tyrosine and tryptophan.
To conclude, the smart bioconjugate of trypsin discussed here is an efficient catalyst for caseinolytic activity. Also, use of soluble matrix made it possible to initiate understanding of structural changes which enzymes undergo when linked to polymeric matrices.
Acknowledgment
This work was supported by project funds from Department of Science and Technology, Council of Scientific and Industrial Research (CSIR) (Technology mission on oil seeds, pulses & maize and Extramural Divison), all Govt. of India organizations. The financial support provided by CSIR to SJ in the form of senior research fellowship is acknowledged.
References
- Arsaratnam V., Galaev I. Y., Matiasson B. Reversibly soluble biocatalyst: optimization of trypsin coupling to Eudragit S-100 and biocatalyst activity in soluble and precipitated forms. Enzyme Microb. Technol. 2000; 27: 254–263, [CSA]
- Blanco R. M., Guisan J. M. Stabilization of enzyme by multipoint covalent attachment to agarose- aldehydes gels: borohydride reduction of trypsin-agarose derivatives. Enzyme Microb. Technol. 1989; 11: 360–366, [CROSSREF], [CSA]
- Cheetham P. S. J. The application of enzymes in industry. Handbook of Enzyme Biotechnology. 3rd ed., A. Wisemann. Ellis Horwood Ltd., London 1995; pp. 419–552
- Cong L., Kaul R., Dissing U., Mattiasson B. A model study on Eudragit and polyethyleneimine as soluble carriers of α-amylase for repeated hydrolysis of starch. J. Biotechnol. 1995; 42: 75–84, [CROSSREF]
- Cornish-Bowden A. Analysis of Enzyme Kinetic Data. Oxford University Press, Oxford 1995; 27–36
- Dominguez E., Nilsson M., Hahn-Hagerdal B. Carbodiimide coupling of β-galactosidase from Aspergillus orygae alginate. Enzyme Microb. Technol. 1988; 10: 606–610, [CROSSREF], [CSA]
- Fujii M., Taniguchi M. Application of reversibly soluble insoluble polymers in bioprocessing. Trends Biotechnol. 1991; 9: 191–196, [PUBMED], [INFOTRIEVE], [CROSSREF]
- Fujimora M., Mori T., Tosa T. Preparation and properties of soluble–insoluble immobilized proteases. Biotechnol. Bioeng. 1987; 29: 747–752, [CSA]
- Gupta M. N., Guoqiang D., Mattiasson B. Purification of endopolygalacturonase by affinity precipitation using alginate. Biotechnol. Appl. Biochem. 1993; 18: 321–327, [PUBMED], [INFOTRIEVE], [CSA]
- Gupta M. N., Mattiasson B. Unique applications of immobilized proteins in bioanalytical systems. Bioanalytical Applications of Enzymes, C. H. Suelter. John Wiley and Sons, Inc., New York 1992; 36: 1–35
- Hoffmann A. S. Bioconjugates of intelligent polymers and recognition properties for use in diagnostics and affinity separations. Clin. Chem. 2000; 46: 1478–1486, [CSA]
- Ibrahim M., Hubert P., Dellacherie E. Covalent attachment of epoxide hydrolase to dextran. Enzyme Microb. Technol. 1985; 7: 66–72, [CROSSREF]
- Lowry O. H., Rosebrough N. J., Fall A. C, Randall R. J. Protein measurement with the Folin phenol reagent. J. Biol. Chem. 1951; 193: 265–275, [PUBMED], [INFOTRIEVE]
- Nguyen A. L., Luong J. H. T. Synthesis and applications of water soluble reactive polymers for purification and immobilization of biomolecules. Biotechnol. Bioeng. 1989; 34: 1186–1190
- Petro M., Svec F., Frechet J. M. J. Immobilization of trypsin onto “molded” macroporous poly (glycidyl methacrylate-co-ethylene dimethacrylate) rods and use of the conjugates as bioreactors and for affinity chromatography. Biotechnol. Bioeng. 1996; 49: 355–363, [CSA]
- Righetti P. G., Tudor G., Kristina E. Isoelectric points and molecular weights of proteins: a new table. J. Chromatogr. 1981; 220: 115–194, [CROSSREF]
- Roy I., Sharma S., Gupta M. N. Smart biocatalysts: design and application. Adv. Biochem. Eng. Biotechnol. 2003, Accepted for publication
- Sardar M., Agarwal R., Kumar A., Gupta M. N. Noncovalent immobilization of enzymes on an enteric polymer Eudragit S-100. Enzyme Microb. Technol. 1997; 20: 361–367, [CROSSREF], [CSA]
- Sardar M., Roy I., Gupta M. N. Simultaneous purification and immobilisation of Aspergillus niger xylanase on the reversibly soluble insoluble polymer Eudragit L-100. Enzyme Microb. Technol. 2000; 27: 672–679, [PUBMED], [INFOTRIEVE], [CROSSREF], [CSA]
- Schmid F. X. Spectral methods of characterizing protein conformation and conformational changes. Protein Structure: A Practical Approach, T. E. Creighton. IRL Press, Oxford 1985; 251–285
- Sears P. S., Clark D. S. Comparison of soluble and immobilized trypsin kinetics: Implications for peptide synthesis. Biotechnol. Bioeng. 1993; 42: 118–124, [CSA]
- Smidsrod C., Skjak-Braek G. Alginate as immobilization matrix for cells. Trends Biotechnol. 1990; 8: 71–78, [PUBMED], [INFOTRIEVE], [CROSSREF]
- Stoscheck C. M. Quantitation of protein. Methods in Enzymology: Guide to Protein Purification, M. P. Deutscher. Academic Press, Inc., San Diego 1990; Vol. 182: 50–67
- Sudhakar K., Laberge M., Tsuneshige A., Vanderkooi J. M. Zinc-substituted hemoglobins: α- and β-chain differences monitored by high resolution emission spectroscopy. Biochemistry 1998; 37: 7177–7184, [PUBMED], [INFOTRIEVE], [CROSSREF]
- Taniguchi M., Kobayashi M., Fujii M. Production of soluble sugars from cellulose material by repeated use of a reversibly soluble autoprecipitating cellulase. Biotechnol. Bioeng. 1992; 39: 287–292, [CSA]
- Turoverov K. K., Kuznetsova I. M. What causes the depolarisation of trypsin and trypsinogen fluorescence: intramolecular mobility or nonradiative energy transfer?. Biophys. Chem. 1986; 25: 315–323, [PUBMED], [INFOTRIEVE], [CROSSREF]
- Vaidya S., Srivastava R., Gupta M. N. Immobilization of acid phosphatase from Arachis hypogaea on CM cellulose. Biotechnol. Bioeng. 1987; 24: 1040–1043
- Van Leemputten E., Horisberger M. Soluble–insoluble complex of trypsin immobilized on acrolein–acrylic acid copolymer. Biotechnol. Bioeng. 1976; 28: 587–590
- Williams R. A., Blanch H. W. Covalent immobilization of protein monolayers for biosensor applications. Biosensors Bioelectron. 1994; 9: 159–167, [CROSSREF], [CSA]
- Xiong Y., Wang W., Lee W., Gao R., Zhang Y. Study and clinical applications of “Emergency kit for snakebites.”. Toxicon 1997; 35: 481–481, [CROSSREF]