Abstract
The aim of the present study was to investigate the suitability of amidated pectin matrix patch for transdermal chloroquine delivery in an effort to mask the bitter taste when orally administered. Chloroquine has easily measurable outputs that are linked to increased renal Na+ excretion. We thus monitored urinary Na+ output in separate groups intravenously administered chloroquine or topically applied pectin hydrogel chloroquine matrix patch. Male groups of anesthetized Sprague-Dawley rats were placed on a continuous jugular infusion of 0.077 M NaCl at 150 µL min−1. After 3 h equilibration period, consecutive 20 min urine collections were made over the subsequent 4 h of 1 h control, 1 h 20 min treatment, and 1 h 40 min recovery periods for measurements of urine flow and Na+ and K+ excretion rates. The effects of intravenous chloroquine infusion or topical application of pectin hydrogel chloroquine matrix patch were examined in rats in which the drug was added to the infusate or patch applied onto the shaved area during the 1 h 20 min treatment period. The animals were switched back to the infusate alone for the final 1 h 40 min recovery period. Vehicle infused animals acted as controls. Trunk blood was collected after the treatment period from parallel groups for chloroquine measurements. The plasma chloroquine concentrations following iv chloroquine or application of pectin chloroquine hydrogel matrix patch were 9.3 ± 0.8 mg L−1 and 7.3 ± 1.1 mg L−1, respectively (n = 7 in both groups). Chloroquine infusion and pectin chloroquine patch significantly (p<0.01) increased Na+ excretion to peak values of 14.1 ± 0.9 µmol min−1, and 20.35 ± 1.0 µmol min−1, respectively by comparison with controls (9.1 ± 0.9 µmol min−1), at the corresponding period. The results suggest that the pectin chloroquine patch matrix preparation has potential applications for transdermal delivery of chloroquine and perhaps in the management of malaria.
Introduction
Chloroquine is the mainstay therapy for malaria in Africa.Citation[[1]] However, therapeutic failure is common due to patient noncompliance probably due to its bitter taste when given orally or repeated doses. Chloroquine resistant strains of Plasmodium falciparum have been reported arising perhaps from the inconvenient oral dosing schedule. Hence, the search for chloroquine preparations, which not only mask the bitter taste, but also lead to the modification of the dosing schedule has aroused much interest.Citation[[1]], Citation[[2]], Citation[[3]] We have previously reported successful prolonged release formulation of amidated pectin hydrogel beads chloroquine in an attempt not only to modify the dosing schedule, but also to mask the bitter taste.Citation[[3]] The skin has increasingly become a route for the delivery of drugs with a range of compounds being considered for transdermal delivery generating a great deal of interest in this area of research.Citation[[4]] Chloroquine is a good candidate for transdermal drug delivery considering its bitterness when orally administered and extensive use to manage malaria. Transdermal drug delivery offers a noninvasive route of drug administration, although its applications are limited by low skin permeability. However, this route is an attractive approach for either local or systemic treatment in medicine. In the last decade, different active transdermal delivery methods have been further investigated such as cationic liposomal delivery and electroporation-enhanced delivery. The main objective of the current study was to investigate the suitability of pectin as a transdermal drug delivery matrix for chloroquine. Pectin (polygalacturonic acid) hydrogel matrix previously used for specific oral delivery to the colonCitation[[3]], Citation[[5]] was chosen for transdermal delivery for chloroquine in this study.
Chloroquine has easily measurable outputs that are linked to increased renal Na+ excretion in the anesthetized rat following iv infusion.Citation[[6]] Therefore, we compared urinary Na+ outputs patterns in rats after intravenous chloroquine and in animals topically applied the pectin hydrogel chloroquine matrix patch to test formulation of the patch.
Methods and Materials
Animals
Male Sprague-Dawley rats (300–350 g body weight) bred and housed in the Medical Faculty animal house in the University of Zimbabwe were used. The animals were maintained on a 12 h light/12 h dark regime and allowed free access to food (Mouse Comproids, National Foods, Zimbabwe, Harare) and water at all times.
Chemicals
Chloroquine diphosphate, calcium chloride, and monobasic potassium phosphate, were obtained from Sigma Chemical Company, St. Louis, MO, USA. Sodium hydroxide, potassium hydroxide, chloroform, ethanol, methanol, and dimethyl sulphoxide were obtained from Associated Chemical Enterprises, Republic of South Africa. Hydrochloric acid (HCl, 32% v/v) was obtained from Saarchem Muldedrift, Republic of South Africa. Amidated low-methoxyl pectin with a degree of methoxylation (DM) of 23, degree of amidation (DE) of 24 was donation from Balmer Pectin, UK. The aqueous buffer system used was Sorensen's Phosphate buffer (pH 1.0 and 7.4).
Pectin Chloroquine Patch Preparation
The method of hydrogel patch production is similar to that previously reported for beads.Citation[[5]], Citation[[7]] Pectin (4 g) was dissolved in 100 mL distilled water with agitation using a mixer (Silverson laboratory mixer emulsifier UK) to which chloroquine diphosphate (10 g) was added and mixed with agitation. Subsequently, dimethyl sulphoxide (3 mL) was added. Following this, an aliquot of the mixture (11 mL) was transferred to a petri dish (424.62 cm2) and frozen at −5°C. After freezing, a 2% CaCl2 solution was added on top of the frozen pectin and left to stand at room temperature for 10 min to allow for cross-linking. Patches with measured widths were cut out and placed on parafilm that served as backing material. The mean calculated area of patches used in the study is given in . The patches were stored at 2°C in a refrigerator until use. Paper adhesive labels (Fleximail Art Corporation, Zimbabwe) were placed around the pectin hydrogel chloroquine matrix cut out to allow its adhesion onto the animal.
Table 1. Mean area measurements and chloroquine loading efficiency of pectin hydrogel matrix patch, (n = 6)
In Vitro Studies of Chloroquine Release
The release of chloroquine from pectin hydrogel chloroquine matrix patch into 900 mL aqueous dissolution medium of Clark and Lub's potassium chloride–hydrochloric acid solution (pH 1.0 and 7.4) at 37°C was investigated by the BP method 1 Apparatus in six separate patches. The pectin hydrogel chloroquine matrix patch was mixed at 37°C at 100 rpm (rotations per minute) using saddles. Five millilitre samples were withdrawn hourly until the absorbance was constant. Absorbance was determined by the UV spectrophotometer (Shimadzu UV visible–160A, Japan).
Renal Function Studies
The animals were anesthetized by intraperitoneal injection of Trapanal [sodium 5-ethyl-5′-(1-methyl-butyl)-2-thio-barbiturate, Byk Gulden, Konstanz, Germany] at 0.11 g kg−1 body weight and challenged with hypotonic saline (0.077 M NaCl) infusion. The right jugular vein was cannulated with polyethylene tubing (i.d., 0.86 mm; o.d. 1.27 mm, Clay Adams, New Jersey, USA) to allow intravenous infusion of 0.077 M NaCl. The urinary bladder was cannulated with polyethylene tubing of the same size via an abdominal incision. Each rat was tracheotomized to maintain a clear airway. The body temperature was maintained at 37 ± 1°C with a heated table. Rats were placed on a continuous infusion of 0.077 M NaCl at 150 µL min−1 (Sage Syringe Pump Model 351) and a 3-h equilibration period was allowed. Following this, consecutive 20 min urine collections were made into pre-weighed plastic vials over the subsequent 4 h, of 1 h control, 1 h 20 min treatment, and 1 h 40 min post-treatment recovery periods. The effects of intravenous infusion of chloroquine at 0.06 µg min−1 or topical application of pectin hydrogel chloroquine patch were examined in rats in which the drug was added to the infusate or patch applied onto the shaved area during the 1 h 20 min treatment period. The animals were switched back to the infusate alone for the final 1 h 40 min recovery periods. An additional control group of animals was sham applied with drug free pectin hydrogel matrix patches. The rate of chloroquine administration was chosen from experience of previous studies.Citation[[8]] The dose of iv chloroquine used in the current study (16 µg kg−1) was significantly (p<0.01) lower than those previously used in rats (20–25 mg kg−1,Citation[[9]] and in man 300 mg kg−1.Citation[[10]]
Trunk blood was collected from parallel groups of animals prepared for renal studies at the end of 1 h 20 min treatment period and 1 h 40 min post-treatment period for plasma chloroquine measurements. The rats were sacrificed by decapitation and blood was collected following decapitation in heparinized glass beakers coated with sigmacote (Sigma Chemical Company, St. Louis, MO, USA) to prevent adsorption of chloroquine on glass walls. The plasma was separated and stored in a Bio Ultra freezer (Malkinckroodt, Ohio) at −70°C until further use.
Chloroquine Assay
The amount of chloroquine in the pectin chloroquine patch was determined after dissolving the patch in Clark and Lub's potassium chloride–hydrochloric acid solution (pH 7.4). The blank contained chloroquine free pectin patches dissolved Clark and Lub's potassium chloride–hydrochloric acid solution (pH 7.4). Chloroquine concentration in the plasma was assayed following extraction as previously described.Citation[[3]] Initially, 0.5 mL of potassium hydroxide (16 M) was added to a separator flask containing 4 mL plasma, followed by 25 mL chloroform. The mixture was well shaken. Subsequently, chloroquine was extracted from the lower chloroform layer (21 mL) with 4 mL hydrochloric acid (1 M) and measured spectrophotometrically using plasma as a blank. The mean chloroquine incorporation efficiency onto the pectin hydrogel matrix is given in .
Data Presentation and Statistical Analysis
Values are presented as means ± SEM. The loading efficiency of the beads was calculated by dividing the actual chloroquine content in the patches by the theoretical content and expressed as a percentage. Renal excretion data are presented graphically showing 20 min collections over the 4 h post-equilibration period. The total fluid voided and Na+ and K+ excreted during the 1 h 20 min of chloroquine infusion or pectin chloroquine patch attachment was compared with values from control vehicle-infused animals at the corresponding period. The data were subjected to one-way analysis of variance. Scheffe's multiple comparison test was used to resolve any apparent differences. A value of p<0.05 was considered significant.
Results
Chloroquine Loading Efficiency
The mean area and chloroquine loading efficiency of the pectin hydrogel matrix patch used in the study are given in .
In Vitro Chloroquine Release
The release of the entrapped chloroquine from pectin hydrogel patch in Clark and Lub's potassium chloride–hydrochloric acid solution (pH 1.0) was low reaching approximately 10% by 6 h (). On the other hand, there was 30% release of chloroquine incorporated in pectin patch released in the solution at pH 7.4 after 2 h and 76% release after 6 h.
Figure 1. Dissolution profiles of chloroquine from chloroquine loaded amidated pectin hydrogel matrix patch at pH 1.0 (▪) and pH 7.4 (▴) obtained by the basket method in dissolution medium of Clark and Lub's potassium chloride–hydrochloric acid solution at 37°C, at 100 rpm. Each point represents the mean of three experiments.
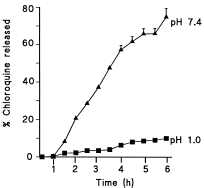
Renal Studies
Urine Flow and Electrolyte Excretion Rates in Control Rats
During the 4 h post-equilibration period, urine flow and Na+ excretion rates in vehicle-infused control animals stabilized at rates comparable to the infusion rates (A–B). The urine flow rate, which ranged from 141 to 161 µL min−1 compared with the infusion rate (150 µL min−1). Na+ excretion rate stayed around 8.5 µmol min−1, a value, which was not significantly different from the infusion rate (11.6 µmol min−1). K+ excretion rates were stable approximating 3.5 µmol min−1 throughout the post-equilibration period.
Figure 2. Na+ and K+ excretion rates in 0.077 M NaCl infused control rats (A), rats administered chloroquine (Chq) at 0.06 µg min−1 (B) or topically applied pectin hydrogel chloroquine matrix patch (C) for 1 h 20 min. Values are presented as means for each 20 min collection for the 4 h post-equilibration period; vertical bars indicate S.E. of means (n = 7 in all groups).
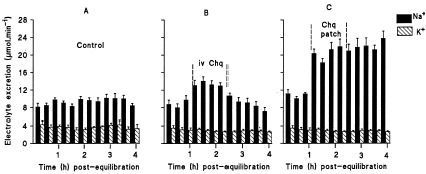
Renal Effects of Chloroquine
Administration of chloroquine increased Na+ excretion rate rising to 14.1 ± 0.9 µmol min−1 (n = 7) by 40 min after the commencement of chloroquine infusion, a value which was significantly (p<0.01) higher than that of controls (9.1 ± 0.9 µmol min−1, n = 7) at the corresponding period (cf A and B). There was no significant effect on K+ excretion caused by chloroquine administration. When compared with vehicle-treated controls, pectin drug free patch application did not affect urine flow or Na+ and K+ excretion rates did since the total fluid volume voided and electrolytes excreted during the treatment time compared with values of control animals at the corresponding period (See ).
Table 2. Plasma chloroquine concentration at the end of the treatment time and total amounts of urine voided and Na+ and K+ excreted during the treatment period (n = 7 in all groups)
C shows that Na+ and K+ excretion rates for the group applied pectin chloroquine patch on the skin during the treatment period. Topical application of the patch significantly increased (p<0.05) Na+ excretion by comparison with all groups (cf, A, B, and C). In this group of animals, Na+ excretion rate was elevated to a maximum of 21.9 µmol min−1 by 60 min after the application of the patch. The rate was significantly (p<0.05) higher by comparison with that of control rats and those animals infused chloroquine at the corresponding time. This increase in Na+ excretion was maintained during the post-treatment period.
Cumulative Data
The mean total volume of urine and amounts of Na+ and K+ excreted in 1 h 20 min of the treatment period are presented in . By comparison with values from control animals at the corresponding period, intravenous chloroquine or pectin chloroquine patch application significantly (p<0.01) elevated urinary Na+. In all treated groups, the total urine and K+ excretion outputs were not significantly altered by any of the treatments.
Plasma Chloroquine Concentration
The plasma chloroquine concentration in rats intravenously administered chloroquine or topical application of pectin hydrogel drug-free patch and pectin hydrogel chloroquine patch on the skin for 1 h 20 min are shown in .
Discussion
The present study investigated the potential application of pectin hydrogel patch as a matrix polymer for transdermal chloroquine delivery. Transdermal chloroquine delivery indices from the pectin hydrogel chloroquine patch permeated through the skin were quantified by the concentration of the antimalarial in the plasma and the effects on urinary Na+ output. We have previously reported that acute intravenous infusion of chloroquine increases renal Na+ excretion in the rat.Citation[[8]] We suggest successful transdermal delivery of the antimalarial since chloroquine detected in the plasma was associated with a concomitant increase in urinary Na+ output following topical application of the pectin chloroquine hydrogel matrix patch on the rat skin.
The loading efficiency of chloroquine in the pectin matrix patch was low () probably due to the high water solubility of the drug which might have led to its loss in the cross linking medium. The factors which affect the loading of the drug into the pectin matrix include the amount of drug to be loaded the dispersion of the drug in the pectin solution just before the gelling process, the viscosity of the drug/pectin solution and the loss of drug during the gelling process.Citation[[11]]
The amount of chloroquine released in a specified time from the pectin hydrogel patch decreased when the pH of the dissolution medium was lowered () suggesting final release in alkaline. At low pH values electrostatic repulsion between the carboxylic acid groups of polygalacturonic acid is low, by that increasing gel strength and minimizing release of the drug via diffusion.Citation[[12]] However, in alkaline media the presence of excess hydroxyl ions increases the electrostatic repulsion between pectin molecules thus solubilizing the gels.Citation[[13]]
The plasma concentration of chloroquine in rats reported in this study following intravenous chloroquine infusion or application of chloroquine pectin hydrogel patch on the skin compares to values previously reported.Citation[[14]] Thus, we believe that transdermal chloroquine delivery by pectin hydrogel patch produced plasma concentration that increased urinary Na+ output. We have previously reported that acute intravenous chloroquine infusion increases plasma AVP to increase renal Na+ excretion.Citation[[8]] Intravenous infusion of physiological doses of AVP in rats increases renal Na+ excretion via V1 receptors.Citation[[15]] Urinary Na+ excretion was significantly elevated in animals in which chloroquine was delivered by the transdermal route by comparison with the iv route although plasma concentrations of the drug were similar in both groups of animals. Results from the current study cannot explain this discrepancy without GFR or plasma aldosterone levels data, parameters known to influence renal Na+ handling. However, we have previously reported that iv chloroquine infusion at rates used in the current study do not affect GFR and aldosterone levels.Citation[[14]] Increased urinary Na+ output persisted in the post-treatment period in animals applied chloroquine pectin hydrogel patch on the skin perhaps due to continued release of residual chloroquine (cf A and B). Current evidence indicates intradermal disposition of drugs after topical application.Citation[[16]] Thus, it is very important to regulate the intradermal disposition of drugs after topical application to develop a more efficient transdermal delivery system. Applications of transdermal drug delivery are limited by low skin permeabilityCitation[[17]] and hence many chemicals have been used to enhance skin permeability.Citation[[18]] In this study, dimethyl sulphoxide was incorporated into the pectin matrix patch as a penetration enhancer to increase the skin permeation of chloroquine. Dimethyl sulphoxide has been established to increase transdermal penetration of drugs.Citation[[19]]
In summary, results from the present study suggest that the hydrogel chloro-quine/pectin patch has potential applications for transdermal delivery of the antimalarial. The route is envisaged to mask the bitter taste of chloroquine to perhaps improve patient compliance and in some cases quality of life where chronic therapy is required.
Acknowledgment
The authors wish to thank the following: Byk Gulden, Konstanz, Germany for the gift of Trapanal and Balmer Pectin, UK for the donation of amidated low-methoxyl pectin.
References
- Moran J.S., Bernard K.W. The spread of chloroquine-resistant malaria in Africa: implications for travellers. Journal of American Medical Association 1989; 262(2)245–248
- Hoffman A.F., Pressman J.H., Code C.F., Wiztun K.F. Controlled entry of orally administered drugs: physiologic considerations. Drug Development and Industrial Pharmacology 1983; 9: 1077–1090
- Munjeri O., Hodza P., Osim E.E., Musabayane C.T. An investigation into the suitability of amidated pectin hyrogel beads as a delivery matrix for chloroquine. Journal of Pharmaceutical Science (USA) 1998; 87(8)905–908
- Mitragotri S. Synergistic effect of enhancers for transdermal drug delivery. Pharmaceutical Research 2000; 17(11)1354–1359
- Rubinstein A., Radai R., Ezra M., Pathak S., Rokem J.S. In vitro evaluation of calcium pectinate: a potential colon-specific drug delivery carrier. Pharmaceutical Research 1993; 10: 258–263
- Musabayane C.T., Windle R.J., Forsling M.L., Balment R.J. Arginine vasopressin mediates the chloroquine induced increase in renal sodium excretion. Tropical Medicine & International Health (London) 1996; 1(4)542–550
- Thompson P.E., Olslewski B.J., Elsanger E.F., Worth D.F. Laboratory studies on 4,6-diamino-1-(p-chlorophenyl)-1,2-dimethyl-s-triazine pamoate (CI-501) as a repository antimalarial drug. American Journal of Tropical Medicine and Hygiene 1963; 12: 481–493
- Musabayane C.T., Ndhlovu C.E., Mamutse G., Bwititi P., Balment R.J. Acute chloroquine administration increases renal sodium excretion. Journal of Tropical Medicine & Hygiene (Lond.) 1993; 96: 305–310
- Sofola O.A., Olude I.O., Adegoke F. The effects of chronic chloroquine toxicity on blood pressure in rats. Journal of Tropical Medicine & Hygiene 1981; 84: 249–252
- Looaresuwan S., White N.J., Chanthavantch P., Edwards G., Nicholl D.D., Bunch C., Warrell D.A. Cardiovascular toxicity and distribution kinetics of intravenous chloroquine. British Journal of Clinical Pharmacology 1986; 22: 31–36
- Wong T.W., Chan L.W., Lee H.Y., Heng P.W. Release characteristics of pectin microspheres prepared by an emulsification technique. Journal of Microencapsulation 2002; 19(4)511–522
- Pillay V., Fassihi. R. In vitro release modulation from crosslinked pellets for site-specific drug delivery to the gastrointestinal tract. I. Comparison of pH-responsive drug release and associated kinetics. Journal of Controlled Release 1999; 59(2)229–242
- Radai R., Rubinstein A. In vivo analysis of colon specificity of calcium pectinate formulations. Proc. Int. Symp. Control Release Bioact. Matex. 1993; 20: 330–331
- Musabayane C.T., Cooper R.G., Osim E.E., Balment R.J. Renal electrolyte and fluid handling in the rat following acute chloroquine and/or ethanol administration. General Pharmacology: The Vascular System (USA) 2000; 34(1)43–51
- Musabayane C.T., Forsling M.L., Balment R.J. Arginine vasopressin increases renal sodium excretion in the anesthetized rat through V1 receptors. Renal Failure (USA) 1997; 19(1)23–32
- Higaki K., Asai M., Suyama T., Nakayama K., Ogawara K., Kimura T. Estimation of intradermal disposition kinetics of drugs: II. Factors determining penetration of drugs from viable skin to muscular layer. International Journal of Pharmacology 2002; 239(12)129–141
- Karande P., Mitragotri S. High throughput screening of transdermal formulations. Pharmaceutical Research 2002; 19(5)655–660
- Sen A., Zhao Y., Zhang L., Hui S. Enhanced transdermal transport by electroporation using anionic lipids. Journal of Control Release 2002; 21(82 (2–3))399
- Kim N., El-Khalili M., Henary M.M., Strekowski L., Michniak B.B. Percutaneous penetration enhancement activity of aromatic S,S-dimethyliminosulfuranes. International Journal of Pharmacology 1999; 187(2)219–229