Abstract
Phanerochaete chrysosporium BKMF-1767 cells were immobilized on different carriers. The optimum carrier according to adsorbed P. chrysosporium cells number (86.38%) was determined to be polystyrene foam, a novel carrier. The conditions for the immobilization of cells on polystyrene foam were optimized and determined as 50 rpm, 37°C, and 2 h. The results show that the adsorption of P. chrysosporium on polystyrene foam follows the Langmuir adsorption isotherm. High manganese peroxidase activity (421 U/L) and dry mass (4.7 g/L) were recovered from the batch mode polystyrene foam solid state fermentation system.
Introduction
The white rot fungus Phanerochaete chrysosporium efficiently degrades lignin as well as a broad spectrum of xenobiotic compounds. The fungus produces an extracellular lignin-degrading enzyme system, consisting of lignin peroxidase (LiP) and manganese peroxidase (MnP) (Tien and Kirk, [Citation1983]). They are synthesized during secondary metabolism in response to nitrogen, carbon, or sulphur limitation (Dosoretz and Grethlein, [Citation1991]; Faison and Kirk, [Citation1985]). MnP and LiP are H2O2-dependent enzymes. MnP is known to oxidize Mn (II) to Mn (III), which acts as a mediator (Wariishi et al., [Citation1992]).
The growth mechanisms of P. chrysosporium in liquid cultures range from dispersed mycelia to compact pellets. The formation of the pellets from a spore inoculum has been investigated in agitated liquid culture (Gerin et al., [Citation1994]). Dispersed mycelia are only significant in static cultures (Prosser and Tough, [Citation1991]).
The phenomenon of microbial cell adhesion by adsorption is almost universal. Advantages of the adsorption method are the simplicity of operation, the mild conditions employed, the possibility of high cell concentration and the minimization of cell build-up and sloughing (Phillips and Poon, [Citation1988]). Various systems have been tested for immobilizing P. chrysosporium, such as inclusion in gel beads, immobilization on plastic disk, in polyurethane foam, and porous plastic material, and silicone tubes, nylon web, sintered glass, and porous ceramic carrier (Asther et al., [Citation1989]; Capdevila et al., [Citation1989]; Linko and Zhong, [Citation1987]). The use of nutrionally inert materials as supports for solid state fermentation (SSF) facilitates accurate designing of media, monitoring of process parameters, scaling-up strategies and various engineering aspects, which are either impossible or difficult with conventional SSF using organic solid substrates such as wheat bran (Zhu et al., [Citation1994]). The inert materials, when impregnated with a suitable medium, will provide a homogeneous aerobic condition throughout the fermenter, will not contribute impurities to the fermentation product and will facilitate maximal recovery of the leachate with low viscosity and high activity for the target product (Pandey, [Citation1992]).
The aim of the present study was to select an adequate support for the immobilization of P. chrysosporium as well as to determine the best immobilization conditions in order to obtain high MnP activity. This enzyme is very important for biotechnological applications. The kinetic constants of P. chrysosporium adsorption on polystyrene foam beads were also estimated.
Materials and Methods
Microorganism and Growth Medium
P. chrysosporium BKM-F-1767 (ATCC 24725) was grown on a medium prepared according to Tien and Kirk ([Citation1988]) with 10 g glucose/L as carbon source, except dimethylsuccinate was replaced by 20 mM acetate buffer (pH 4.5) (Tien and Kirk, [Citation1988]).
P. chrysosporium Immobilization on Different Carriers and Culture Conditions
Carriers (1 g) such as Amberlite IR 45, Eupergite C, Eupergite C 250 L, Perlit (<70 mesh), Purolite CT 275, Purolite MN 500, polypropylene, Coco-peat (<35 mesh), Coco-peat (>35 mesh), polystyrene foam (diameter 3–4 mm) and rough bran were placed in 100 mL Erlenmeyer flasks and then autoclaved at 121°C for 20 min. Spores of 6 days old were harvested in sterile water, filtered through glass-wool and diluted to 0.5 at OD650. Then 5 mL of this spore suspension (5 × 106 spor/mL) was added. The immobilization (adsorption of cells on support materials) was carried out for about 2 h on a shaker with a controlled speed (50 rpm) at 37°C. The number of adsorbed cells of P. chrysosporium was estimated by determining the difference of the cell titers before and after immobilization.
Manganese peroxidase enzyme production was achieved in 100 mL flasks with growth medium containing inductors 174 µM Mn2+, 0.05% (v/v) Tween 80 (unpublished data). The Erlenmeyer flasks were loosely capped with cellulose stoppers, which permitted a passive aeration, and incubated statically under an air atmosphere at 37°C and 90% humidity to avoid evaporation.
Analytical Methods
Manganese(II)-dependent peroxidase activity was assayed spectrophotometrically by the method of Kuwahara et al. ([Citation1984]). One activity unit was defined as the amount of enzyme that oxidized 1 µmol of dimethoxyphenol per minute and the activities were expressed in U/L.
Dry weight was measured daily by filtering cultures and drying at 105°C.
Results and Discussion
After the incubation at 50 rpm and 37°C for 2 h, the amount of adsorbed cells of P. chrysosporium (%) was estimated ().
Obtained values from show that the best carrier for P. chrysosporium cells was polystyrene foam. The use of polystyrene foam that is expanded polystyrene granules as carrier for immobilization of P. chrysosporium cells has not been reported so far. To the best of our knowledge this study is the first report on immobilization using polystyrene foam by any white rot fungi.
Table 1. Adsorbed P. chrysosporium spores (%) on various carriers
Previous reports on the immobilization of P. chrysosporium are contradictory. According to Asther et al. ([Citation1989]) the amount of immobilized mycelium is higher for hydrophobic (polypropylene, polyurethane) than for hydrophilic carriers (stainless steel and grey) (Asther et al., [Citation1989]). Jones and Bredis ([Citation1992]) found that biomass adhesion is correlated to the surface energy of the carriers, with greater adhesion observed on materials of higher surface energy, that is to say materials with higher degree of hydrophilicity (Jones and Bredis, [Citation1992]). Guimaraes et al. ([Citation2002]) found no correlation between surface hydrophobicity or hydrophilicity of tested solid carriers, poly(vinylchloride), stainless steel, polyurethan foam and Scotch-Brite, and the amount of immobilized P. chrysosporium (Guimaraes et al., [Citation2002]). They also reported that morphology of the carriers was important and governed the amount of immobilized mycelium and especially the fungal biodegradation activity. From , hydrophobicity is more effective than hydrophilicity for the adhesion of P. chrysosporium on carriers as in the case of macro porous ion exchange resins.
Polystyrene is a vinyl polymer. Structurally, it is a hydrocarbon chain, with a phenyl group attached to every other carbon atom. Polystyrene is produced by free radical vinyl polymerization, from the monomer styrene ().
Expanded polystyrene (EPS) is a generic term for polystyrene and styrene copolymers that are expanded into a variety of useful products. EPS is supplied to mold in the form of a polystyrene bead (Hendrick et al., [Citation2001]).
The Effect of Shake Speed on the Adsorption of P. chrysosporium Cells
Immobilization of P. chrysosporium on polystyrene foam was achieved at 37°C and various shake speeds for 2 h ().
Table 2. The effect of shake speed on adsorbed P. chrysosporium amount (%)
The adsorbed cell number (%) was decreased by increasing shake speeds. The optimum shake speed was determined as 50 rpm.
Gerin et al. ([Citation1994]), as in this study, concluded that adhesion of P. chrysosporium spores requires specific conditions, such as surface treatment of the carrier, high ionic strength, and absence of liquid motion. It was also shown that spore-spore aggregation is detrimental for spore–carrier adhesion (Gerin et al., [Citation1994]).
The Effect of Incubation on Time on the Adsorption of P. chrysosporium Cells
In order to measure the quantity of immobilized cells attached to the surface of the carrier, 1 g polystyrene foam beads and 5 mL cell suspension with known cell titer were added to flasks. The flasks were shaken at 37°C with 50 rpm. At different intervals of elapsed time, each flask was taken out of the shaker; the quantity of cells in suspension was determined spectrophotometrically. Obtained values were plotted against time ().
As shown in , the quantity of immobilized cells attached to the surface of carriers depends on immobilization time. After about 2 h, adsorption equilibrium was reached between free and immobilized cells.
The Effect of Initial Cell Quantity on the Adsorption
The influence of initial cell quantity on the adsorption was investigated. For this reason, 1 g of polystyrene foam beads and 5 mL of spore suspensions containing different number of cells were added to a set of flasks. The flasks were shaken at 37°C with 50 rpm for 2 h. The quantity of cells in the suspension was determined spectrophotometrically at 650 nm ().
shows that the extent of cell adsorption increased with increasing initial cell density in the spore solution. The specific cell loading (number of cells adsorbed/g carrier) was shown to give a first-order dependence on the cell concentration in the residual suspension. The desired cell loading can be obtained by varying the initial concentration cell in the spore suspension.
From the experimental results given in , it is possible to describe adsorption kinetics using a model obtained from diffusion kinetics. If it is assumed that adsorption takes place in a semi-infinitive medium and that cell concentration at the interface follows a law of type, where Co, initial cell concentration; C, cell concentration after a time t; K, a constant. The following mathematical model is obtained:
where Xa, quantity of cells adsorbed of a time; X1, maximum quantity of cells adsorbed; ko, a constant (may be assimilated with an initial adsorption rate constant); k1, a constant (variation of adsorption rate with time).
The validity of this model may be tested by varying (Xa/X1) (1/t) as a function of 1/. The values of the constants ko and k1 may thus be estimated (Chen and Skidmore, [Citation1987]).
The constant k1 is negative. This means that the adsorption rate decreases with time, and the higher the initial rate, the more rapidly the adsorption rate decreases. By analogy with Langmuir's adsorption theory, microbial cell adsorption at equilibrium may be described by the following model:
where Xa, quantity of cells adsorbed; X1, maximum quantity of cells adsorbed; s, quantity of free cells in spore suspension; ka, microorganism/support affinity constant. The validity of this model has been examined by plotting 1/Xa as a function of 1/Xs, using the experimental data of ().
The values of X1 and ka may thus determine from .
The results show an excellent correlation between the experimental values and mathematical description. The experimental results in this study show that the adsorption of P. chrysosporium on polystyrene foam yields results, which follow the Langmuir isotherm.
Production of MnP from Free and Immobilized P. chrysosporium
The production of MnP and biomass by P. chrysosporium was also investigated in free and immobilized cultures (a–b).
As it shown in a–b, the maximum MnP and bio mass production was achieved on the 6th day. The enzyme activity was increased from 355 UL−1 to 421 UL−1 for free and immobilized cultures. The dry mass was increased from 3.1 g L−1 to 4.7 g L−1 for free and immobilized cultures. Immobilization was shown to be the most efficient process for the production of MnP by this fungus.
Figure 6. Production MnP (-▪-) and dry mass (-•-) from free (a) and immobilized (b) P. chrysosporium cells.
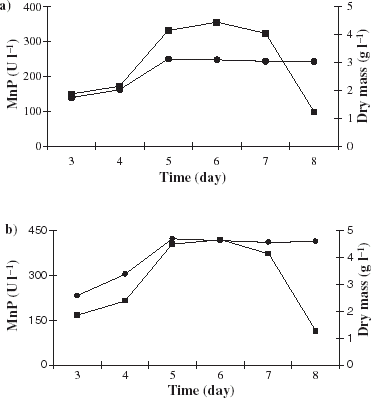
Growth of immobilized cells can be viewed as an alternative to growth of free cells in many instances. In others, immobilization confers a precision of control over the process not possible in free growth. Immobilization of cells can sometimes be considered to be a lower–cost alternative to immobilization of enzymes (Phillips and Poon, [Citation1988]).
The white rot fungus P. chrysosporium has delignifying potential. Ligninolytic microbial systems have been used in improving the digestibility and nutritive value of animal feeds, degradation of toxic pollutants, bioconversion of lignin into useful organic compounds (Tien and Kirk, [Citation1983]; Zadrazil, [Citation1980]).
References
- Asther M., Bellon-Fontaine M. N., Capdevila C., Corrieu G. A thermodynamic model to predict Phanerochaete chrysosporium INA-12 adhesion to various solid carriers in relation to lignin peroxidase production. Biotechnol. Bioeng. 1989; 35: 477–482
- Capdevila C., Corrieu G., Asther M. A feed-harvest culturing method to improve lignin peroxidase production by Phanerochaete chrysosporium INA-12 immobilized on polyurethane foam. J. Ferment. Bioeng. 1989; 68: 60–63
- Chen C. Y., Skidmore D. R. Langmuir adsorption isotherm for sulfolobus acidocaldorius on coal particles. Biotechnol. Lett. 1987; 9: 191–194
- Dosoretz C. G., Grethlein H. E. Physiological aspects of the regulation of extracellular enzymes of Phanerochaete chrysosporium. Appl. Biochem. Biotechnol. 1991; 28: 253–265
- Faison B. D., Kirk T. K. Factors involved in the regulation of a ligninase activity in Phanerochaete chrysosporium. Appl. Environ. Microbiol. 1985; 52: 251–254
- Gerin P. A., Asther M., Sleytr U. B., Rouxhet P. G. Detection of rodlets in the outer wall region of conidiospores of Phanerochaete chrysosporium. Can. J. Microbiol. 1994; 40: 412–416
- Guimaraes C., Matos C., Azeredo J., Mota M., Oliviera R. The importance of the morphology and hydrophobicity of different carriers on the immobilization and sugar refinery effluent degradation activity of Phanerochaete chrysosporium. Biotechnol. Lett. 2002; 24: 795–800
- Hendrick V., Muniz E., Geuskens G., Werenne J. Adhesion, growth and detachment of cells on modified polystyrene surface. Cytotechnology 2001; 36: 49–53
- Jones S. C., Bredis D. M. Adhesion and lignin peroxidase production by the white rot fungus Phanerochaete chrysosporium in a rotating biological contactor. J. Biotechnol. 1992; 24: 277–290
- Kuwahara M., Glenn J. K., Morgan M. A., Gold M. H. Separation and characterization of two extracellular H2O2-dependent oxidases from ligninolytic cultures of Phanerochaete chrysosporium. FEBS Lett. 1984; 169: 247–250
- Linko S., Zhong L. C. Comparison of different methods of immobilization for lignin peroxidase production by Phanerochaete chrysosporium. Biotechnol. 1987; 1: 251–256
- Pandey A. Recent process developments in solid-state fermentation. Process Biochem. 1992; 27: 109–117
- Phillips C. R., Poon Y. C. Immobilization of Cells. Springer-Verlag, Berlin Heidelberg 1988; pp. 1–73
- Prosser J. I., Tough A. J. Growth mechanisms and growth kinetics of filamentous microorganisms. Crit. Rev. Biotechnol. 1991; 10: 253–274
- Tien M., Kirk T. K. Lignin degrading enzyme from the hymenomycete Phanerochaete chrysosporium. Science 1983; 221: 661–663
- Tien M., Kirk T. K. Lignin peroxidase of Phanerochaete chrysosporium. Methods Enzymol. 1988; 16: 238–249
- Wariishi H., Valli K., Gold M. H. Manganese(II) oxidation by manganese peroxidase from the basidiomycete Phanerochaete chrysosporium: kinetic mechanism and role of chelators. J. Biol. Chem. 1992; 267: 23688–23695
- Zadrazil F. Conversion of different plant into feed by basidiomycetes. Eur. J. Appl. Microbiol. 1980; 9: 243–248
- Zhu Y., Smits J. O., Knol W., Bol J. A novel solid state fermentation system using polyurethane foam as inert carrier. Biotechnol. Lett. 1994; 16: 643–648