Abstract
A simple two-step aqueous polymerization method was introduced to synthesize a polyacrylate/polyethylene glycol (PAC/PEG) interpenetrating network (IPN) hydrogel. On the basis of the effects of the ratio of PAC to PEG, neutralization degree, heavy-metal ion concentration, and temperature on the adsorption behavior of PAC/PEG IPN hydrogel toward Ni2 +, Cr3 + and Cd2 +, the preparation conditions were optimized. In our system, the greatest amount of Ni2 +, Cr3 + and Cd2 + adsorbed were 102.34, 49.38 and 33.41 mg g- 1, respectively. The adsorption abilities of a dried PAC/PEG composite and a swollen PAC/PEG IPN hydrogel were compared. It was found that the efficiency of removing metal ions using the swollen hydrogel was greater than that using the dried composite. The adsorption mechanism and model are also discussed.
Introduction
According to the World Health Organization, the heavy-metals of most immediate concern are chromium, nickel, cadmium, copper, lead, mercury and zinc. The removal of heavy-metal ions from industrial and human wastewater is attracting increased attention. Conventional treatment techniques for removing dissolved heavy-metal ions from wastewater by chemical treatment, ultrafiltration or their combination do not always sufficiently remove contaminants to meet pollution control limits [Citation1–4]. Recently, many types of hydrogel such as poly(acrylic acid), poly(acrylic acid) grafted cellulose and macroporous poly(glycidyl methacrylate-co-ethylene glycol dimethacrylate) have been reported for the removal of Cu(II), Cd(II) and Cr(III) under competitive conditions [Citation5–7].
A superabsorbing hydrogel is a slightly cross-linked hydrophilic polymer with a three-dimensional network structure [Citation8, Citation9]. It has a porous structure and many hydrophilic groups, which can be used for heavy-metal ion removal. Owing to their unique properties, hydrogels have been used in drug delivery, batteries and tissue engineering [Citation10–12]. In this study, a polyacrylate/polyethylene glycol (PAC/PEG) hydrogel with an interpenetrating network (IPN) structure was synthesized by a simple two-step aqueous solution polymerization. The synthetic pathway of the PAC/PEG IPN hydrogel is shown in equation (Equation11 ) (acrylic acid and acrylate are denoted AA and AC, respectively). Polymerization takes place in step I to form a PAC prepolymer. Then, copolymerization between the PAC prepolymer and PEG occurs in step II to form an IPN structure [Citation13, Citation14].
1 Using the PAC/PEG IPN hydrogel, the effects of synthesis parameters, target ion concentration and ambient temperature on the adsorption ability of the hydrogel for heavy-metal ions are investigated. Also, the adsorption abilities of a dried PAC/PEG composite and a swollen PAC/PEG IPN hydrogel are compared, and the adsorption mechanism and model are discussed in this paper.
Experimental
Materials
An acrylic acid (AA) monomer was distilled under reduced pressure prior to use. Polyethylene glycol (PEG, Mn= 20 000) was used as received. Ammonium persulfate (APS) as a radical initiator for the synthesis of PAC was purified by recrystallization from 66 wt.% ethanol/water solution. N,N′-methylene bisacrylamide (NMBA) was used as a cross-linker for the preparation of PAC. Potassium hydroxide was used to neutralize the AA monomer. Ni2 +, Cr3 + and Cd2 + from NiCl2, CrCl3 and Cd(NO3 )2 solutions, respectively, were used as target toxic ions. Ethylenediaminetetraacetic acid (EDTA) was used as a common indicator. All the above materials were purchased from Shanghai Chemical Reagents Co., China. Each solution was prepared using distilled water. All aqueous solutions were prepared in 18 MΩ water obtained by purifying deionized water with a Millipore Milli-Q system.
Preparation of PAC/PEG IPN composite
A solution of AA and acrylate (AC) monomers was prepared by agitating AA (15 g) and potassium hydroxide of the necessary concentration in distilled water (15 ml) at ambient temperature. Under a nitrogen atmosphere, the solution was stirred and heated to 80 °C in a water bath for 10 min, and then the initiator (APS, 0.045 g) was added to the reaction mixture. The polymerization reaction mixture was stirred for 10 min to prepare PAC prepolymer solutions.
Another solution (10 ml) of PEG and the cross-linker (PEG 2.5 g, NMBA 0.006 g) was poured into each PAC prepolymer solution, and the initiator (APS, 0.045 g) was added. Under a nitrogen atmosphere, the solution was stirred until the dilute reactant solution was ropy. Then the reactant was cooled to room temperature for about 30 min to allow copolymerization to take place. Half of the product was washed with distilled water and then dried under vacuum at 80 °C for more than 12 h until it reached a constant weight to remove low-molecular-weight polymers and impurities. After milling and sifting using a 40-mesh screen, a powdered PAC/PEG composite was obtained.
Heavy-metal ion adsorption
Adsorption agent samples (dried PAC/PEG composite or swollen PAC/PEG IPN hydrogel) were immersed in 0.03 M NiCl2, CrCl3 and Cd(NO3 )2 solutions. The residual solutions were analyzed to determine the concentration of rejected ions by a spectrophotometrical method using EDTA and a UV-vis spectrophotometer (Shimadzu Corporation, Japan) [Citation15]. The amount of adsorbed heavy-metal ions was determined using
2 where C0 and Ct are the concentrations (mg L- 1) of heavy-metal ion at the beginning and after t min in solution, respectively, V is the solution volume (L) and m is the mass of dried PAC/PEG composite (g).
Characterization
The swollen PAC/PEG IPN hydrogel samples in the equilibrium swelling state were quickly frozen in liquid nitrogen and then freeze-dried under vacuum at -42 °C for 3 days until all the water sublimed. The freeze-dried hydrogel was fractured carefully, and its morphology was studied by scanning electron microscopy (SEM, Hitachi S-5200, Japan). The hydrogel specimens were fixed on aluminum stubs and coated with gold for SEM [Citation16].
The hydrogel samples were analyzed using a Nicolet Impact 410 Fourier transform infrared (FTIR) spectrophotometer in the region of 500–3700 cm- 1. Prior to the measurement, the hydrogel samples were dried under vacuum overnight until they reached a constant weight. The dried hydrogel was pressed into a powder, mixed with 10 times as much KBr powder and then compressed into a pellet for characterization by FTIR spectroscopy.
Thermogravimetric analysis (TGA) was conducted using a TA TGA 2050 instrument to monitor the weight change of the as-obtained samples. The experimental procedure consisted of packaging the sample using an aluminum foil and followed by heating the samples in flowing nitrogen (99.999% purity, 100 ml min- 1) at a rate of 15 °C min- 1 from room temperature to 650 °C until all the material was completely consumed.
Results and discussion
Characterization
Figure shows SEM images of the PAC/PEG IPN hydrogels. The hydrogels exhibit regular netlike structures. No phase separation is observed in the SEM images, which implies the formation of a complete IPN structure consisting of a PAC network and PEG chains. Figure (b), which is a high magnification of part of figure (a), shows a connective, oriented and even porous structure, which is expected to have infiltration, adsorption, swelling and drug release properties.
The FTIR spectra of PAC, PEG, PAC/PEG–Ni, PAC/PEG–Cr and PAC/PEG–Cd are shown in figure . The bands of PAC are observed at 3635 cm- 1 (O–H stretching), 1690 cm- 1 (C=O stretching), 2923 cm- 1 (C–H bending) and 1319 cm- 1 (C–C stretching). In the PAC/PEG composite, a peak at 1109 cm- 1 due to the C–O–C stretching vibration of PEG is observed, which indicates that PEG has been introduced into the composite.
Figure 2 FTIR spectra of PAC (a), PAC/PEG–Cr (b), PAC/PEG–Cd (c), PAC/PEG–Ni (d) and PEG 20 000 (e).
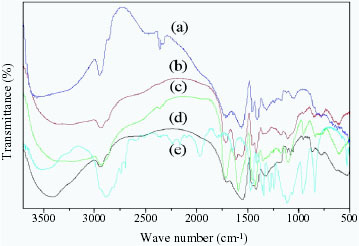
The peaks at 1570 and 1407 cm- 1 represent C=O bending in the –COOH and –COOK groups of PAC, respectively. These two peaks are shifted to 1555, 1614 and 1592 cm- 1 and to 1418, 1418 and 1424 cm- 1 for PAC/PEG–Ni, PAC/PEG–Cr and PAC/PEG–Cd complexes, respectively. The peaks at 1250 and 1456 cm- 1, attributed to O–H distortions upon –COOH and –COO- stretching, respectively, disappear in the corresponding ion composites, which indicates the chelation of –COOH/–COO- groups with Ni2 +, Cr3 + and Cd2 +.
Effect of PAC/PEG mass ratio on heavy-metal ion adsorption
The effect of PAC/PEG mass ratio on the adsorption of heavy-metal ions is shown in figure . It is clear that the amount of adsorbed heavy-metal ions decreased quickly with decreasing PAC/PEG mass ratio. In the PAC/PEG IPN hydrogel, PEG is a hybrid agent for constructing the IPN structure. The –O– and –OH groups on the PEG chains have some coordination ability and electrostatic affinity to heavy-metal ions, which however are less than those of the –COOH and –COOK groups in the PAC network. Therefore, a greater amount of PAC in the PAC/PEG hydrogel is favorable for improving the adsorption ability of the hydrogel for heavy-metal ions. In our experiments, to obtain an optimal IPN structure and high adsorption ability, PAC/PEG mass ratio is fixed at 6.
Effect of neutralization degree on heavy-metal ion adsorption
The effect of the neutralization degree of the AA monomer on Ni2 + sorption value is shown in figure (a). Ni2 + sorption value increases with increasing neutralization degree. In fact, the PAC polymer backbone contains –COO- and –COOH groups, and –COO -/–COOH ratio increases with increasing neutralization degree. Because the coordination ability and electrostatic affinity of the –COO - group to Ni2 + are higher than those of the –COOH group, Ni2 + sorption value increases with increasing neutralization degree. The same effect of neutralization degree on the sorption values of Cr3 + and Cd2 + by the PAC/PEG IPN hydrogel is shown in figure (b).
Effect of target ion concentration on adsorption
Ion concentration is another parameter affecting the adsorption ability of the PAC/PEG IPN hydrogel. Figure shows the effect of Ni2 + concentration on Ni2 + sorption value. For low Ni2 + concentrations, the Ni2 + sorption value increases rapidly with increasing Ni2 + concentration. When the Ni2 + concentration is higher than 0.1 M, the Ni2 + sorption value remains constant. Giles et al have classified the adsorption isotherms in solution into four types: S (shape), L (Langmuir), H (high affinity) and C (constant partition) [Citation17]. From figure , it can be seen that the isotherm of the adsorption of Ni2 + by the PAC/PEG IPN hydrogel is of the L-type. This means that many adsorption sites are distributed in the hydrogel. For higher concentrations, it is easier for Ni2 + to reach its adsorption site, while it becomes increasingly difficult for Ni2 + to reach the sites with the adsorption. There are two causes of the formation of L-type isotherms: the arrangement of the adsorbate in the adsorbent is not vertical and there are no competing adsorptions by the adsorbate and solvent on the adsorbent surface [Citation17]. In our system, Ni2 + forms a five- or six-membered ring structure with active sites as the most stable state. Once Ni2 + coordinates with the PAC/PEG IPN hydrogel, it loses its activity. Thus, for the above two reasons an L-type isotherm was obtained.
Effect of temperature on heavy-metal ion adsorption
The effect of temperature on ion sorption from 273 to 348 K is shown in figure . An increase in temperature beyond 298 K adversely affected the sorption behavior of the polymeric supports. The results are of technical interest because at lower temperatures these hydrogels exhibit higher efficiency. The same phenomenon was also observed by another group [Citation15]. At higher temperatures, metal ions have higher velocity and activation energy. Thus, it is easier for ions to escape from the restrictions due to electrostatic affinity and chelation, which results in decreases in the amounts of Ni2 +, Cr3 + and Cd2 + adsorbed.
Adsorption mechanism of heavy-metal ions in hydrogels
The Langmuir theory is introduced to study Ni2 + adsorption rate. The correlative equation and its conversion form are presented in equations (Equation33 ) and (Equation4
4 ), respectively
3
4 where v is the amount of adsorbed ion, vs is the saturated sorption value, ve is the equilibrium sorption value and k1 and k2 are the adsorption and desorption rates, respectively; ϕ is the net adsorption rate defined as k1+k2.
PAC/PEG IPN hydrogel has a regular porous structure (shown in figure ). The special structure gives the hydrogels good adsorption properties. In our experiment, equation (Equation44 ) becomes
5 Here, mt and me are the Ni2 + sorption values at time t and ∞, respectively. On the basis of equation (Equation5
5 ), the function ln (1-mt/me)−t at different neutralization degrees is shown in figure . This function shows a strong linear relation with time, and ϕ increases with increasing neutralization degree. This is because the number of –COO- groups increases with increasing neutralization degree. The –COO
- groups have good coordination ability and electrostatic affinity to heavy-metal ions, thus, the adsorption rate increases with increasing neutralization degree.
Figure 7 Values of ln (l-mt/me)−t for PAC/PEG IPN hydrogel with different neutralization degrees for AA. The neutralization degrees and ϕ values are (▪) 20%, 0.0235; (•) 40%, 0.0299; (▴) 60%, 0.0323; (▾) 80%, 0.0343 and (◂) 100%, 0.0365, respectively.
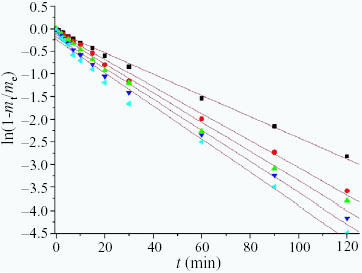
To determine the diffusion mechanism of heavy-metal ions in the hydrogels, the Fickian diffusion theory is introduced [Citation18]:
6 where Mt and M∞ are the masses of diffusion at time t and ∞, respectively. The relative diffusion rate of heavy-metal ions is proportional to the square root of time. Peppas extended the Higuchi model to a more generalized form:
7 where nis the diffusion exponent. Information on the diffusion mechanism can be gained by fitting the adsorption data and comparing the values of nwith the semi-empirical values for the various geometries reported by Peppas and Ritger [Citation19, Citation20]. For a cylindrical geometry, n 0.45 corresponds to a purely Fickian diffusion mechanism, n> 0.89 indicates a relaxation-controlled mechanism, and 0.45 <n< 0.89 indicates an anomalous diffusion mechanism. Despite the approximate nature of these values, it has been found that these relationships can be applied to adsorption data, indicating that the diffusion-based models of Higuchi and Peppas describe the diffusion of ions from a polymeric system quite well. On the basis of equation (Equation7
7 ) and figure , the relationship of ln(Mt/M∞) versus ln t is plotted in figure .
Figure 8 Values of lnMt/M∞−ln t for PAC/PEG IPN hydrogel with different neutralization degrees. The slopes of the two lines under different neutralization degrees are (▪) 20%, 0.8503 and 0.2858; (•) 40%, 0.7897 and 0.1567; (▴) 60%, 0.6899 and 0.1549; (▾) 80%, 0.6596 and 0.1469; (◂) 100%, 0.6444 and 0.0951.

It is clear that there are two different slopes for each curve. The diffusion exponent n decreases with increasing neutralization degree in both stages. In 0.03 M Ni2 + solution, the n for hydrogels with different neutralization degrees are 0.8503 and 0.2858 at 20%, 0.7897 and 0.1567 at 40%, 0.6899 and 0.1549 at 60%, 0.6596 and 0.1469 at 80% and 0.6444 and 0.0951 at 100%, respectively. From figure , in the first stage, 0.45 <n< 0.89, which indicates an anomalous diffusion mechanism for Ni2 + adsorption. In the second stage, all n values are lower than 0.45, which indicates Fickian diffusion. The first stage is associated with heavy-metal ion diffusion in the porous hydrogel, and the second stage is attributed to the synergy effect between electrostatic force and chelation.
Comparison of adsorption behavior
In the present work we focus on comparing the adsorptions of a swollen PAC/PEG IPN hydrogel and a dried PAC/PEG composite. The PAC/PEG species in the forms of both swollen hydrogel and dried composite can adsorb metal ions. The amount of adsorbed metal ions during swelling and deswelling in the NiCl2, CrCl3 and Cd(NO3 )2 solutions used for Ni2 +, Cr3 + and Cd2 + removal, respectively, are presented in figure . It can be seen that the amount of adsorbed metal ions during deswelling is several times that during swelling, and the time to reach adsorption equilibrium is much shorter. According to the general process described in [Citation21]. First, water molecules enter the hydrogel; in this period, the swollen ratio (SR) [Citation13] of the hydrogel is proportional to the square root of absorption time (about 0.5 h) [Citation22]; second, macromolecule chains relax, during which SR is proportional to the absorption time (about 3 h) [Citation23]; third, the whole of each macromolecule chain stretches in water. In heavy-metal ion solutions, the adsorption to Ni2 +, Cr3 + and Cd2 + by the hydrogel occurred in the second and third steps, which explains why the metal ion adsorption time during deswelling is shorter than that during swelling.
Figure 9 Adsorption rates of Ni2 +, Cr3 + and Cd2 + by swollen hydrogel (•) and dry composite (▪) in 0.03 M solution at room temperature and neutralization degree of 80%.
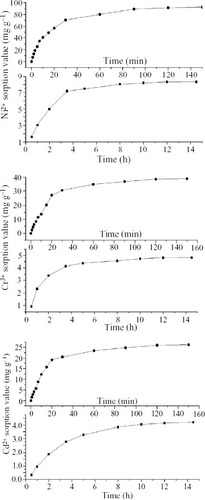
The PAC/PEG IPN hydrogel is expected to remove many other heavy-metal ions. Figure shows that the amounts of Ni2 +, Cr3 + and Cd2 + adsorbed by the swollen hydrogel are much greater than those by the dried composite under the same conditions. Moreover, the swelling kinetics of the dried PAC/PEG composite in salt solution is as follows [Citation24]. Step (A): water diffuses into the composite network owing to capillary forces as soon as it comes in contact with the PAC/PEG composite, then the PAC begins to ionize in water, and K + is released and moves freely in the swelling hydrogel network. Step (B): an osmotic pressure between the salt solution and the composite network is produced, which further impels the penetration of water into the network and ionizes the composite, while the composite is transformed into a hydrogel. According to the principle of electrical neutrality, K + in the hydrogel can only diffuse to the interface of the hydrogel and absorb the anions of the swelling medium there because of electrostatic affinity. Thus, a double-electrode layer is formed on the surface of the hydrogel, which prevents the heavy-metal ions in the solution from diffusing into and chelating with the hydrogel network. Step (C): the osmotic pressure of the double-electrode layer begins to decrease when composite swelling ratio increases to a certain value, and absorption rate decreases correspondingly. On the other hand, once the chelation occurs after step (A), the effective crosslink density at the surface increases markedly [Citation25]; thus according to the Flory theory [Citation26], the swelling rate decreases. Furthermore, the compact hydrogel surface prevented the diffusion and chelation of heavy-metal ions to the –COOH and –COO- groups inside the hydrogel; thus toxic-ion removal capability is reduced.
The network is fully stretched for the swollen hydrogel in distilled water. A negative osmotic pressure between the solution and the composite network is produced, which is advantageous for the penetration of heavy-metal ions into the hydrogel network. With the enhancement of chelation, the negative osmotic pressure reduces gradually until adsorption equilibrium is obtained. According to above discussion and our experiment results, however large the hydrogel network becomes, it ultimately becomes a metal complex. Thus, it is preferable to remove heavy-metal ions using a swollen hydrogel rather than a dried one.
Thermal analysis
Figure shows a typical TGA graph of weight loss as a function of temperature for the PAC/PEG IPN composite and its resultant complexes of PAC/PEG–Cd, PAC/PEG–Cr and PAC/PEG–Ni. The TGA data of the samples show no weight loss up to 200 °C, after which a sharp decrease is observed at approximately 350 °C. This sharp weight loss is observed up to 400 °C and translates into overall weight losses of 3.8, 8.5, 11.4, and 13.6% for PAC/PEG IPN, PAC/PEG–Cd, PAC/PEG–Cr and PAC/PEG–Ni, respectively. From the TGA curves, it can be seen that the thermal stability of the PAC/PEG IPN composite increases after the chelation with heavy-metal ions. The species that remain undecomposed above 500 °C are the corresponding metal and oxide. It can be seen that the order of the thermal stabilities of the three complexes is PAC/PEG–Ni>PAC/PEG–Cr>PAC/PEG–Cd, indicating that the amounts of heavy-metal ion adsorbed are in the order of Ni2 +> Cr3 +> Cd2 +, which is consistent with the experimental results.
Conclusions
A PAC/PEG IPN hydrogel was synthesized by a simple two-step aqueous polymerization method. We investigated the effects of the synthesis parameters, temperature and ion concentration on Ni2 +, Cr3 + and Cd2 + adsorption onto IPN hydrogels. At higher neutralization degrees, higher AA dosages and lower temperatures, the hydrogel exhibited higher adsorption capacity. We compared the adsorption of the hydrogel during swelling and deswelling. The experimental results revealed that the amount of heavy-metal ion adsorbed during deswelling was higher than that during swelling. The diffusion and adsorption mechanisms were investigated using the Fickian diffusion and Langmuir theories.
Acknowledgments
The authors acknowledge the joint support of the National High Technology Research and Development Program of China (863 Program) (no. SQ2008AA03Z2470974), the National Natural Science Foundation of China (no. 50572030, 50842027) and the Key Scientific Technology Program of Fujian, China (no. 2005HZ01-4 and no. 2007HZ0001-3).
References
- LeeW FChenY C 2005 Eur. Polym. J. 41 1605 http://dx.doi.org/10.1016/j.eurpolymj.2005.02.011
- EssawyH AIbrahimH S 2004 React. Funct. Polym. 61 421 http://dx.doi.org/10.1016/j.reactfunctpolym.2004.08.003
- Abd El-RehimH AHegazyE AEl-Hag AliA 2000 React. Funct. Polym. 34 105 http://dx.doi.org/10.1016/S1381-5148(99)00009-7
- El-Hag AliAShawkyH AAbd El RehimH AHegazyE A 2003 Eur. Polym. J. 39 2337 http://dx.doi.org/10.1016/S0014-3057(03)00150-2
- ChauhanG SLalS 2003 Desalination 159 131 http://dx.doi.org/10.1016/S0011-9164(03)90065-8
- ChauhanG SMahajanS 2002 J. Appl. Polym. Sci. 76 667 http://dx.doi.org/10.1002/app.10943
- NastasoviAJovanoviSDordevecDOnjiaAJakovljeviDNovakovicT 2004 React. Funct. Polym. 58 139 http://dx.doi.org/10.1016/j.reactfunctpolym.2003.11.015
- WuJ HLinJ MZhouM 2000 Macromol. Rapid Commun. 21 1032 http://dx.doi.org/10.1002/1521-3927(20001001)21:15<1032::AID-MARC1032>3.0.CO;2-N
- LinJ MWuJ HYangZ FPuM L 2001 Macromol. Rapid Commun. 22 422 http://dx.doi.org/10.1002/1521-3927(20010301)22:6<422::AID-MARC422>3.0.CO;2-R
- NazmutdinovaGSensfussSSchrödnerMHinschASastrawanRGerhardDHimmlerSWasserscheidP 2006 Solid State Ion. 177 3141 http://dx.doi.org/10.1016/j.ssi.2006.08.020
- SaravananPRajuM PAlamS 2007 Mater. Chem. Phys. 103 278 http://dx.doi.org/10.1016/j.matchemphys.2007.02.025
- WuGSuBZhangW GWangC T 2008 Mater. Chem. Phys. 107 364 http://dx.doi.org/10.1016/j.matchemphys.2007.07.028
- TangQ WLinJ MWuJ HZhangC JHaoS C 2007 Carbohyd. Polym. 67 332 http://dx.doi.org/10.1016/j.carbpol.2006.05.026
- HouXSiowK S 2001 Polymer 42 4181 http://dx.doi.org/10.1016/S0032-3861(00)00818-1
- ChauhanG SJaswalS CVermaM 2006 Carbohyd. Polym. 66 435 http://dx.doi.org/10.1016/j.carbpol.2006.03.030
- ZhangX ZWuD QChuC C 2004 Biomaterials 25 4719 http://dx.doi.org/10.1016/j.biomaterials.2003.11.040
- GilesG HSmithDHuitsonA 1974 J. Colloid Interface Sci. 47 755 http://dx.doi.org/10.1016/0021-9797(74)90252-5
- CrankG 1975 The Mathematics of Diffusion Oxford Clarendon
- PeppasNRitgerP L 1987 J. Control. Release 5 23 http://dx.doi.org/10.1016/0168-3659(87)90034-4
- PeppasNRitgerP L 1987 J. Control. Release 5 37 http://dx.doi.org/10.1016/0168-3659(87)90035-6
- EnscoreD JHopfrabergH BStannettV T 1977 Polymer 18 793 http://dx.doi.org/10.1016/0032-3861(77)90183-5
- ZhangX ZZhouR X 2000 J. Colloid Interface Sci. 223 311 http://dx.doi.org/10.1006/jcis.1999.6654
- ZhangX ZZhouR XYangY 2002 Biomaterials 23 1313 http://dx.doi.org/10.1016/S0142-9612(01)00249-6
- ChenZ BLiuM ZQiX HZhanF LLiuZ 2007 Electrochim. Acta 52 1839 http://dx.doi.org/10.1016/j.electacta.2006.07.044
- WangJ QWuW H 2005 Eur. Polym. J. 41 1143 http://dx.doi.org/10.1016/j.eurpolymj.2004.11.034
- FloryP J 1953 Principles of Polymer Chemistry New York Cornel University Press