Abstract
Supramolecular aggregates can be aligned in solution using a magnetic field. Because of the optical anisotropy of the molecular building blocks, the alignment results in an anisotropic refractive index of the solution parallel and perpendicular to the magnetic field. We present a model for calculating the magnetic birefringence, using solely the magnetic susceptibilities and optical polarizabilities of the molecules, for any molecular arrangement. We demonstrate that magnetic birefringence is a very sensitive tool for determining the molecular organization within supramolecular aggregates.
Introduction
The fabrication of functional organic nanostructures is a topic of great current interest because of their potential applications in drug delivery systems [Citation1], nanoelectronics [Citation2], sensors [Citation3] and optical devices [Citation4]. The use of small organic molecules as building blocks that spontaneously self-assemble into nanostructures is very promising [Citation5], because of the relatively easy synthesis and large variety of the chemical structure and functionality of the molecules. To date, different structures have been made, ranging from spherical [Citation6] and cylindrical aggregates [Citation7] to nanometer thin wires [Citation8]. However, given a certain molecule, it is very difficult to predict the shape and structure of the aggregates formed, since chemical self-assembly is a complicated process, that depends not only on the molecules and their mutual interactions, but also on the experimental conditions, such as type of solvent, concentration and preparation method, which can greatly influence the supramolecular aggregate characteristics [Citation9].
To gain a better understanding of the self-assembly process, it is important to develop methods by which the molecular arrangement within aggregates can be resolved, preferably in solution. The molecular arrangement is usually determined on a substrate in the solid state, which has the drawback that the molecular structure might be influenced by the underlying substrate or by drying effects of the evaporating solvent [Citation10]. Here, we present a method of determining the molecular structure in solution using magnetic birefringence. Our method makes use of the fact that the organic molecules used for self-assembly are anisotropic, to achieve directionality in the intermolecular non-covalent interactions [Citation11]. Consequently, the magnetic and optical properties of the molecules are also anisotropic, which enables the magnetic alignment of aggregates, and the measurement of this alignment using birefringence. In this paper, we present a model that relates the magnetic birefringence to the molecular structure and shape of the aggregates.
Our model is an alternative formulation of Levy's method [Citation12], and describes the magnetic anisotropy in the refractive index of an aggregate solution, accounting for both the form birefringence caused by an anisotropic aggregate shape, as well as the intrinsic birefringence due to anisotropic optical polarizabilities. The only input parameters of our model are the molecular magnetic susceptibility and optical polarizability tensors, as opposed to Levy's model in which the anisotropic refractive index is described in terms of the anisotropic refractive indices of the objects in the mixture. Although the refractive index and the polarizability of an aggregate are related through its density, the relationship between the aggregate volume and the number of molecules involved is not always unambiguous, particularly for small aggregates such as those we consider here. It is, therefore more convenient to express the refractive index of the mixture in terms of molecular polarizabilities, which are often available in literature and can be readily calculated.
In this paper, we first discuss the energy of a diamagnetic molecule in a magnetic field, followed by a description of the resulting magnetic alignment and the induced refractive index of the aggregates in solution. We will illustrate the obtained expression with a theoretical example, demonstrating the two main contributions to the birefringence, and an experimental example on thiophene-based aggregates.
Molecule in magnetic field
The magnetic moment induced in a molecule in an applied magnetic field depends on the molar magnetic susceptibility
1 where the x′, y′ and z′-axes are the principle axes of the molecule (figure ). In general, the diamagnetic susceptibility of an organic molecule is given by the sum of all contributions of the different chemical groups [Citation13]. Since the susceptibility of most chemical groups is known [Citation14], it is relatively straightforward to calculate the susceptibility of the molecular building blocks.
Figure 1 Schematic representation of a molecule in a magnetic field. The (x′, y′, z′) axes represent the principle molecular axes that are rotated over the Euler angles θ and ψ with respect to the lab axes (x, y, z). The magnetic field is directed along the z-axis.
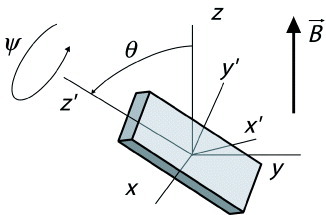
In an external magnetic field , the induced magnetic moment is
2 with μ0 being the permeability of free space and Na Avogadro's number. The energy related to the induced moment is given by
3 For anisotropic molecules, this energy depends on the orientation of the molecules with respect to the magnetic field direction. The transformation of the magnetic field applied in the z-direction to the molecular coordinate system leads to an expression of the magnetic energy ΔE as a function of the Euler angles θ and ψ (omitting the terms independent of the molecular orientation)
4 Note that the Euler rotation described by the angle φ represents a rotation about the magnetic field axis, which does not affect the magnetic energy of the molecule.
For individual molecules, ΔE is typically very small compared with the thermal energy kbT, and therefore it is not possible to align individual molecules with the static magnetic fields that are currently available (30–45 T). However, for an aggregate consisting of N molecules, the torque on the aggregate can be large enough to overcome the thermal randomization when NΔ E(θ, ψ) kb
T. In such a case, the degree of alignment is determined by a Boltzmann distribution function
5 Besides the magnetic alignment due to the anisotropy in the magnetic susceptibility, an alternative alignment mechanism due to demagnetizing effects exists for large aggregates of anisotropic shape [Citation15]. However, the energy involved in this process is typically several orders of magnitude lower than ΔE of equation (Equation4
4 ), and can therefore be safely neglected.
Refractive index of aligned aggregates
The magnetic orientation of aggregates leads to a difference in the refractive index of the solution parallel and perpendicular to the magnetic field. We calculate this magnetic birefringence incorporating two contributions: the intrinsic birefringence due to the anisotropic molecular polarizability [Citation16] and the form birefringence caused by an anisotropic aggregate shape [Citation17].
In the following, we limit ourselves to aggregates that are small with respect to the wavelength of light, which allows us to use an electrostatic approximation. We consider the solution as a host dielectric with refractive index n1 and we describe the aggregates as inclusions in this host medium. The electric field applied over the overall system can be expressed in terms of the field
in the inclusions and the field
in the host medium near a dielectric (figure ), using the effective medium theory [Citation17]
6 where f is the volume fraction of the aggregates, and the brackets 〈… 〉 denote an orientational average.
Figure 2 Schematic representation of the aggregates and the electric fields in solution. The (x″, y″, z″) coordinate system represents the aggregate axes. The magnetic field points in the z-direction.
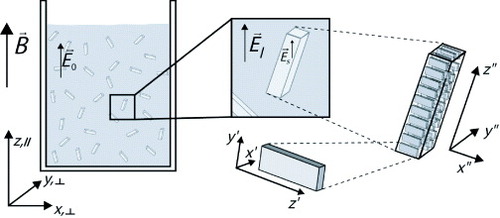
The effective electric field within the aggregate is lower than the field outside because of the form birefringence: the induced surface charges at an anisotropically shaped object reduce the electric field inhomogeneously. The shorter the aggregate is along a certain direction, the more the field will be reduced. The reduction describes the ratio between the field inside and outside the inclusion as
, with
7 and
8 where da,ii represents the depolarizing coefficients along the different aggregate axes (the axes with double prime indicate the principle axes of the aggregate), and nf is the average refractive index of the aggregate. Since the form birefringence usually has a small effect, a typical refractive index for conjugated organic molecules of nf≈1.6–1.7 [Citation18, Citation19] can be used without the need to calculate the exact refractive index for the aggregate configuration. For any shape, the depolarizing coefficients are tabulated or can be calculated numerically. For cylinder symmetric aggregates, the depolarizing factors can even be calculated analytically [Citation15].
Equation (Equation66 ) can be rewritten as
9 The denominator term accounts for the influence of an aggregate on the electric field experienced by the other aggregates. If nf> n1, the denominator term is larger than unity, and the effective field experienced by the aggregates is reduced.
The intrinsic birefringence is described in terms of the aggregate polarizability,
10 where α a,i″i″ is the polarizability along the principle axes of the aggregate and is obtained by spatial averaging of the molecular polarizabilities α a,i′i′ over the orientations of the molecules within the aggregate. The magnetic susceptibility of the aggregate χ i″i″ is obtained in a similar fashion.
The dipole moment induced in the aggregate is then
11 Since
, we find, for the dielectric constant of the mixture
12
This tensor expression for the dielectric constant contains three diagonal components: ε m, zz parallel to the magnetic field, and ε m, xx = ε m, yy perpendicular to the magnetic field. It includes both the form birefringence via and the molecular polarizabilities
, and also accounts for the fact that the effective electric field is reduced owing to the presence of the aggregates. The actual magnetic birefringence is now given by
13
Theoretical examples
We illustrate the roles of the two components of the birefringence, the form and the intrinsic birefringence, by two theoretical examples. In the first case, we consider a cylindrically symmetric situation where χ x″x″ = χ y″y″ ≪χ z″z″ and α x″x″ = α y″y″ < α z″z″, and we apply these parameters respectively to a cylindrical aggregate, a spherical aggregate and a disc-shaped aggregate. Figure shows the resulting birefringence together with a schematic picture of the aggregate in the high-field-limit orientation. In this situation, the aggregates align with their z″-axis, which is the axis of highest polarizability, parallel to the magnetic field. For the cylindrical aggregate, the birefringence is enhanced by the form birefringence compared with the spherical aggregate, since the effective polarizabilities α x″x″ and α y″y″ are reduced by the depolarization effect. The disc-shaped aggregate exhibits a reduced value of the birefringence because, now, the polarizability α z″z″ along the symmetry axis is diminished by the form birefringence.
Figure 3 Influence of the shape of the supramolecular aggregate in magnetic-field-induced birefringence. The corresponding depolarizing coefficients da, i″i″ are given in the figure legend. The aggregate shapes are drawn schematically in the energetically most favourable orientation when the magnetic field points upwards. The parameters used for the calculations are χ x″x″= χ y″y″= 4χ z″z″= - 4000 × 10- 12 m3 mol- 1, α x″x″= α y″y″= 0.5α z″z″= 200 au, C = 10- 3 M, f = 10- 4, n1= 1.4, nf= 1.6, N = 2 × 106 and T = 293 K.
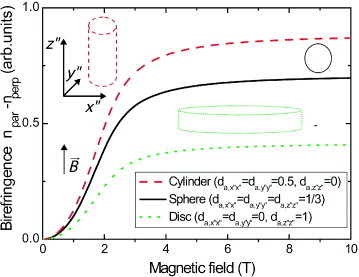
To illustrate the intrinsic birefringence, we consider disc-shaped aggregates composed of molecules with susceptibilities χ x′x′, χ y′y′ and χ z′z′ and polarizabilities α x′x′, α y′y′ and α z′z′, using typical parameters for π-conjugated oligomer molecules: χ x′x′ ≈ χ y′y′ ≫χ z′z′ and α x′x′ > α y′y′ > α z′z′. The magnetic birefringence depends on the way the molecules are oriented within the aggregates. Figure shows three simple configurations: a linear arrangement (top right panel, red solid line), a radial arrangement (middle right panel, green dashed line) and a herringbone arrangement (bottom right panel, blue dotted line). Because of the molecular orientation within the aggregate, we find α x″x″ = α y″y″ = 0.5α y′y′ + 0.5αz′z′ and α z″z″ = α x′x′ for the linear configuration, α x″x″ = α y″y″ = 0.5α x′x′ + 0.5αz′z′ and α z″z″ = α y′y′ for the radial configuration, and αx″x″=αz′z′ cos2η+αx′x′ sin2η, αy″y″=αz′z′ sin2η+αx′x′ cos2η and α z″z″ = α y′y′ for the herringbone configuration, where η is the herringbone angle of the molecules with the y″-axis. For the aggregate susceptibilities χ i″i″, we find similar expressions in terms of molecular susceptibilities χ i′i′.
Figure 4 Theoretical magnetic birefringence curves for disc shaped aggregates. Both the shape of the curve and the saturation value strongly depend on the molecular orientation within the aggregate. The three situations correspond to linear (solid red line), radial (dashed green line) and herringbone (dotted blue line) arrangements. The parameters used for the calculations are χ x′x′= χ y′y′=- 1000 × 10- 12 m3 mol- 1, χ z′z′=-4000 × 10- 12 m3 mol-1, αx′x′= 700 au, α y′y′= 400 au, α z′z′= 100 au, C = 10- 3 M, f = 10- 4, n1= 1.4, nf = 1.6, N = 2 × 106, dxx= dyy= 0, dzz= 1, T = 293 K and herringbone angle η = 65°.
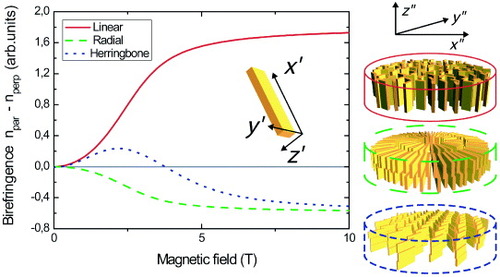
All three configurations align with their symmetry axis along the magnetic field, with the molecular z′-axis perpendicular to the magnetic field. For the linear configuration, we find a positive birefringence because the molecules align with their highest polarizability axis (x′) parallel to the magnetic field. For the radial arrangement, the molecular y′-axis is parallel to the magnetic field, resulting in a negative birefringence. The absolute value of the birefringence is lower than that in the linear case because the molecular x′-axis is allowed to rotate about the magnetic field axis. The herringbone arrangement results in a two-step alignment process that gives rise to the peculiar shape of the birefringence curve. In high fields, the birefringence is equal to the radial aggregate, because, in both cases, the molecules align with the y′-axis parallel to the magnetic field. However, the initial birefringence is positive since the orientation with the x″-axis parallel to the magnetic field is slightly more favorable than the y″-axis being parallel to B. This two-step alignment process is caused by an anisotropy in the magnetic susceptibility along the x″-axis and y″-axis, and clearly demonstrates the high sensitivity of magnetic birefringence for the internal molecular arrangement of supramolecular aggregates.
Experimental example
We have recently reported the formation of spherical structures built of α,α′-linked sexithiophene (T6) molecules with chiral oligo(ethyleneoxide) side chains at both the α- and ω-positions [Citation6]. The spherical aggregates were prepared by solving T6 molecules in tetrahydrofuran, and subsequent injection into isopropanol. It was found that changing the solvent from isopropanol to decanol while keeping all other experimental conditions the same greatly influenced the shape, size and sign of the magnetic birefringence curves (figure ). These results strongly suggest that the internal structure of the aggregates strongly depends on the solvent. The birefringence signal of the T6/isopropanol solution is very large and positive, whereas T6/decanol exhibits a very small signal that changes from positive to negative at a magnetic field strength of 3 T.
Figure 5 Magnetic-field-induced birefringence of T6 aggregates and the theoretical prediction for two different solvents. The difference in alignment indicates a different internal molecular arrangement. The parameters used for the calculations are χ x′x′= χ y′y′=- 3063 × 10- 12 m3 mol - 1, χz′z′= - 6813×10-12 m3 mol-1, α x′x′= 772 au, α y′y′= 313 au, α z′z′= 93 au, C = 8.24 × 10- 4 M, f = 4.3 × 10- 4, n1= 1.4, nf= 1.6, Nisopropanol = 5 × 105, Ndecanol= 1.5 ×105, T = 293 K and herringbone angle η = 62 °.
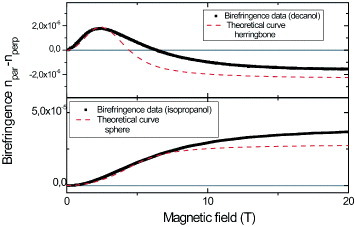
It is beyond the scope of this work to include elasticity in the calculation for the birefringence of the spherical aggregates in isopropanol [Citation20]. Instead, we approximate the maximum birefringence for this case by assuming that all T6 molecules are oriented with their long axis parallel to the magnetic field. This corresponds to the limiting situation of a completely deformed sphere. In this case, we find the maximum calculated birefringence of 3×10- 5 at 20 T, which is close to the experimental value of 3.7×10- 5, when using diamagnetic susceptibilities from the addition scheme [Citation21] and the polarizabilities given by Champagne et al [Citation22]. Given the uncertainties in these values, the agreement between the theoretical and experimental values is actually very good.
As was shown above (figure ), birefringence changing its sign with the field, as was observed for the decanol solution, only occurs for a very specific combination of aggregate polarizabilities and susceptibilities. The measured birefringence is well described by a herringbone structure in a cylindrical aggregate, which is probably the most widely encountered structure for linear conjugated oligomers [Citation23]. Additional experiments are required to verify the internal structure, but the birefringence results can exclude many possible configurations and puts serious constraints on the structure.
Conclusions
We have presented a model for calculating the magnetic-field-induced birefringence of a solution of supramolecular aggregates. The measured signal depends strongly on the shape of the aggregates (form birefringence) and the molecular orientation within the aggregates (intrinsic birefringence). We have shown some theoretical examples illustrating the combined effects of the form and intrinsic birefringence, and we have applied the model to supramolecular self-assemblies of sexithiophene molecules. The data on this system clearly suggest a herringbone molecular arrangement, which is one of the most frequently found structures of this type of molecular aggregates, although additional experiments are required to verify this result. Since the model gives a semiquantitative description, the alignment of supramolecular aggregates is a very useful technique for determining the internal molecular arrangement of supramolecular aggregates in solution. This can provide information that could not be obtained by other methods, giving a valuable contribution towards an improved understanding on the formation of supramolecular aggregates.
Acknowledgements
We thank P Jonkheijm, O Henze, A F M Kilbinger and W J Feast for synthesizing and supplying the T6 molecules.
References
- RöslerAVandermeulenG W MKlokH-A 2001 Adv. Drug Deliv. Rev. 53 95 http://dx.doi.org/10.1016/S0169-409X(01)00222-8
- TsengR JHuangJOuyangJKanerR BYangY 2005 Nano Lett. 5 1077 http://dx.doi.org/10.1021/nl050587l
- HuangJVirjiSWeillerB HKanerR B 2003 J. Am. Chem. Soc. 125 314 http://dx.doi.org/10.1021/ja028371y
- BersonSDe BettigniesRBaillySGuillerezS 2007 Adv. Funct. Mater. 17 1377 http://dx.doi.org/10.1002/adfm.200600922
- WhitesidesG MBonchevaM 2002 Proc. Nat. Acad. Sci. 99 4769 http://dx.doi.org/10.1073/pnas.082065899
- ShklyarevskiyI OJonkheijmPChristianenP C MSchenningA P H JMeijerE WKilbingerA F MFeastW JGuerzoA DDesvergneJ-PMaanJ C 2005 J. Am. Chem. Soc. 127 1112 http://dx.doi.org/10.1021/ja0431096
- WangZMedforthC JShelnuttJ A 2004 J. Am. Chem. Soc. 126 15954 http://dx.doi.org/10.1021/ja045068j
- JeukensCJonkheijmPWijnenF J PGielenJ CChristianenP C MSchenningA P H JMeijerE WMaanJ C 2005 J. Am. Chem. Soc. 127 8280 http://dx.doi.org/10.1021/ja051781c
- JonkheijmPvan der SchootPSchenningAMeijerE W 2006 Science 313 80 http://dx.doi.org/10.1126/science.1127884
- Van HamerenR et al 2006 Science 314 1433 http://dx.doi.org/10.1126/science.1133004
- HoebenF J MJonkheijmPMeijerE WSchenningA 2005 Chem. Rev. 105 1491 http://dx.doi.org/10.1021/cr030070z
- LevyO 2002 Phys. Rev. E 66 011404 http://dx.doi.org/10.1103/PhysRevE.66.011404
- MaretGDransfeldK 1985 Strong and Ultrastrong Magnetic Fields and Their Application F Herlach Berlin Springer p 143
- GuptaR R 1986 Diamagnetische Suszeptibilität (Landolt Börnstein . Gruppe II, Atom- und Molekularphysik) vol. 16 R RGupta Berlin Springer
- OsbornJ A 1945 Phys. Rev. 67 351 http://dx.doi.org/10.1103/PhysRev.67.351
- BuckinghamA DPopleJ A 1956 Proc. Phys. Soc. B 69 1133 http://dx.doi.org/10.1088/0370-1301/69/11/311
- BraggW LPippardA B 1953 Acta Crystallogr. 6 865 http://dx.doi.org/10.1107/S0365110X53002519
- TammerMMonkmanA P 2002 Adv. Mater. 14 210 http://dx.doi.org/10.1002/1521-4095(20020205)14:3<210::AID-ADMA210>3.0.CO;2-2
- TakazawaK 2007 J. Phys. Chem. C 111 8671 http://dx.doi.org/10.1021/jp071455e
- ManyuhinaO V et al 2007 Phys. Rev. Lett. 98 146101 http://dx.doi.org/10.1103/PhysRevLett.98.146101
- SutterD HFlygareW H 1969 J. Am. Chem. Soc. 91 4063 http://dx.doi.org/10.1021/ja01043a007
- ChampagneBMosleyD HAndreJ M 1994 J. Chem. Phys. 100 2034 http://dx.doi.org/10.1063/1.466555
- GavezzottiAFilippiniG 1991 Synth. Met. 40 257 http://dx.doi.org/10.1016/0379-6779(91)91781-5