Abstract
Clinical and experimental evidence has indicated that chronic renal failure (CRF) is related to increased free radical production. CRF patients show increased lipid peroxidation after a progressive reduction in vitamin E, one of the most important antioxidants. In the present study the role of vitamin E deficiency in lipid peroxidation was investigated in rats submitted to subtotal nephrectomy. Male Wistar rats were divided into groups receiving different diets for a period of 45 days: SND – sham rats submitted to a regular diet containing vitamin E; ERD – nephrectomized rats submitted to a regular diet containing vitamin E; SDD – sham rats submitted to a vitamin E-deficient diet; EDD – nephrectomized rats submitted to a vitamin E-deficient diet. After 30 days the Experimental animals were submitted to 5/6 nephrectomy and the Controls were submitted to sham operation. The vitamin E levels of the SDD and EDD groups were significantly reduced (p<0.05) in plasma (4.92 ± 1.22 and 8.37 ± 2.09 mmol/L, respectively), liver (7.57 ± 2.72 and 9.44 ± 2.55 mg/g tissue, respectively) and kidney (8.17 ± 2.38 and 9.40 ± 3.10 mg/g tissue, respectively) when compared to the SRD and ERD groups. In contrast, in the EDD group the levels of thiobarbituric acid-reactive substances, expressed as nmol/mg protein, were significantly increased (p<0.05) in the liver (1.41 ± 0.27) and kidney (1.67 ± 0.47), and superoxide dismutase activity was significantly increased in the erythrocytes (4455.80 ± 1322.63 Ug/Hb) compared to all other groups. The vitamin E-deficient diet associated with subtotal nephrectomy determined an increase in lipid peroxidation, suggesting an important role of free radicals in the development of chronic renal failure.
INTRODUCTION
Experimental evidence has indicated that reactive oxygen species (ROS) play an intermediary role in the physiopathological processes of a wide variety of experimental and clinical diseases.Citation[[1]] ROS react with polyunsaturated fatty acids present on the cell membrane, with consequent lipid peroxidation.Citation[[2]]
The defense mechanism that protects cells against the toxic effects of radicals includes Mn, Cu, Zn, Se, vitamins E and C, and the enzymes superoxide dismutase (SOD), catalase and glutathione peroxidase.Citation[[2]] The major antioxidant action of vitamin E on biological membranes is primarily to inhibit the stage of propagation of lipid peroxidation.Citation[[2]], Citation[[3]]
Phospholipid peroxidation, accompanied by increased malondialdehyde (MDA) concentrations, causes an increased rigidity and deformity of erythrocyte membranes, which in turn increase susceptibility to hemolysis.Citation[[4]] According to several investigators, the presence of these factors contributes to the onset of anemia in renal patients.Citation[[5]] Increased MDA can be explained by the oxidative stress to which the erythrocytes are submitted.Citation[[6]] In addition, it has been demonstrated that rats fed a vitamin E- and selenium-deficient diet develop tubulointerstitial nephritis after a period of 14 weeks.Citation[[7]] Tubulointerstitial nephritis is considered to be one of the most common causes of end stage renal failure.Citation[[7]]
A significant increase in plasma and urinary MDA has been demonstratedCitation[[8]] in patients with different degrees of renal dysfunction. This increase is accompanied by a significant decrease in vitamin E and catalase and glutathione peroxidase levels in the erythrocytes of these patients.
Machlin and BendichCitation[[9]] stated that the balance between the production of free radicals and defense antioxidants may be lost due to overproduction of free radicals, exposure to sources that saturate he defense antioxidants, or to inadequate ingestion of nutrients that contribute to the defense system of the organism.
In view of the above considerations, in the present study we investigated the hypothesis that lipid peroxidation is increased in rats submitted to chronic renal insufficiency due to a greater production of free radicals and/or to deficiency of antioxidant defenses induced by ingestion of a vitamin E-deficient diet.
MATERIAL AND METHODS
Animals
We studied 96 male Wistar rats with an initial mean weight of 115.50 ± 3.48 g. The rats belonging to the Experimental Groups were submitted to 5/6 nephrectomy performed by the technique of pole ligation of Platt et al.Citation[[10]] The remaining animals were submitted to sham operationCitation[[11]] and were used as the Control Group.
After surgery, the animals were returned to individual stainless steel cages and kept in a closed room at a temperature of approximately 22°C, with a 10 h light cycle.
Experimental Protocol
The 96 animals were divided into four groups: Sham receiving a diet with normal vitamin E levels (SDN); Experimental receiving a diet with normal vitamin E levels (EDN); Sham receiving a vitamin E-deficient diet (SDD); and Experimental receiving a vitamin E-deficient diet (EDD). All animals were studied for a period of 45 days. During the first 30 days, each group was fed its respective diet. On the 30th day of study (T0), Groups SDN and SDD were submitted to sham surgery and Groups EDN and EDD were submitted to subtotal nephrectomy. After surgery, all animals continued to receive their respective diets for 15 days, after which they were sacrificed (T2). On the day of sacrifice (T1 and T2), eight animals per group were picked at random and submitted to a 12 h fast, after which blood, urine and tissue samples were obtained for laboratory determinations. Plasma urea and creatinine were determined on T1 and T2. Twenty-four hour urinary albumin, glomerular filtration rate (GFR), vitamin E, thiobarbituric acid-reactive substances (TBARS), and SOD were determined only at T2. All animals received the diet recommended by the AOAC,Citation[[12]] containing 12 protein (12 g/100 g diet). The normal experimental and control Groups received 1000 mg/100 g mixture of 50% vitamin E, whereas the experimental and control deficient Groups received no vitamin E in the diet. All animals received diet and water ad libitum.
Analytical
Creatinine, MDA and SOD were determined on the day of animal sacrifice. The blood, urine and tissue samples used for the determination of 24 h urinary albumin, GFR and vitamin E were stored frozen at −20°C or −70°C, and processed within a maximum of one week after collection. Blood was collected into heparinized tubes and liver and kidney samples were immediately frozen in liquid nitrogen. All samples were obtained in the morning after an overnight fast.
Blood, kidney and liver samples were immediately prepared for the determination of plasma urea and creatinine and of plasma, liver and kidney MDA, as well as SOD activity, as described below. Plasma was separated from the blood samples for the determination of urea and creatinine using a kit from LAB-TEST Sistema para Diagnóstico S.A., with readings taken in a spectrophotometer (Spectronic 601-Milton Roy) at 520 nm and 510 nm wavelength, respectively.
MDA concentrations in liver and kidney were determined by measuring TBARS by the method of Uchiyama and Mihara.Citation[[13]] The absorbance of the product was measured with the same spectrophotometer at 535 nm wavelength. TBARS concentration was measured considering the coefficient of molar absorptivity of the product (E535 = 1.56 × 10−5 M−1 cm−1).Citation[[13]] A standard curve was constructed using a stock solution of 10 mM MDA prepared from tetrametoxypropane (Sigma), showing good linearity. The results are reported as nmol/mg protein and protein concentration was determined by the method of Lowry et al.Citation[[14]]
After plasma removal, the erythrocyte sediment was separated and lysed in 1.5 mL ultrapure water and 12 mL of a 3 : 5 mixture of chloroform/ ethanol (v/v) was added. The preparation was then centrifuged at 3000 rpm at 4°C for 10 min and the supernatant was removed and used to determine SOD activity.Citation[[15]] Erythrocyte SOD activity was determined in a reaction mixture containing 1 µmol hydroxylamine, 60 µg xanthine oxidase, 1 mM hypoxanthine, 65 µmol alphanaphthylamine, 1 mM KCN, 5 µg naphthylenediamine, and 300 µg/mL sulfanilic acid in phosphate–borate buffer (20.8 mM/15.6 mM, pH 8.2). The absorbance of the product formed was measured spectrophotometrically at 550 nm. SOD activity was calculated considering that 1 unit SOD inhibits 50% of the formation of the reaction product at 37°C. The samples were read in duplicate and the results are expressed as U/g Hb. Hemoglobin concentration was determined in parallelCitation[[16]] in the same erythrocyte sample in order to express the results as SOD units per g hemoglobin using the same visible UV light spectrophotometer at 540 nm.
Twenty-four hour urinary albumin determined by electroimmunoassay (EIE) as proposed by Schmid et al.Citation[[17]] using agarose gel containing specific antiserum GFR was determined on the basis of inulin clearance.Citation[[18]] The animals were anesthetized with thionembutal (50 mg/kg), and tracheostomized, and the femoral artery and vein were cannulated for the collection of blood samples and for fluid infusion. The ureters were cannulated for urine collection. A priming inulin dose of 12.0 mg/100 g body weight was injected into each animal, followed by a maintenance inulin dose of 0.5 mg/min/100 g delivered with an infusion pump at a flow of 2.5 mL/h. After a 1 h period of equilibration, urine samples were removed and plasma samples were obtained at the midpoint of each urine collection period. The intensity of urine and plasma color was read with a Micronal B 382 spectrophotometer at 620 nm wavelength. Inulin clearance (GFR) was calculated using a standard formula.
Plasma, kidney and liver vitamin E was determined by the method of Arnaud et al.Citation[[19]] according to the following procedures. A 0.5 mL or 0.5 g of plasma, kidney or liver was homogenized in 2.0 mL ethanol and pipetted into 2.0 mL n-hexane, with shaking for 2 min. A 0.5 mL aliquot of the supernatant (n-hexane) was pipetted into a test tube, dried under nitrogen, re-suspended in 0.5 mL of the mobile phase and filtered. The analyses were performed with an HPLC instrument (Shimadzu LC-9A), using a C18 column (4.6 × 25.0 cm), and acetonitrile/dichloromethane/methanol (70/20/10) as the mobile phase at a flow of 2 mL/min.
Statistical Analysis
Data are reported as means ± standard deviation (x ± SD) and were analyzed statistically by the nonparametric Kruskal–Wallis test,Citation[[20]] with the level of significance set at p<0.05. To determine possible correlations between groups the test of significance for the Pearson correlation coefficient was used.Citation[[20]]
RESULTS
The renal function of control animals and of animals submitted to subtotal nephrectomy was determined on the basis of plasma urea and creatinine and 24 h urinary albumin levels and GFR. The plasma urea and creatinine levels of sham-operated animals and of animals submitted to subtotal nephrectomy were measured 24 h (T1) and 15 days (T2) after surgical intervention. The normal and deficient experimental groups presented respective mean urea values of 130.56 ± 29.77 mg/dL and 111.06 ± 30.34 mg/dL (T1) and 43.15 ± 6.75 mg/dL and 49.96 ± 8.74 mg/dL (T2) and mean creatinine values of 2.99 ± 1.11 mg/dL and 2.26 ± 0.86 mg/dL (T1) and 0.99 ± 0.15 mg/dL 9 and 1.45 ± 0.92 mg/dL (T2), which were significantly higher (p < 0.05) than those for the normal control group (T1: 25.57 ± 6.0 mg/dL and 0.53 ± 0.07 mg/dL; T2: 27.35 ± 5.75 mg/dL and 0.56 ± 0.08 mg/dL) and for the deficient control (T1: 23.59 ± 1.35 mg/dL and 0.68 ± 0.13 mg/dL; T2: 31.27 ± 6.54 mg/dL and 0.43 ± 0.11 mg/dL). Fifteen days after surgery (T2), 24 h urinary albumin levels were 0.46 ± 0.21 mg/dL and 0.60 ± 0.24 mg/dL in nephrectomized rats submitted to a normal and a deficient diet, respectively, significantly higher values (p<0.05) compared to the normal and deficient control groups (0.10 ± 0.08 mg/dL and 0.19 ± 0.15 mg/dL, respectively).
The GFR () of the nephrectomized rats submitted to a normal diet and a deficient diet was significantly lower (p<0.05) than that of the normal and deficient control groups.
Table 1. Glomerular Filtration Rate (GFR) of the Groups Submitted to Sham Surgery and to Subtotal Nephrectomy Determined 15 Days (T2) After Surgery
Mean vitamin E levels of the sham and normal experimental groups were 21.42 ± 8.12 and 28.42 ± 7.40 mmol/L in plasma, 56.01 ± 21.80 and 28.79 ± 9.69 mg/g tissue in the liver, and 37.79 ± 6.66 and 23.68 ± 2.69 mg/g tissue in the kidney, respectively, and were significantly lower (p<0.05) than the levels detected in the plasma (4.92 ± 1.22 and 8.37 ± 2.09 mmol/L), liver (7.57 ± 2.72 and 9.44 ± 2.55 mg/g tissue) and kidney (8.17 ± 2.38 and 9.40 ± 3.10 mg/g tissue) of the sham and deficient experimental groups, respectively. The results are demonstrated in .
Table 2. Vitamin E in the Liver and Kidney of Sham Rats Receiving a Normal Diet (SDN), Experimental Rats Receiving a Normal Diet (EDN), Sham Rats Receiving a Deficient Diet (SDD), and Experimental Rats Receiving a Deficient Diet (EDD), 15 Days (T2) After Surgical Intervention, Mean ± SD
The extent of lipid peroxidation (MDA) was measured on the basis of TBARS levels. Fifteen days after surgery (T2) mean TBARS in liver and kidney were significantly higher (p<0.01) in deficient nephrectomized animals (1.41 ± 0.27 nmol/g protein, and 1.67 ± 0.47 nmol/g protein, respectively) than in nephrectomized rats receiving a normal diet (0.66 ± 0.14 nmol/g protein and 0.90 ± 0.23 nmol/g protein), normal controls (0.71 ± 0.18 nmol/g protein; 0.76 ± 0.11 nmol/g protein) and deficient controls (0.76 ± 0.13 nmol/g protein; 0.96 ± 0.21 nmol/g protein) as demonstrated in . No significant correlation (p>0.05) was observed between TBARS levels and vitamin E levels in the liver and kidney of the various groups studied.
Table 3. TBARS in the Liver and Kidney of Sham Rats Receiving a Normal Diet (SDN), Experimental Rats Receiving a Normal Diet (EDN), Sham Rats Receiving a Deficient Diet (SDD), and Experimental Rats Receiving a Deficient Diet (EDD), 15 Days (T2) After Surgical Intervention, Mean ± SD
SOD concentrations in the erythrocytes of sham-operated animals and of animals submitted to 5/6 nephrectomy 15 days after surgery (T2) are listed in . It can be seen that SOD concentrations were significantly higher (p<0.05) in deficient nephrectomized rats (4455.80 ± 615.12 U/g Hb) than in nephrectomized rats receiving a normal diet (1877 ± 402.16 U/g Hb), and normal and deficient controls (2257.80 ± 416.00 U/g Hb and 1938.42 ± 615.12 U/g Hb, respectively). Also, there was no correlation between erythrocyte SOD activity and vitamin E concentration in plasma, liver or kidney or TBARS concentrations in the liver and kidney of the groups studied. However, there was a positive correlation between erythrocyte SOD activity and plasma creatinine in the nephrectomized deficient group.
Figure 1. Erythrocyte SOD activity on the 15th day after surgical intervention (T2) in sham rats receiving a normal diet (SDN), experimental rats receiving a normal diet (EDN), sham rats receiving a deficient diet (SDD), and experimental rats receiving a deficient diet (EDD). Values are presented as means ± SD. *p < 0.05.
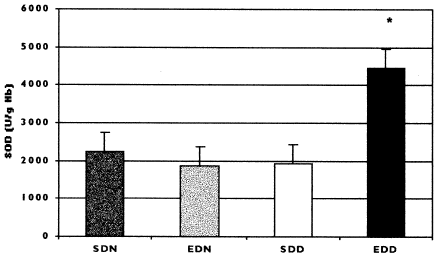
DISCUSSION
The so-called remaining kidney model or 5/6 nephrectomyCitation[[10]] is currently the model most often utilized to induce chronic renal insufficiency in animals. The principle of this model is that, after reducing the renal mass to 1/6 of the original size, the renal overload for the remaining nephrons results in progressive damage, with consequent end-stage renal failure. Hypertrophy of the remaining kidney, the increase in intraglomerular pressure and renal blood flow, intracapillary coagulation and proteinuria are factors that may contribute to the evolution of fibrosis with a progressive loss of renal function followed by uremia.
In the present study, urea and creatinine levels increased significantly (p<0.05) 24 h and 15 days after surgery for 5/6 ablation of the renal mass in the experimental groups receiving normal and vitamin E-deficient diets compared to control. No significant difference was detected between these groups. In addition, no significant change was observed in the urea and creatinine levels of sham-operated animals receiving a normal or vitamin E-deficient diet.
Thus, the high urea and creatinine levels were probably related to the 5/6 reduction of renal mass. This may perhaps be explained by the fact that during the early phase of renal failure there is an increase in serum urea and creatinine levels resulting from the increased catabolism of the muscle mass.Citation[[21]] The progressive losses in renal function are usually represented by increased urea and creatinine concentrations. However, these values vary according to the different approaches and the extent of renal mass reduction.Citation[[22]] Thus, rats submitted to subtotal nephrectomy by pole resection present significantly increased serum urea levels when sacrificed 1, 2 and 3 weeks after nephrectomy.Citation[[23]] Similar results were obtained by Eliahou et al.Citation[[24]] who observed increased serum urea levels of 67, 63 and 59 mg/dL during the 1st, 4th and 8th week after surgery, respectively.
Thus, we may propose that in the present study the vitamin E-deficient diet had no effect on renal function parameters in rats submitted to subtotal nephrectomy. We suggest that the changes in renal function that occurred in the nephrectomized animals were related to the remaining functioning nephrons undergoing gradual deterioration.
In the present study, after 15 days of subtotal nephrectomy, renal injury was characterized by a significant increase in the rate of urinary albumin excretion and a reduction in GFR both in the normal and in the deficient experimental groups. These changes reflected impaired general kidney function in response to subtotal nephrectomy. Vitamin E-deficient nephrectomized rats also showed urinary albumin and GFR similar to those of nephrectomized animals receiving a normal vitamin E diet.
Proteinuria is the major manifestation of glomerular disease, reflecting increased vascular permeability to macromolecules, including albumin. Hostetter et al.Citation[[25]] reported that urinary protein excretion was significantly increased in rats receiving a high protein diet submitted to subtotal nephrectomy. The rate of urinary protein excretion was 3–5 times higher compared to rats on a low protein diet.
Another common manifestation of chronic renal insufficiency is reduced GFR.Citation[[26]] In the present study, GFR was significantly reduced in animals submitted to subtotal nephrectomy. Studies on humans have demonstrated that the “functional reserve” may disappear in the presence of a considerable number of nephrons.Citation[[27]]
In the present study, the efficiency of dietary treatments in terms of vitamin E was confirmed by the determination of vitamin E concentrations in plasma, liver and kidney. It can be seen that, 15 days after nephrectomy (T2), the deficient control and experimental groups maintained lower mean vitamin E levels, whereas the normal control and experimental groups maintained higher mean levels. These results show that the deficient diet probably caused a reduction in plasma, hepatic and renal vitamin E concentrations in the deficient control and experimental groups and the normal diet probably maintained vitamin E concentrations in the normal control and experimental groups. Furthermore, no additional vitamin E consumption occurred in the presence of the renal injury provoked by subtotal nephrectomy in the deficient experimental groups.
According to Vannucchi et al.Citation[[28]] vitamin E deficiency itself is responsible for greater susceptibility to oxidative injury. Ong-Ajyooth et al.Citation[[8]] reported that patients with chronic renal insufficiency presented significantly reduced concentrations of vitamin E, glutathione, glutathione peroxidase and catalase compared to control. These data confirm that a change in the antioxidant system is associated with increased lipid peroxidation. Furthermore, the reduction in erythrocyte vitamin E concentrations may contribute to the onset of anemia in these patients.
To determine the production of free radicals after renal injury induced by subtotal nephrectomy we determined TBARS concentrations, with MDA being the main substance resulting from this reaction. In the present study, liver and kidney TBARS levels were significantly increased in the deficient experimental group 15 days after subtotal nephrectomy. This result indicates that vitamin E deficiency made the animals more susceptible to lipid peroxidation 15 days after subtotal nephrectomy. On the other hand, in the normal experimental animals TBARS values were not significantly different from control. Thus, it can be seen that vitamin E in recommended amounts played a protective role against the toxic effects of the presence of free radicals.
According to Doni et al.Citation[[29]] rats fed a vitamin E-deficient diet present increased renal MDA after 3 months of study. This increase was accompanied by signs of platelet lipid peroxidation and increased erythrocyte fragility.
In the present study, the antioxidant system was assessed on the basis of erythrocyte SOD activity. The results showed a significant increase in SOD activity only in the deficient experimental group compared to all other groups studied. Furthermore, in the deficient experimental group the high SOD activity was correlated with high plasma creatinine levels. We suggest that the increase in erythrocyte SOD activity observed in the deficient experimental group served as a defense mechanism in an attempt to protect the cells against the toxic effects provoked by the increase in reactive oxygen species formed in response to subtotal nephrectomy and to vitamin E deficiency. However, the increase in erythrocyte SOD activity observed in this group may have contributed to the occurrence of an additional increase in lipid peroxidation since SOD converts superoxide anion to hydrogen peroxide (H2O2). Thus, excessive production of reactive oxygen species may have resulted in increased SOD expression.Citation[[30]] On the other hand, excessive SOD production may have caused a reduction in catalase concentration, with consequent inefficient removal of H2O2. Both the increase and the reduction of antioxidant enzymes may exacerbate the production of reactive oxygen species, thus representing evidence of oxidative stress.Citation[[31]] Furthermore, no increase in SOD activity occurred in the nephrectomized rats receiving a normal vitamin E diet. We suggest that the increase in SOD activity did not occur in these animals due to the low production of reactive oxygen species, as shown by TBARS levels in liver and kidney.
Chen et al.Citation[[32]] observed that SOD activity was increased in 50% of renal patients and suggested that the production of oxygen reactive species by neutrophil infiltration resulted in increased SOD activity as a protective mechanism. A significant increase in SOD activity was also observed in patients with different degrees of chronic renal failure.Citation[[33]] The activity of the enzyme increased with the progress of chronic renal failure and was more pronounced in patients with end-stage disease. The authors suggested that the increase in SOD activity occurred as a mechanism of cell protection in response to the increased production of free radicals in chronic renal failure.
Thus, we may conclude that the increased oxidative damage and deterioration of the antioxidant system may contribute to the development of uremic complications such as red blood cell destruction and the consequent onset of anemia.Citation[[33]] On this basis, prospective studies are needed to confirm the benefits of antioxidant therapy, especially vitamin E, in an attempt to minimize progressive renal damage in patients with chronic renal failure.
REFERENCES
- Ichikawa I., Kiyama S., Yoshioka T. Renal Antioxidant Enzymes: Their Regulation and Function. Kidney Intern. 1994; 45: 1–9
- Halliwell B. Free Radicals and Antioxidants: A Personal View. Nutr. Rev. 1994; 52: 253–265
- Liebler D.C. The Role of Metabolism in the Antioxidant Function of Vitamin E. Critical Reviews in Toxicology 1993; 23: 147–169
- Miguel A., Miguel A., Linares M. Evidence of an Increased Susceptibility to Lipid Peroxidation in Red Blood Cells of Chronic Renal Failure Patients. Nephron 1988; 50: 64–65
- Durak I., Akyol Ö., Basesme E., Canbolat O. Reduced Erythrocyte Defense Mechanisms Against Free Radical Toxicity in Patients with Chronic Renal Failure. Nephron 1994; 66: 76–80
- Weiss S.J. Tissue Destruction by Neutrophils. N. Engl. J. Med. 1989; 320: 365–367
- Nath K.A. Tubulointerstitial Changes as a Major Determinant in the Progression of Renal Damage. Am. J. Kidney Dis. 1992; 20: 1–17
- Ong-Ajyooth L., Ong-Ajyooth S., Tiesong K. Reduced Free Radical Scavengers and Chronic Renal Failure. J. Med. Assoc. Thai. 1997; 80: 101–108
- Machlin L.J., Bendich A. Free Radical Tissue Damage: Protective Role of Antioxidant Nutrients. FASEB J. 1987; 1: 441–445
- Platt R., Roscoe M.H., Smith F.W. Experimental Renal Failure. Clin. Sci. 1952; 11: 217–231
- Chantler C., Lieberman E., Holliday M.A. A Rat Model for the Study of Growth Failure in Uremia. Pediat. Res. 1974; 8: 109–113
- Association of Official Analytical Chemists (A.O.A.C.). Official Methods of Analysis, 12th Ed. Washington, DC 1975; 857
- Uchiyama M., Mihara M. Determination of Malonaldehyde Precursor in Tissues by Thiobarbituric Acid Test. Anal. Biochem. 1978; 86(1)271–278
- Lowry O.M., Rosebrough N.J., Farr A.L. Protein Measurement with the Folin Phenol Reagent. J. Biol. Chem. 1951; 193: 265–275
- Oyanagui Y. Reevaluation of Assay Methods and Establishment of Kit for Superoxide Dismutase Activity. Anal. Biochem. 1984; 142: 290–296
- Van Assendelft O.W. The Measurement of Hemoglobin. Modern Concepts in Hematology, G. Izak, S.M. Lewis. Academic Press, New York 1972; 14–25
- Schmid H., Bertoluci M.C., Coimbra T.M. Determinação da excreção urinária de albumina por eletroimunoensaio (EIE). Arq. Bras. Endocrinol. Metabol. 1989; 33: 75–76
- De-Barros-Silva M.L., Varanda W.A., Lachat J.J. Glomerular Permeablity to Macromolecules in Gentamicin-Treated Rats. Brazilian J. Med. Res. 1992; 25: 409–417
- Arnaud J., Fortis I., Blachier S. Simultaneous Determination of Retinol, Alpha-Tocopherol, and Beta-Carotene in Serum by Isocratic High Performance Liquid Chromatography. J. Chromatogr 1991; 572: 103–116
- Mosteler F., Rouker R.E. Sturdy Statistics. Adson Wefley, New York 1970; 396
- Brenner B.H. Hemodynamically Mediated Glomerular Injury and the Progressive Nature of Kidney Disease. Kidney Int. 1983; 23: 647–655
- Gretz N., Meisinger E., Waldherr R. The Remment Kidney Model. Experimental and Genetic Rat Models of Chronic Renal Failure, N. Gretz, M. Strauch. Karger, Basel 1993; 1–28
- Gretz N., Meisinger E., Waldherr R. Acute Renal Failure After 5/6 Nephrectomy; Histological and Functional Changes. Contr. Nephrol. 1988; 60: 56–63
- Eliahou H.E., Cohen D., Herzog D. The Control of Hypertension and its Effect on Renal Function in Rat Remmant Kidney. Nephrol. Dial. Dial. Transplant. 1988; 3: 38–44
- Hostetter T.H., Meyer T.W., Rennke H.G. Chronic Effects of Dietary Protein in Rat with Intact and Reduced Renal Mass. Kidney Int. 1986; 30: 509–517
- Fox R.B. Prevention of Granulocyte-Mediated Oxidant Lung Injury in Rats by a Hydroxyl Radical Scavenger, Dimethiourea. J. Clin. Invest. 1984; 74: 1456–1464
- Bosch J.P., Lauer A., Glabman S. Short-Term Protein Loading in the Assessment of Patients with Renal Disease. N. Engl. Med. 1984; 77: 873–879
- Vannucchi H., Jordão-Jr. A.A., Iglesias A.C.G. Effect of Different Dietary Levels of Vitamin and on Lipid Peroxidation in Rats. Arch. Latinoam. Nutr. 1997; 47: 34–37
- Doni M.G., Falanga A., Delaini F. The Effect of Vitamin and or Selenium on the Oxidant-Antioxidant Balance in Rats. Br. J. Exp. Path. 1994; 65: 75–80
- Yoshioka T., Bills T., Moore-Jarett T., Greene H.L. Role of Intrinsic Antioxidant Enzymes in Renal Oxidant Injury. Kidney Intern. 1990; 38: 282–288
- Wang J.S., Ger L.P., Tseng H.H. Expression of Glomerular Antioxidant Enzymes in Human Glomerulonephritis. Nephron 1997; 76: 32–38
- Chen H.C., Tomino Y., Yaguchi Y. Detection of Polymorphonuclear Cells, Superoxide Dismutase and Poly C9 in Glomeruli of Patients with IgA Nephropathy. Nephron 1991; 59: 338
- Mimic-Oca J., Simic T., Ekmescic V. Erythrocyte Glutathione Peroxidase and Superoxide Dismutase Activities in Different Stages of Chronic Renal Failure. Clinical Nephrology 1995; 44: 44–48