Abstract
Enzyme electrodes for the determination of organophosphate pesticides were developed by using acetylcholinesterase (AChE) in combination with a pH electrode. AChE was immobilized on the surface of pH electrode by using gelatin and chitosan membranes, respectively. The measurement system is based on potentiometric detection of the inhibiting properties of organophosphates on enzyme activity. The phosphate buffer (2.5 mM, pH 8.0) and the borate buffer (2.5 mM, pH 8.5) at 25°C were established as providing the optimum conditions for the gelatin and chitosan based biosensor systems, respectively. Furthermore, operational, thermal, and organic phase stabilities were also tested. Linear ranges for different organophosphates such as malathion, parathion-methyl, and methamidophos were detected by using both types of biosensor system. Moreover, as well as accuracy, the regeneration conditions by using pyridine-2-aldoximethiodide (PAM-2) as a reactivating agent for the inhibited electrodes were also investigated.
Introduction
Increasing use of pesticides all-over the world has generated a growing interest for pesticide environmental monitoring. The organochlorine insecticides (DDT, aldrin, and lindane) used in the past has been progressively replaced with organophosphate compounds. These compounds show environmental persistence lower than the organochlorines but have a higher acute toxicity which can be a serious problem for the equilibrium of aquatic ecosystems (Evtugyn et al., Citation[[1997]]; Mulchandani et al., Citation[[2001]]; Rekha et al., Citation[[2000]]). Another problem is food contamination which could have a serious impact on human health. Moreover, the toxicity of these compounds has a direct impact on farmers and workers in pesticide manufacturing industries. The analysis of pesticides is generally carried out using gas chromatographic or HPLC methods (Farid, Citation[[2001]]; Hoff and Zoonen, Citation[[1999]]; Maye and Miles, Citation[[1988]]) which identify single compounds in complex mixtures and reach detection limits compatible with those imposed by law for pesticide residues in the environment. However, these methods generally require sample pretreatment and preconcentration steps and are not very suitable for field analysis (Arkhypova et al., Citation[[2001]]; Ivanov et al., Citation[[2000]]). Biosensors based on the inhibition of cholinesterases are useful for this purpose. One of the resulting products of the enzymatic hydrolysis of acetylcholine chloride is H+ and it can be easily detected by a pH meter. The decrease in the concentration of the enzymatic reaction product caused by enzyme inhibition is related to pesticide concentration (Hendji et al., Citation[[1993]]; Ristori et al., Citation[[1996]]; Rogers et al., Citation[[1991]]; Skladal, Citation[[1996]]).
The inhibition of immobilized enzyme is almost permanent. After the inhibition reaction, the resulting enzyme-phosphorus bond cannot be broken by water, but partial recovery of the activity can be obtained by using nucleophilic reagents such as oximes (Forget et al., Citation[[2002]]; Okazaki et al., Citation[[2000]]).
In this study, our goal was to develop simple biosensor system based on acetylcholinesterase immobilized with two different membrane systems on the surface of pH-electrode for the potentiometric detection of organophosphate pesticides.
Materials and Methods
Chemicals
Acetylcholinesterase (AChE), (EC.3.1.1.7) from electric eel (450 U mg−1), 225 bloom calf skin gelatin, glutaraldehyde, and the regenerating agent; pyridine-2-aldoximide methiodide (PAM-2), acetylcholine chloride (AChCl) were obtained from Sigma Chem. Co., St. Louis, USA. Other reagents were analytical-reagent grade.
Apparatus
The potential measurements were performed in magnetically stirred cell (35 mL), maintained at 25°C with a thermostat (WEB MLW, Germany). Biomembrane coated glass electrode was linked to digital pH meter (Hanna, Portugual).
Electrode Preparation
Gelatin based AChE electrode was prepared as follows; AChE (30 IU) and gelatin (66 mg/mL) in 50 mM potassium phosphate buffer (pH 7.0) were mixed at 38°C and then, 0.1 mL of this mixture was deposited on the sensitive area of the pH electrode by deep coating method. The average amount of enzyme was around 3 U. The sensor was placed in glutaraldehyde solution (2.5% in 50 mM phosphate buffer, pH 7.5) for 5 min to achieve complete polymerization of the bio-membrane and subsequently washed in a stream of distilled water (Ertaş et al., Citation[[2000]]).
Chitosan based AChE electrode was prepared as follows; AChE (30 U) and 100 µL of chitosan-acetate solution (1%) were mixed and then covered on the pH electrode as the same procedure given for gelatin based AChE electrode then, crosslinked with tripolyphosphate solution (2%, pH 8.2) which is counter ion for chitosan, for 30 min and subsequently washed with distilled water (Zihnioğlu and Telefoncu, Citation[[1995]]), and stored at 4°C until used.
Measurement of Enzyme Activity, Inhibition, and Reactivation
All measurements were carried out in phosphate buffer (2.5 mM, pH 8.0) for gelatin based system and borate buffer (2.5 mM, pH 8.5) for chitosan based system at 25°C by using a thermostatic cell. The sensitive part of the sensor was immersed in the stirred buffer solution and appropriate amount of stock AChCl solution in buffer was added to obtain the required final AChCl concentration. A change in proton concentration produced by the enzymatic reaction at the glass-electrode surface and the corresponding change in the output voltage is considered to be a measure of the enzymatic membrane activity.
For the pesticide analysis, the measurement procedure involved the following steps; the response for the saturated substrate concentration was recorded and used as a reference, the sensor was washed and dipped in pesticide solution in the buffer (50 mL) with stirring until 60 min and then, the excess of the pesticide solution was removed with a buffer stream and the enzymatic activity was determined as described above. The percentage of the residual activity was calculated by direct comparison with initial activity.
In order to reactivate the inhibited enzyme, the sensor was immersed for 60 min in a stirred reactivation solution (0.1 mM PAM-2), (Imato and Ishibashi, Citation[[1995]] and Ivanov et al., Citation[[2000]]). Nevertheless, the reactivated membranes were not used again to ensure uniform initial conditions in the inhibition study.
Results and Discussion
Enzyme Electrode Optimization and Characterization
Optimum pH
Some preliminary experiments were performed to establish the optimum pH and buffer concentration to obtain maximum sensor response. The electrode response can be changed substantially by varying the buffer concentration and pH. Maximum electrode response was obtained at 2.5 mM phosphate buffer, pH 8.0 for gelatin based AChE electrode and at 2.5 mM borate buffer, pH 8.5 for chitosan based enzyme electrode (a,b). As is seen in b, optimum pH was shifted because of the ionic character of chitosan membrane (Wang et al., Citation[[2002]]; Zihnioğlu and Telefoncu, Citation[[1995]]). On the other hand, when phosphate buffer was tested with chitosan based AChE system, lower responses were obtained because of the effect of phosphate ions to the chitosan structure. Moreover, to increase the electrode response, the effect of buffer concentration was examined over the range of 2.0–10 and 2.5 mM was chosen for the experiments.
Optimum Temperature
The effect of temperature on the electrode response was tested in the range of 15–42°C. Maximum response was found to be 25°C for both gelatin and chitosan based AChE electrode (a,b).
Effect of Substrate Concentration
The effect of substrate (AChCl) concentration on the electrode response was tested. It is reported that the choice of an appropriate substrate concentration is important in enzyme inhibition studies. The percentage inhibition was evaluated from the response of the active and inhibited forms of the enzyme. Hence, all the enzymes have to take part in the reaction that corresponds to the saturation part of the calibration graph (response of the biosensor vs. substrate concentration). The saturation of the enzymatic membrane with the substrates contributes to the most sensitive determination of the inhibiting effect (Hendji et al., Citation[[1993]]). For this reason, the saturation concentrations of AChCl were chosen from graphs for each biosensor design. The effect of AChCl concentration on the biosensor responses were given in a,b and 0.28 and 1.42 mM of AChCl were used in inhibition and regeneration studies as saturated substrate concentrations for gelatin and chitosan based electrodes, respectively.
Thermal and Operational Stabilities
The stabilities of enzyme electrodes were assessed by its operational and thermal efficiency at 25 and 37°C, respectively for both systems. No decrease was obtained with gelatin based system during 6 h at mentioned temperatures. However, for the chitosan based AChE electrode, sensor responses were found to be decreased up to 91 and 81% at 25 and 37°C, respectively ().
Organic Phase Stabilities
The influence of organic solvents with different hydrophobicities on the stability of electrode was tested. It was performed in a two-step method; incubation of enzyme electrode in organic medium and measurement of the remaining activity in working buffer solution.
The effects of the organic solvents were investigated to estimate the remaining activity (%) after solvent contact for 30 and 60 min, respectively (Tables and ). Our results showed that the hydrophobicity of organic solvents plays a major role in determining the stability and catalytic activity of cholinesterase as reported in previous works (Montesinos et al., Citation[[2001]]). The inactivation observed in water-miscible organic solvents (methanol, ethanol, acetone etc.) was probably caused by a reduction in amount of water bound to the enzyme. In contrast, nonpolar organic solvents (hexane, cyclohexane, iso-octane etc.) seemed to have little effect on the structure of the enzyme. This low denaturation could be correlated to the rigidity of the enzyme caused by a reduction in flexibility in organic solvents (Campanella et al., Citation[[2001]]). On the other hand, chitosan and gelatin based enzyme electrodes have different stability towards the same solvents used in experiments. The immobilization may have effected the conformation, stability, and activity of enzymes as reported in previous works (Fennouh et al., Citation[[1997]]). In organic media, the amount and activity of the water retained by the support material are also important for the stability and activity. The immobilized AChE electrode in gelatin matrix found to be more stable after incubation in organic solvents. Furthermore, surprisingly high activity was observed in some organic solvents (cyclohexane, toluene) as reported for subtilisin and porcine pancreatic lipase. A possible explanation for this observation is that these enzymes retain their hydration shell strongly and the membrane system used for the immobilization contributed the stability increase (Skadal and Krejci, Citation[[1996]]).
Table 1. Effects of organic solvents on chitosan based AChE electrode (incubation time; 30 and 60 min)
Table 2. Effects of organic solvents on gelatin based AChE electrode (incubation time; 30 and 60 min)
Enzyme Inhibition Study and Pesticide Determination
The dependence of enzyme inhibition on the pesticide concentration (malathion, parathion-methyl, and methamidophos) was investigated up to 60 min of incubation time. The percentage inhibition of the enzyme electrode is not directly proportional to the incubation time (data is not shown). The inhibition curves when plotted against logarithm of pesticide concentration, the percentage inhibition was found to be proportional to the logarithm of pesticide concentration ( and ).
Figure 5. Inhibition curves of malathion (a), parathion-methyl (b), and methamidophos (c) for gelatin based AChE electrode.
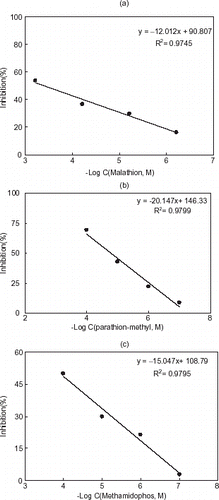
Figure 6. Inhibition curves of malathion (a), parathion-methyl (b), and methamidophos (c) for chitosan based AChE electrode.
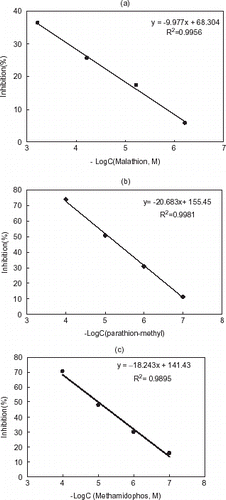
According to the inhibition curves, the determination of pesticides by using both systems was possible in the concentration range of 0.1 to 100 µM for parathion-methyl and methamidophos and 0.6 to 600 µM for malathion. These results showed that the proposed sensors can be used to detect organophosphates without preconcentration step in both aqueous and organic medium. Furthermore, these systems are portable and no special skills are required to use them.
Accuracy
The reproducibility of biosensors for the pesticide detection was also tested. Standard deviations and variation coefficients are given in .
Table 3. Reproducibility of gelatin and chitosan based AChE biosensors
Regeneration of Inhibited Enzyme
Regeneration of the inhibited enzyme after treatment of different amount of pesticides was performed by using pyridine aldoximethiodine (PAM-2) as a reactivating reagent (Tables and ). As is shown in tables activities were completely recovered in the cases of lower inhibition levels. When higher pesticide concentrations were used partial enzyme regeneration was achieved for both types of biosensors.
Table 4. Regeneration of chitosan based AChE electrode inhibited by various organophosphates; malathion, parathion-methyl, and methamidophos (PAM-2; 0.1 mM in 2.5 mM sodium borate buffer (pH 8.5), 60 min)
Table 5. Regeneration of gelatin based AChE electrode inhibited by various organophosphates (malathion, parathion-methyl, and methamidophos). PAM-2; 0.1 mM in 2.5 mM sodium borate buffer (pH 8.5), 60 min)
Acknowledgment
This work was supported by Ege University Research Fund through Project 98 Fen 009.
References
- Arkhypova V. N., Dzyadevych S. V., Soldatkin A. P., El'skaya A. V., Jaffrezic-Renault N., Jaffrezic H., Martelet C. Multibiosensor based on enzyme inhibition analysis for determination different toxic substances. Talanta 2001; 55: 919–927
- Campanella L., Favero G., Persi L., Sammartino M. P., Tomasetti M., Visco G. Organic phase enzyme electrodes: applications and theoretical studies. Anal. Chim. Acta 2001; 426: 235–247, [CROSSREF]
- Ertaş N., Timur S., Akyılmaz E., Dinçkaya E. Specific determination of hydrogen peroxide a catalase biosensor based on mercury thin film electrodes. Turk. J. Chem. 2000; 24: 95–99
- Evtugyn G. A., Rizaeva E. P., Stoikova E. E., Latipova V. Z., Budnikov H. C. The application of cholinesterase potantiometric biosensor for preliminary screening of the toxicity of waste waters. Electroanalysis 1997; 9: 1124–1128
- Farid E. A. Analyses of pesticides and their metabolites in foods and drinks. TrAC Trends in Analytical Chemistry 2001; 20: 649–661, [CROSSREF]
- Fennouh S., Casimiri V., Burstein V. Increased paraoxon detection with solvents using acetylcholinesterase inactivation measured with a choline oxidase biosensor. Biosens. Bioelectron. 1997; 12: 97–104, [CROSSREF]
- Forget J., Livet S., Leboulenger F. Partial purification and characterization of acelylcholinestresterase (AChE) from the estuarine copepod Eurytemora affinis (Poppe). Comparative Biochemistry and Physiology Part C: Toxicology of Pharmacology 2002; 132: 85–92, [CROSSREF]
- Hendji A. M. N., Jaffrezic-Renault N., Martelet C., Clechet P. Sensitive detection of pesticides using a differential ISFET-baset system with immobilized cholinesterase Anal. Chim. Acta 1993; 281: 3–11, [CROSSREF]
- Hoff G. R., Zoonen P. Trace analysis of pesticides by gas chromatography. J. Chrom A. 1999; 843: 301–322, [CROSSREF]
- Imato T., Ishibashi N. Potentiometric butyrylcholine sensor for organophosphorus pesticides. Biosens. Bioelectron. 1995; 10: 455–441, [CROSSREF]
- Ivanov A. N., Evtugyn G. A., Gyurosanyi R. E., Toth K., Budnikov H. C. Comparative investigation of electrochemical cholinesterase biosensors for pesticide determination. Anal. Chim. Acta 2000; 404: 55–65, [CROSSREF]
- Maye A. H., Miles C. J. Postcolumn photolysis of pesticides for fluorometric determination by HPLC. Anal. Chem. 1988; 60: 220–226
- Montesinos T., Perez-Munguia S., Valdez F., Marty J. L. Disposable cholinesterase biosensor for the detection of pesticides in water-miscible organic solvents. Anal. Chim. Acta 2001; 431: 231–237, [CROSSREF]
- Mulchandani A., Chen W., Mulchandani P., Wang J., Rogers K. Biosensors for direct determination of orgonoshosphate pesticides. Biosens. Bioelectron. 2001; 16: 225–230, [PUBMED], [INFOTRIEVE], [CROSSREF]
- Okazaki S., Nakagawa H., Fukuda K., Asakura S., Kiuchi H., Shigemori T., Takahashi S. Reactivation of an amperometric organophosphate pesticide biosensor by 2-pyridine aldoxime methochloride. Sensors and Actuators B. 2000; 66: 131–134, [CROSSREF]
- Rekha K., Thakur M. S., Karanth N. G. Biosensors for the detection of organophosphorous pesticides. Critical Reviews in Biotechnology 2000; 20: 213–235, [PUBMED], [INFOTRIEVE]
- Ristori C., Del Carlo C., Martini M., Barbaro A., Ancarani A. Potentiometric detection of pesticides in water samples. Anal. Chim. Acta 1996; 325: 151–160, [CROSSREF]
- Rogers K. R., Cao C. J., Valdes J. J., Eldefrawi A. T., Eldefrawi M. E. Acetylcholinesterase fiber-optic biosensor for detection of anticholinesterases. Fundam. Appl. Toxicol. 1991; 16: 810–820, [PUBMED], [INFOTRIEVE], [CROSSREF]
- Skladal P. Biosensors based on cholinesterase for detection of pesticides. Food Technol. Biotechnol. 1996; 34: 43–49
- Skadal P., Krejci J. Performance of amperometric biosensor with immobilized butyrylcholinesterase in organic solvents. Collect. Czech. Chem. Commun. 1996; 61: 985–991, [CROSSREF]
- Wang G., Xu J. J., Ye L. H., Zhu J. J., Chen H. Y. Highly sensitive sensors based on the immobilization of tyrosinase in chitosan. Bioelectrochemistry 2002; 57: 33–38, [PUBMED], [INFOTRIEVE], [CROSSREF]
- Zihnioğlu F., Telefoncu A. Diffusion characterizatic of chitosan entrapped microsomal UDP-Glucuronyltransferase Gel Beads. Biochimica Biophysica Acta 1995; 1244: 291–294