Abstract
Fe3Al intermetallics (Fe3Al, Fe3Al-Zr, Fe3Al-Zr,Mo and Fe3Al-Zr, Mo, Nb) were oxidized at 950 °C in dry and humid (11 vol% water) synthetic air. Thermogravimetric measurements showed that the oxidation rates of the tested intermetallics were lower in humid air than in dry air (especially for Fe3Al-Zr, Mo and Fe3Al-Zr, Mo, Nb). The addition of small amounts of Zr, Mo or Nb improved the kinetics compared with that of the undoped Fe3Al. Fe3Al showed massive spallation, whereas Fe3Al-Zr, Fe3Al-Zr, Mo and Fe3Al-Zr, Mo, Nb produced a flat, adherent oxide layer. The rapid transformation of transient alumina into alpha alumina may explain the decrease in the oxidation rate in humid air.
Introduction
Intermetallic compounds [Citation1–4], particularly iron aluminides [Citation5–10], are resistant to oxidation, owing to the presence of a large Al ‘reservoir’ that enables the formation and maintenance of a protective alumina layer [Citation11–15].
The addition of small amounts of alloy can significantly improve the mechanical and corrosion resistance properties. This is particularly true for reactive-element addition, whose effect on the high-temperature corrosion of alumina-forming materials was largely studied [Citation16–27].
In many applications, humid air is corrosive [Citation28]. The effects of water vapor on the oxidation behavior and mechanical properties of intermetallics have not been extensively studied [Citation29–32]. Zeller et al [Citation29] found that water vapor causes a significant increase in the oxidation kinetics accompanied by a change in the microstructure and morphology of the oxide layer in TiAl alloys. Pint et al [Citation31] observed an increase in the level of spallation in NiAl intermetallics with increasing water vapor content, particularly if they do not contain small amounts of elements such as Y or Hf. Pérez et al [Citation30] studied the effect of iron aluminide coatings on ferritic steels. The presence of the coating delayed the degradation of the steel by preventing the formation of Cr-containing volatile species. Similar results were obtained by Agüero et al for iron aluminide-coated ferritic steels [Citation32] after a very prolonged exposure (32 000 h) to steam at 650 °C. According to a recent review by Saunders et al [Citation33], water vapor strongly affects the high-temperature oxidation behavior of alumina-forming materials. Except for the paper of Regina et al [Citation34], which deals with the effect of water on the oxidation of Fe(14–19)Al(2–5)Cr alloys in O2/CO2/N2 atmosphere, we are not aware of any other study that deals with the effect of water vapor on iron aluminide intermetallics.
The goal of this study is to examine the effect of water vapor on the high-temperature oxidation behaviors of Fe3Al intermetallic compounds. To test the effect of humidity in the oxidizing atmosphere, a set of experiments was performed at 950 °C in synthetic air with a controlled water vapor pressure. The effect of the selected addition of Zr, Mo, Nb and Cr on the high-temperature corrosion (degradation) behavior of tested intermetallics in dry or humid air under isothermal conditions at 950 °C was examined.
Experimental procedure
The base materials used for the experiments were iron aluminide with the addition of supplementary elements (Cr, Zr, Mo, Nb, B and C) provided by the Military University of Technology in Warsaw (Poland). The chemical compositions of the Fe3Al-modified elements are listed in table . The alloys were melted in a Balzers induction furnace in argon atmosphere and cast into graphite moulds (with a diameter of 25 mm and length of 500 mm). The samples were homogenized at 1100 °C for 5 h, followed by hot forging at 1100 °C and hot rolling at 650–750 °C, resulting in tapes 12 mm wide and 1 mm thick. The specimens consisted of grains with an average size of 200–250 μm. Specimens cut from the ingots were approximately 10×10×1 mm3 in size. They were manually ground using SiC abrasive paper to 1200 grade. The samples were ultrasonically cleaned in ethanol, dried at room temperature, and weighed.
Table 1 Chemical composition of Fe3Al alloys (at.%) (Fe balance).
For the water vapor experiments, synthetic air was enriched with 11 vol% H2O; the experiments were conducted with a thermobalance (Setaram TGA 92) that was adapted for H2O vapor atmosphere (figure ). The synthetic air (carrier gas) was passed through boiling distilled water of a flow rate of approximately 50 ml min−1. The H2O fraction was controlled by bubbling synthetic air through a cooling tube maintained at a certain temperature (36 °C for 11 vol%), so that the H2O partial pressure could be adjusted. The humidity content of 11 vol% was used for most ‘humid’ oxidation experiments, and this medium is called humid air thereafter. Figure shows the relationship between the temperature of the cooling column and the water vapor content of air. The samples were oxidized for 24 h at 950 °C and continuously weighed. Their oxidation behavior was compared with that of the same samples oxidized in dry air.
Figure 1 Equipment for the thermogravimetric measurements in humid atmosphere: (1) water bath thermostat, (2) gas inlet, (3) flow meter, (4) water bath/humidifier, (5) heating wire, (6) furnace, (7) thermobalance and (8) gas outlet.
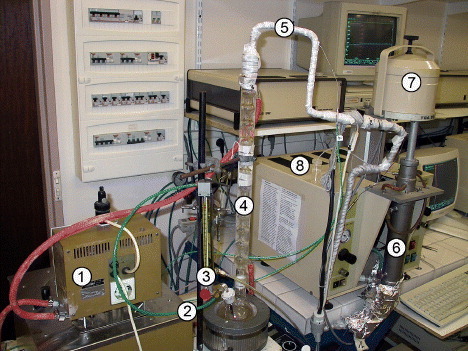
The morphology, structure and chemistry of the oxidized specimens were investigated using a JEOL JSM-6400F scanning electron microscope (SEM, spatial resolution: 1.5 nm) equipped with a field emission gun and an energy dispersive x-ray analyzer (EDX). The phase compositions of the produced surface oxides were identified by x-ray diffraction (XRD) using CuKα (λ=0.154056 nm) radiation.
Results and discussion
Comparison of kinetics in dry and humid air
Figure shows the specific mass gain as a function of oxidation time at 950 °C in humid air for Fe3Al, Fe3Al-Zr, Fe3Al-Zr,Mo and Fe3Al-Zr,Mo,Nb. The oxidation data of Fe3Al, Fe3Al-Zr, Fe3Al-Zr,Mo and Fe3Al-Zr,Mo,Nb were fitted by parabolic law except for the first 5 h of oxidation. The oxidation behavior is classified into two groups. Undoped Fe3Al exhibits a higher weight gain and a kp value increase of about one order of magnitude compared with the kp values determined for the second group composed of Fe3Al-Zr, Fe3Al-Zr,Mo and Fe3Al-Zr,Mo,Nb.
Figure 3 Comparison of isothermal oxidation kinetic curves for Fe3Al, Fe3Al-Zr, Fe3Al-Zr,Mo and Fe3Al-Zr,Mo,Nb alloys at 950 °C in dry air and humid air (11 vol% H2O).
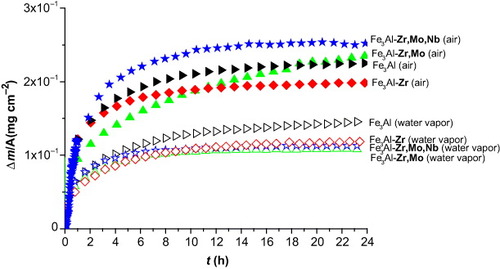
Fe3Al-Zr appears slightly less efficient than Fe3Al-Zr,Mo and Fe3Al-Zr,Mo,Nb, as its weight gain and kp value are slightly higher than those of the two other intermetallics. Table summarizes the parabolic rate constants kp at 950 °C for each Fe3Al alloy.
Table 2 Parabolic rate constants obtained for the Fe3Al alloys oxidized for 24 h at 950 °C in humid or dry synthetic air under isothermal condition.
Figure shows a superimposition of the kinetics obtained in dry or humid air. The kinetics clearly shows that, whatever the intermetallic, the weight gains strongly decrease when the oxidizing atmosphere is enriched with water vapor. This effect is also visible in the case of the parabolic rate constants (table ), particularly for Fe3Al-Zr,Mo and Fe3Al-Zr,Mo,Nb that exhibit kp values one order of magnitude higher in dry air than in humid air.
Because the Mo-doped alloy exhibited a higher oxidation mass gain in dry air, but this gain became the lowest in humid air, it appears that Mo plays an important role in the oxidation behavior. As Mo is known to form hydroxide in humid atmosphere, the specific behavior of Mo-containing samples in humid air could be explained by the sufficiently high vapor pressure of molybdenum hydroxide that could cause mass loss during the oxidation.
The effect of water vapor is prominent during the first step of the oxidation. A steep weight gain curve is observed for the samples oxidized in dry air; it rapidly evolves toward a parabolic shape for humid air, whereas it transforms later when the oxidation is performed in dry air. The change in the slope of the weight gain curve is generally ascribed to the formation of transient alumina, which transforms into continuous and stable α-Al2O3 [Citation35].
Surface morphologies and characteristics after oxidation in humid air
Figure show the surface morphologies of the oxide layers formed on Fe3Al, Fe3Al-Zr, Fe3Al-Zr,Mo and Fe3Al-Zr,Mo,Nb alloys at different magnifications after isothermal oxidation in humid air for 24 h at 950 °C.
Figure 4 SEM images of the layer formed on Fe3Al (a and b), Fe3Al-Zr (c and d), Fe3Al-Zr,Mo (e and f) and Fe3Al-Zr,Mo,Nb (g and h) alloys after 24 h at 950 °C in humid air.
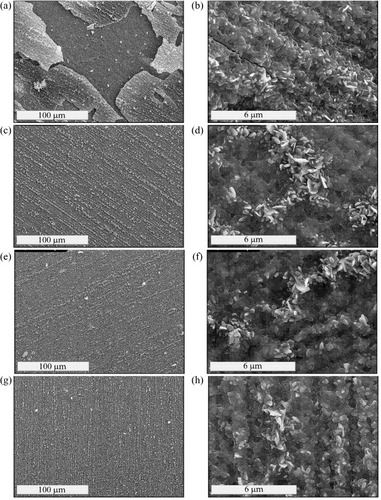
The oxide layer formed on the Fe3Al alloy shows massive spallation in large sheets (figure (a)). The underlying metallic substrate is covered with α-Al2O3 nuclei and oxide imprints. The layer is composed of platelets, pores and cellular depressions (figure (b)). Moreover, grinding marks and cracks are visible.
A different morphology is observed in Fe3Al-Zr. The layer is flat, uniform and spallation-free (figure (c)). An enhanced formation of fine oxide nodules aligned with polishing marks and accompanied by crater formation is observed at the layer surface. At the boundary regions of the oxide crater nodule, there is a radial arrangement of the platelets, but they increasingly appear as bumps towards the central region (figure (d)). Some pores are visible all over the surface.
The Fe3Al-Zr,Mo produced a similar microstructure, except that the radial arrangements appeared to be smaller and less pronounced (figures (e) and (f)).
The oxide layer formed on Fe3Al-Zr,Mo,Nb appears to be similar to those of the above-mentioned Fe3Al-Zr and Fe3Al-Zr,Mo (figures (g) and (h)). Sparse spallation was noted.
No traces of transient alumina could be detected in our SEM observations. The presence of pores (ridge and cellular shape) in the Al2O3 layer is often described in the literature as the result of the rapid transformation of transient to alpha alumina owing to the huge volume contraction (around 14% [Citation36]). This is confirmed by the absence of any evidence of transient alumina (needle or platelet) and by the steep kinetics that is usually proposed to result from a very rapid transformation of the initial transient alumina to α-Al2O3.
Analyses of the XRD patterns of all the specimens after oxidation at 950 °C in humid air revealed only α-Al2O3, the metallic substrate (figure ). In air, a previous study clearly showed transient alumina morphology on the top of the alumina layers formed on Fe3Al and Fe3Al-Zr [Citation37].
Figure 5 X-ray diffraction patterns for Fe3Al, Fe3Al-Zr, Fe3Al-Zr,Mo and Fe3Al-Zr,Mo,Nb alloys oxidized for 24 h at 950 °C in humid air.
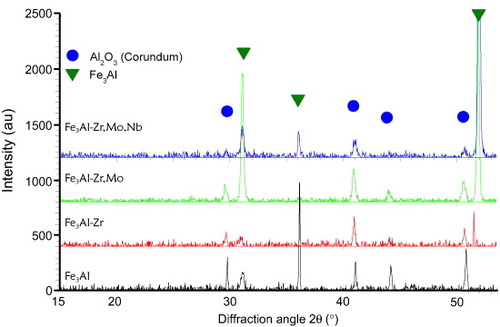
The transformation from transient alumina to α-Al2O3 appears much faster in humid air than in dry air. Similar observations were made by Buscail et al [Citation38, Citation39] who noted a lower mass gain under humid conditions and a continuous layer with a structure close to that of the protective α-Al2O3. Götlind et al [Citation40] reported that the presence of 40% water vapor in O2 increased the oxidation rate of FeCrAl steel during the first 72 h at 900 °C.
These preliminary results reveal the role played by water vapor. Data collected from the literature show that steam, or water in the gaseous state, can have various effects on the oxidation of alloys. These effects depend on the alloy under consideration, oxidation temperature, water vapor pressure and gas flow rate. Water vapor was found to affect the high-temperature oxidation of alloys in the following ways through the following mechanisms:
The presence of water vapor results in the formation of H2–H2O in voids that facilitate a rapid inward transport of oxygen across the pores via dissociative gas transport (an oxidation–reduction process) [Citation41, Citation42].
Water vapor can affect the plasticity of oxide layers. Some investigators proposed that the plasticity of oxide layers decreases and, consequently, the spallation of layers increases [Citation43]. Other investigators have proposed that plasticity of oxide layers increases with the increased oxidation rates or improved adherence [Citation41, Citation44].
The presence of water vapor adversely affects the selective oxidation of elements such as aluminum and chromium from iron-based alloys [Citation45]. Water vapor also affects the selective oxidation of aluminum from TiAl [Citation46].
Water vapor can affect diffusion of species through oxides such as SiO2 [Citation41] by changing the network structure [Citation47].
Water vapor can increase the level of the vaporization of some oxides because of the formation of hydrated species at high vapor pressures [Citation48, Citation49].
Water vapor increases the concentration of proton defects in oxide layers that can affect defect-dependent properties such as high-temperature creep and diffusion [Citation50, Citation51].
The still unanswered question is how and why the presence of water vapor can increase this transformation. Further investigations, such as, transmission electron microscopy observations and marker experiments on cross sections with concentration profiles of elements after prolonged oxidation, should be conducted to explain the growth mechanism of the alumina layer in humid air. Recent marker experiments using H216O/H218O [Citation53] and 16O2/18O2 [Citation54] yielded interesting results on the growth mechanism of oxide layers in humid or dry air. Ongoing investigations on H216O/H218O/D2O, the location of the oxygen isotopes, as well as that of deuterium in the alumina layers, should improve our understanding of the high-temperature oxidation in water vapor, which requires information on the role of OH and proton species in the growth of the alumina layer. Their location, using isotopes, could explain their effect on the transport properties in the thermally growing alumina layer. The transformation of transient phases to stable alpha alumina could then be directly related to the transport mechanism through the layer during its establishment in water vapor.
Conclusions
The weight gains and parabolic rate constants of the tested intermetallics were lower in air mixed with 11% H2O than in dry air. The effect is dependent on the intermetallics, as the oxide layer growth rates for Fe3Al and Fe3Al-Zr did not appear to be strongly affected by the water vapor atmosphere in comparison with oxidation in air at the same temperature.
The presence of pores (ridge and cellular shape) in the Al2O3 layer, absence of transient alumina (needle or platelet) and steep kinetics at the start of the oxidation suggest a very rapid transformation of the initial transient alumina to α-Al2O3.
Acknowledgment
The authors would like to thank Professor G Moulin (UT Compiègne) for his fruitful collaboration in the calibration of the H2O-producing device.
References
- WestbrookJ H 1959 Mechanical Properties of Intermetallic Compounds New York Wiley
- WestbrookJ H 1960 Intermetallic Compounds New York Wiley
- StoloffN SDavisR G 1966 Prog. Mater. Sci. 13 1 http://dx.doi.org/10.1016/0079-6425(68)90018-2
- BakerIMunroeP R 1990 High Temperature Aluminides and Intermetallics Warrendale, PA Materials Research Society
- BoggsW E 1971 J. Electrochem. Soc. 118 906 http://dx.doi.org/10.1149/1.2408222
- TomaszewiczPWallworkG R 1978 Rev. High Temp. Mater. 4 75
- SmialekJ LDoychakJGaydoshD J 1990 Oxid. Met. 34 259 http://dx.doi.org/10.1007/BF00665018
- RommerskirchenIEltesterBGrabkeH J 1996 Mater. Corros. 47 646 http://dx.doi.org/10.1002/maco.19960471109
- XuC HGaoWGongH 2000 Intermetallics 8 769 http://dx.doi.org/10.1016/S0966-9795(00)00007-8
- XuC HGaoWLiD 2001 Corros. Sci. 43 671 http://dx.doi.org/10.1016/S0010-938X(00)00104-9
- KofstadP 1988 High-Temperature Corrosion New York Elsevier
- WrightI GPintB ATortorelliP F 2001 Oxid. Met. 55 333 http://dx.doi.org/10.1023/A:1010316428752
- PintB ATortorelliP FWrightI G 1996 Mater. Corros. 47 663 http://dx.doi.org/10.1002/maco.19960471202
- PintB ATortorelliP FWrightI G 1999 Mater. High Temp. 16 1 http://dx.doi.org/10.3184/096034099783641236
- PintB AMoreK LTortorelliP FPorterW DWrightI G 2001 Mater. Sci. Forum 369–372 411 http://dx.doi.org/10.4028/www.scientific.net/MSF.369-372.411
- JedlinskiJ 1997 Solid State Ion. 101–103 1033 http://dx.doi.org/10.1016/S0167-2738(97)00299-3
- CueffRBuscailHCaudronEIssartelCRiffardF 2002 Oxid. Met. 58 439 http://dx.doi.org/10.1023/A:1020511304401
- CueffRBuscailHCaudronEIssartelCRiffardF 2003 Appl. Surf. Sci. 207 246 http://dx.doi.org/10.1016/S0169-4332(02)01506-4
- TienJ KPettitF S 1972 Met. Trans. 3 1587 http://dx.doi.org/10.1007/BF02643050
- PrescottRGrahamM J 1992 Oxid. Met. 38 233 http://dx.doi.org/10.1007/BF00666913
- JedlinskiJ 1993 Oxid. Met. 39 55 http://dx.doi.org/10.1007/BF00666609
- KimK Y 1994 Oxid. Met. 41 179 http://dx.doi.org/10.1007/BF01080780
- TaniguchiS 1997 Mater. Corros. 49 1
- ChevalierSStrehlGBuscailHBorchardtGLarpinJ P 2004 Mater. Corros. 55 352 http://dx.doi.org/10.1002/maco.200303748
- ChevalierSTankeuA P DBuscailHIssartelCBorchardtGLarpinJ P 2004 Mater. Corros. 55 610 http://dx.doi.org/10.1002/maco.200303773
- ChevalierSStrehlGBuscailHIssartelCBorchardtGLarpinJ P 2006 Mater. Corros. 57 476 http://dx.doi.org/10.1002/maco.200503943
- HoungninouCChevalierSLarpinJ P 2006 Oxid. Met. 65 409 http://dx.doi.org/10.1007/s11085-006-9033-y
- DouglassD LKofstadPRahmelAWoodG C 1996 Oxid. Met. 45 529 http://dx.doi.org/10.1007/BF01046850
- ZellerADettenwangerFSchützeM 2002 Intermetallics 10 59 http://dx.doi.org/10.1016/S0966-9795(01)00104-2
- PérezF JCastanedaS I 2007 Surf. Coat. Technol. 201 6239 http://dx.doi.org/10.1016/j.surfcoat.2006.11.029
- PintB AHaynesJ AZhangYMoreK LWrightI G 2006 Surf. Coat. Technol. 201 3852 http://dx.doi.org/10.1016/j.surfcoat.2006.07.244
- AgüeroAMuelasRPastorAOsgerbyS 2005 Surf. Coat. Technol. 200 1219 http://dx.doi.org/10.1016/j.surfcoat.2005.07.080
- SaundersS R JMonteiroMRizzoF 2008 Prog. Mater. Sci. 53 775 http://dx.doi.org/10.1016/j.pmatsci.2007.11.001
- ReginaJ RDuPontJ NMarderA R 2004 Oxid. Met. 61 69 http://dx.doi.org/10.1023/B:OXID.0000016277.86061.25
- BerthomeEN'DahE GWoutersYGalerieA 2005 Mater. Corros. 56 389 http://dx.doi.org/10.1002/maco.200403851
- PrescottRGrahamM 1992 Oxid. Met. 38 675
- JuzonPChevalierSGalerieABorchardtGPrzybylskiKLarpinJ P Oxid. Met. to be published
- BuscailHHeinzeSDufourP 1997 J. Chim. Phys. 94 553
- BuscailHHeinzeSDufourPLarpinJ P 1997 Oxid. Met. 47 445 http://dx.doi.org/10.1007/BF02134786
- GötlindHLiuFSvenssonJ EHalvarssonMJohanssonL G 2007 Oxid. Met. 67 252 http://dx.doi.org/10.1007/s11085-007-9055-0
- RahmelATobolskiJ 1965 Corros. Sci. 5 33
- TuckC WOdgersMSachsK 1969 Corros. Sci. 9 271 http://dx.doi.org/10.1016/S0010-938X(69)80056-9
- McCarronR LShulJ W 1973 The effects of water vapor on the oxidation behavior of some heat-resistant alloys Proc. Symp. High Temperature Gas–Metal Reactions in Mixed Environment New York AIME p 360
- BouaouineHArmanetFCoddetC 1989 International Congress on Metallic Corrosion (Toronto, Canada) p 379
- KvernesLOliveiraMKofstadP 1997 Corros. Sci. 17 237 http://dx.doi.org/10.1016/0010-938X(77)90049-X
- KremerRAuerW 1997 Mater. Corros. 48 35 http://dx.doi.org/10.1002/maco.19970480107
- IreneE A 1974 J. Electrochem. Soc. 121 1613 http://dx.doi.org/10.1149/1.2401753
- OpikaE JHannR E 1997 J. Am. Ceram. Soc. 80 197 http://dx.doi.org/10.1111/j.1151-2916.1997.tb02810.x
- SmialekJ LRobinsonR COpikaE JFoxD SJacobsonN S 1999 Adv. Compos. Mater. 8 33 http://dx.doi.org/10.1163/156855199X00056
- SedriksA J 1996 Corrosion of Stainless Steels 2nd edn New York Wiley
- KofstadP 1991 Microscopy of Oxidation M JBennett G WLorimer London The Institute of Metals p 2
- ChevalierSMolinsRHeintzOLarpinJ P 2005 Proc. 6th Int. Conf. on Microscopy of Oxidation (Birmingham, England) G JTatlock H EEvans p 365
- BambaGWoutersYGalerieABorchardtGShimadaSHeintzOChevalierS 2007 Scr. Mater. 57 671 http://dx.doi.org/10.1016/j.scriptamat.2007.06.050
- ChevalierS 2007 Traitements de surface et nouveaux matériaux: Quelles solutions pour lutter contre la dégradation des matériaux à haute température? Dijon, France Les Editions Universitaires de Dijon