Abstract
Mn-90.4 wt% Sb alloy specimens were solidified under both uniform magnetic field and magnetic field gradient conditions. The solidification behavior was examined to elucidate the effects of high magnetic fields on the solidified structure evolution of this hypoeutectic alloy. The macrostructures on the longitudinal section of the alloys were investigated by optical microscopy and x-ray diffraction (XRD). The volume fraction of primary MnSb phases and the interrod spacing of the eutectic were measured by metallographic analysis. It was found that the segregation of the primary MnSb particles at the certain regions of the specimens occurred under the influence of high magnetic field gradients. The MnSb phases obtained under magnetic fields were oriented with their (h0 l) planes along the direction of the magnetic field. Both the volume fraction of primary MnSb phases and the interrod spacing of the eutectic were decreased upon the application of the high magnetic fields.
Introduction
The control of the solidification conditions of metallic materials to improve their properties is of importance in many industrial processes [Citation1, Citation2]. The solidification process under high magnetic field conditions has attracted widespread interest from both fundamental and practical viewpoints, because of the attractive nature of magnetic fields for such processing. At high magnetic field intensities, even nonmagnetic materials, such as para- or diamagnetic materials, have shown obvious responses to the field. For example, utilizing magnetic force, a great number of nonmagnetic materials with a wide range of dimensions, such as water, glass, organic materials and even alloy melts, have been successfully levitated [Citation2–5]. This provides the possibility of controlling the solidified structures of the materials using high magnetic fields. It is widely recognized that a high magnetic field can exert three effects on a system, that is, Lorentz force, magnetic force and magnetization. On the basis of these three effects, investigations on non- or unidirectional solidification under high magnetic fields have shown that high magnetic fields can induce aligned microstructures [Citation6–8]; change the equilibrium partition coefficient [Citation9, Citation10], phase transformation temperature [Citation11, Citation12] and the distribution of solute elements [Citation13, Citation14]; decrease the diffusion coefficient [Citation15, Citation16]; and induce thermoelectromagnetic convection [Citation17, Citation18].
In this study, the effects of high magnetic fields on solidified microstructures, such as crystal orientation and macrosegregation, have been investigated. A binary eutectic system, Mn–Sb alloy, is an ideal candidate, since there is a significant difference in the volume susceptibility of the alloying elements (Mn and Sb) in liquid states. The mechanism of the high magnetic field effect on the solidified structure was also briefly discussed.
Experimental details
Mn-90.4 wt% Sb alloy was prepared by the vacuum–induction–melting method by mixing Mn and Sb of high 4N purity in a graphite crucible. The obtained ingot was then machined into cylindrical specimens of 9.5 mm diameter and 15 mm length. A specimen was inserted into an alumina crucible (inner diameter 10 mm; outer diameter 15 mm), heated to 1123 K under an argon atmosphere at a heating rate of 5 K min−1 and held for 30 min to ensure its homogeneity. The temperature was cooled to 913 K at a cooling rate of approximately 6 K min−1, and then to 773 K at a cooling rate of approximately 1.4 K min−1. After that, the specimen was cooled to room temperature by turning off the dc power source. The solidification experiment was repeated in the presence of an 11.5 T uniform magnetic field and two magnetic field gradients of ±282 T2 m−1. The details of the experimental apparatus have been reported previously [Citation14]. The magnetic fields were imposed and removed when the temperature was 677 K, which was approximately 90 K higher than the Curie temperature (587 K) of the MnSb phases in the alloys. The as-solidified structures of the specimens were examined by optical microscopy and x-ray diffraction (XRD, CuKα radiation) analysis on the longitudinal section, which was parallel to the direction of the imposed magnetic field. Energy dispersive x-ray (EDX) analysis was performed to characterize the chemical compositions of the phases in the specimens. The area fraction of the primary MnSb dendrites and the interrod spacing of the eutectics were measured by image analysis techniques. The temperature/time-dependent curves were obtained by inserting a Pt-13% Rh thermocouple, which was contained in an alumina sheath (inner diameter 2 mm; out diameter 3 mm), into the bottom of the crucible, as shown in figure .
Results and discussion
Distribution of primary MnSb phases
Figure shows the macrostructures of Mn-90.4 wt% Sb alloy specimens solidified under various magnetic field conditions. By EDX analysis, the dark and bright phases were identified to be MnSb and Sb, respectively. Due to the hypoeutectic composition of the alloys, primary MnSb dendrites are found to be distributed in a matrix of eutectic MnSb and Sb for all magnetic field conditions, although large holes are also observed. However, the distribution of these primary MnSb dendrites shows various features when the samples are subjected to different magnetic field conditions. At 0 T, the primary MnSb dendrites are found to segregate slightly in the upper region of the specimen. In the case of 11.5 T, the primary MnSb dendrites are distributed relatively uniformly throughout the specimen. In the presence of a magnetic field gradient, the primary MnSb dendrites again exhibit strong segregation, and the position where they segregate strongly depends on the direction of the magnetic field gradient. The statistical measurements of the area fraction of the primary MnSb dendrites throughout the longitudinal section (figure ) confirm these segregations.
Figure 2 Macrostructures of Mn-90.4 wt% Sb alloy specimens solidified under various magnetic field conditions. (a) B=0 T; (b) B=11.5 T; (c) B=11.5 T, BdB/dz=−282 T2 m−1, (d) B=11.5 T, BdB/dz=+282 T2 m−1.
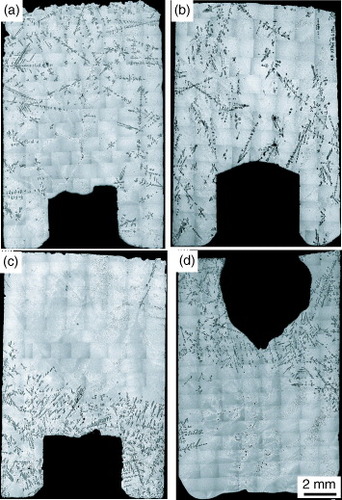
Figure 3 Area fraction distributions of the primary MnSb dendrites along the depth from the top surface in the specimens solidified under various magnetic field conditions.
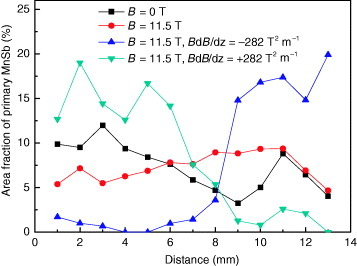
Since the density of alloying element Sb is larger than that of Mn, the crystallized segregation of primary MnSb dendrites would occur at the top of the crucible without a magnetic field. It has traditionally been suggested that high magnetic field can significantly suppress the convection in the melt during the solidification process because of Lorentz force induced by the relative movement between the electrically conducting materials and the magnetic field. It, in turn, can also act on the moving primary MnSb particles, resulting in a counteraction of the movement. Therefore, the phenomenon of the relatively uniform distribution of the primary MnSb dendrites at 11.5 T can be attributed to the inhibitory effect of the magnetic field on the convection of the melt as well as on the sedimentation of the primary MnSb dendrites. Wang et al [Citation14], on the basis of a study using a magnetic field gradient, have suggested that a magnetic force may drive the clusters of Mn in a hypoeutectic Mn–Sb alloy to move along the direction of force if the magnetic flux density is sufficiently high and the field gradient is sufficiently steep. Furthermore, Liu et al [Citation19] observed that the movement of MnSb particles could also be driven by the magnetic force under a high magnetic field gradient. Therefore, the segregation of the primary MnSb dendrites, observed in the presence of magnetic field gradients, could be a consequence of the effects of the magnetic force on the Mn clusters (before the solidification begins) or on MnSb crystals (during the solidification process) or both.
In all specimens solidified at 0, 11.5 and 11.5 T with a gradient of −282 T2 m−1, there is a large hole on the bottom of the specimens (figures (a)–(c)), whereas the large hole appears in the upper region of the specimen in the case of the field gradient of +282 T2 m−1 (figure (d)). These holes are related to the configuration of the assembly for thermal analysis, as shown in figure , where the bottom of crucibles protruded inward in order to contain the thermocouple. In order to fit the shape of the crucible, holes were drilled into the bottom of the specimens before the solidification experiments. These drilled holes were remained after re-melting and solidifying at 0 T, 11.5 T and a negative field gradient, since the specimens were acted on gravity or the combination of gravity with downward magnetic force. On the contrary, the hole was forced to move towards the top surface of the specimen owing to the surface tension when the specimen was subjected to a positive field gradient such that it was in a levitated state as a result of the upward magnetic force acting on it.
Average area fraction of primary MnSb phases
The measurement results of the average area fraction of the primary MnSb phase on the longitudinal section are shown in figure . It is clear that the application of high magnetic fields decreased the average area fraction of the primary MnSb phase, and magnetic field gradients had no greater effect than uniform ones on the average area fraction. Supposing that a paramagnetic material is magnetized in a magnetic field, the energy needed for the magnetization of unit volume of the material is given by
1 where μ0 is the magnetic constant, χ is the magnetic susceptibility, N is the demagnetization factor and H is the magnetic field. From equation (Equation1
1 ), it can be found that the larger magnetic susceptibility of a material corresponds to a lower magnetic energy. The MnSb phase is ferromagnetic at room temperature, while it will transform to become paramagnetic above its Curie temperature, particularly near the melting temperature, and show an extremely small magnetic susceptibility, χMnSb. In addition, the Mn element has the largest value of magnetic susceptibility in liquid, χMn (882.62×10−6 [Citation14]), compared with other elements. It can thus be deduced that the value of χMnSb near the melting temperature is smaller than that of χliquid, the susceptibility of the remaining liquid that consists of Mn and Sb. That is, the magnetic energy of the MnSb crystal is higher than that of the remaining liquid. According to equation (Equation1
1 ), the magnetization energy of the MnSb phase, UMnSb, will be higher than that of the remaining liquid, Uliquid. In this case, the decrease in the volume faction of the primary MnSb phases will contribute to reducing the systematic magnetic energy, which stabilizes the system. Therefore the average area fraction of the primary MnSb dendrites was decreased under the influence of high magnetic fields during the hypoeutectic reaction. Obviously, the decrease in volume of the primary phase in a eutectic system should be accompanied by a decrease in the interval of the primary reaction, which may be a consequence of the decrease of the liquidus or the increase of the eutectic temperature or both. Furthermore, some thermal analysis experiments with Mn–Sb mother alloys of various compositions have been carried out using a modified assembly, and have yielded more accurate results rather than those in this work. Although the measurement results suggest an increase in the eutectic temperature, which should result in a decrease in the interval of the primary reaction, the variation of the liquidus has not been confirmed (this will be further discussed elsewhere).
Orientation of MnSb crystals
To investigate the orientation of MnSb crystals in the alloys, x-ray diffraction analysis was performed on the longitudinal section parallel to the magnetic field direction. The XRD patterns of the specimens solidified at 0, 11.5 and 11.5 T with the gradient of −282 T2 m−1 are shown in figure , in which only the index of MnSb phases is included. In figure , it is evident that the peaks of (101) and (103) planes, which are strong in the specimen without a magnetic field, are considerably depressed in the specimen with magnetic fields, while the peaks of (002) and (004) planes are strongly enhanced. Considering the crystal structure of the MnSb phase, i.e. a hexagonal structure, it can be concluded that the MnSb crystals are oriented with their c-plane parallel to the magnetic field direction.
Figure 5 XRD patterns of MnSb in the plane parallel to the magnetic field direction under various magnetic field conditions. (a) B=0 T; (b) B=11.5 T; (c) B=11.5 T, BdB/dz=−282 T2 m−1.

It is well known that when a crystal with an anisotropic magnetic susceptibility is subjected to a magnetic field, it tends to rotate itself to an angle that minimizes the system energy. A possible explanation for the orientation of the MnSb phases is that the magnetic fields cause MnSb crystals to rotate to the position at which their c-plane is parallel to the magnetic field direction, so as to assume a lower energy state during the solidification process. This should be further confirmed because the anisotropic property of the MnSb phase near or above its melting temperature has not yet been clarified.
Microstructure morphology of eutectics
Figure shows the microstructures of eutectics in the specimens solidified under various magnetic field conditions. The microstructures in all cases show a rodlike morphology of the MnSb–Sb eutectic, in which MnSb rods are well embedded in a Sb matrix. The application of high magnetic fields is found to alter the morphology of MnSb, as an increase of the rod density and a decrease of the rod size. However, the magnetic field gradients do not show greater effects than the uniform field on the morphology of MnSb. The measurement results of interrod spacing (figure ) show decreases in the interrod spacing under high magnetic field conditions and confirm the increase in rod density.
Figure 6 Typical microstructures of eutectics under various magnetic field conditions. (a) B=0 T; (b) B=11.5 T; (c) B=11.5 T, BdB/dz=−282 T2 m−1, (d) B=11.5 T, BdB/dz=−282 T2 m−1.
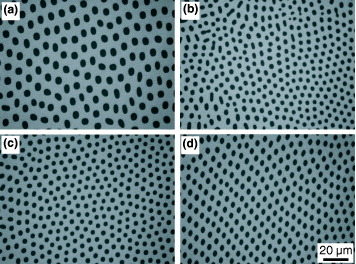
It has already been suggested that the eutectic growth is a diffusion-controlled process. Any change in the diffusion behavior at the liquid–solid interface would lead to a eutectic spacing alteration. During the eutectic growth without a high magnetic field, the strong thermal convection in the melt enhances the diffusion rate of the solute elements away from the liquid–solid interface and thus results in a larger eutectic spacing and size of the embedded phase. As discussed above, high magnetic fields can significantly suppress the convection caused by the temperature gradient or the density gradient. The studies focusing on the effect of the magnetic field on the diffusion in a liquid have also suggested that this suppression of convection can significantly decrease the diffusion coefficient [Citation15]. That is, the application of a high magnetic field should reduce the eutectic spacing and the size of the embedded phase by decreasing the diffusion of solute at the liquid–solid interface. Therefore, under high magnetic field conditions, it was found that both the interrod spacing and the rod size of the eutectic MnSb were decreased (figures and ).
Conclusions
The Mn-90.4 wt% Sb alloy specimens were solidified under both uniform magnetic field and magnetic field gradient conditions. The effects of high magnetic fields on the solidified structure of Mn-90.4 wt% Sb alloy were investigated. The macrostructures on the longitudinal section of the alloys were examined by optical microscopy and x-ray diffraction. It was found that the application of high magnetic field gradients caused the segregation of the primary MnSb particles at certain regions of the specimens. The MnSb phases obtained under magnetic fields were oriented with their (h0 l) planes along the direction of magnetic field. Both of the volume fraction of the primary MnSb phase and the interrod spacing of the eutectic were decreased upon the application of high magnetic fields.
Acknowledgments
This work was supported by the National Natural Science Foundation of China (Grant No. 50374027), the Program for New Century Excellent Talents in University, PR China (Grant No. NCET-06-0289), the 111 project (Grant No. B07015) and the Liaoning BaiQianWan Talents Program.
References
- BoettingerW JCoriellS RGreerA LKarmaAKurzWRappazMTrivediR 2000 Acta Mater. 48 43 http://dx.doi.org/10.1016/S1359-6454(99)00287-6
- LiuFYangG C 2006 Int. Mater. Rev. 51 145 http://dx.doi.org/10.1179/174328006X102484
- BeaugnonETournierR 1991 Nature 349 470 http://dx.doi.org/10.1038/349470a0
- WeilertM AWhitakerD LMarisH JSeidelG M 1996 Phys. Rev. Lett. 77 4840 http://dx.doi.org/10.1103/PhysRevLett.77.4840
- VallesJ MLinKDenegreJ MMowryK L 1997 Biophys. J. 73 1130 http://dx.doi.org/10.1016/S0006-3495(97)78145-1
- WangC JWangQWangZ YLiH TNakajimaKHeJ C 2008 J. Cryst. Growth 310 1256 http://dx.doi.org/10.1016/j.jcrysgro.2007.12.045
- MorikawaHSassaKAsaiS 1998 Mater. Trans. JIM 39 814
- YasudaHOhnakaIYamamotoYTokiedaKKishioK 2003 Mater. Trans. JIM 44 2007 http://dx.doi.org/10.2320/matertrans.44.2007
- YoudelisW VDorwardR C 1966 Can. J. Phys. 44 139
- YenC TTillerW A 1992 J. Cryst. Growth 118 85 http://dx.doi.org/10.1016/0022-0248(92)90052-K
- LiXRenZ MFautrelleY 2007 Intermetallics 15 845 http://dx.doi.org/10.1016/j.intermet.2006.10.048
- LiXRenZ MFautrelleY 2007 J. Cryst. Growth 299 41 http://dx.doi.org/10.1016/j.jcrysgro.2006.10.249
- WangQPangX JWangC JLiuTLiD GHeJ C 2007 Mater. Sci. Forum 539–543 457 http://dx.doi.org/10.4028/0-87849-428-6.457
- WangQLiuTGaoAZhangCWangC JHeJ C 2007 Scr. Mater. 56 1087 http://dx.doi.org/10.1016/j.scriptamat.2007.02.026
- BottonVLehmannPBolcatoRMoreauRHaettelR 2001 Int. J. Heat Mass Transfer 44 3345 http://dx.doi.org/10.1016/S0017-9310(00)00362-8
- TomaruTFujiiKOhyamaTOtsukaE 1990 Phys. Rev. B 42 9104 http://dx.doi.org/10.1103/PhysRevB.42.9104
- GorbunovL A 1987 Magnetohydrodynamics 23 404
- WangQLiD GWangKWangZ YHeJ C 2007 Scr. Mater. 56 485 http://dx.doi.org/10.1016/j.scriptamat.2006.11.026
- LiuTWangQGaoAZhangCWangC JHeJ C 2007 Scr. Mater. 57 992 http://dx.doi.org/10.1016/j.scriptamat.2007.08.011