Abstract
Regenerative medicine is an exciting field that aims to create regenerative alternatives to harvest tissues for transplantation. In this approach, the delivery of cells and biological molecules plays a central role. The scaffold (synthetic temporary extracellular matrix) delivers cells to the regenerative site and provides three-dimensional environments for the cells. To fulfil these functions, we design biodegradable polymer scaffolds with structural features on multiple size scales. To enhance positive cell–material interactions, we design nano-sized structural features in the scaffolds to mimic the natural extracellular matrix. We also integrate micro-sized pore networks to facilitate mass transport and neo tissue regeneration. We also design novel polymer devices and self-assembled nanospheres for biomolecule delivery to recapitulate key events in developmental and wound healing processes. Herein, we present recent work in biomedical polymer synthesis, novel processing techniques, surface engineering and biologic delivery. Examples of enhanced cellular/tissue function and regenerative outcomes of these approaches are discussed to demonstrate the excitement of the biomimetic scaffold design and biologic delivery in regenerative medicine.
Introduction to polymers in regenerative medicine
Biocompatible polymers have played an integral role in tissue engineering, especially within the realm of scaffold design [Citation1, Citation2]. These materials are chosen based on their acceptance in the body environment and their ability to biodegrade at a rate comparable to the rate of tissue regeneration. Of the multiple polymeric materials used for tissue engineering, some are naturally derived, including collagen, gelatin and chitosan, and many more are synthetic, including polyethylene oxide (PEO), polyvinyl alcohol (PVA), poly(lactic acid) (PLA) and poly(glycolic acid) (PGA), and copolymers of the two (PLGA).
Naturally derived polymers are limited in supply and can therefore be costly, while synthetic polymers are widely available and have been broadly used in the design of scaffolds for various tissue engineering applications. PLA, PGA, and their copolymer are the most commonly used synthetic polymers that degrade through hydrolysis into non-toxic products. Poly(L-lactic acid) (PLLA) in particular is a synthetic polymer which can be readily manipulated into thin films, micro- and nano-fibers, porous scaffolds, three-dimensional fibrous networks and other complex geometries, making it a good candidate as a material for scaffold fabrication.
Our group has developed multiple techniques for forming highly porous biodegradable polymer and polymer/apatite composite scaffolds with various geometries conducive to cell differentiation and bone tissue regeneration. This review showcases some of these results, in which polymer scaffolds are designed to positively affect cell differentiation and ultimately lead to new tissue formation. This is done by mimicking the structural components of the extracellular matrix, as well as through using the scaffold as a vehicle for the delivery of biologic agents to aid in bone formation.
The scaffold as a temporary extracellular matrix
The extracellular matrix (ECM) is a model used for inspiration when designing biomimetic scaffolds for tissue engineering [Citation3]. By mimicking the structure and/or surface properties of the naturally occurring ECM, engineers may be able to influence cell growth and tissue formation. The features shared by the ECM and these scaffolds act to promote cell attachment and proliferation and provide biological cues to the cells.
The collagen fibers present in the bone ECM are between 50 and 500 nm in diameter. These fibers act as a foundation, onto which osteoblasts deposit apatite crystals during bone formation. It is widely hypothesized that this fibrous construct may play an important role in regulating cell behavior and subsequent tissue growth. Much work in biomimetic scaffold design has focused on maintaining and improving upon these features by more effectively mimicking the natural ECM [Citation4].
Another way in which this is being done is by creating biomimetic composite scaffolds, which incorporate a ceramic component into the polymer network. For example, by combining a polymer with hydroxyapatite (HA), we can study the mineralization of the ECM collagen creating a scaffold that mimics those mineralized collagen fibers.
Nano-scale engineering and scaffold design
The fabrication of nano-fibers that mimic the collagen present in the natural ECM is approached in three ways. These are electrospinning, molecular self-assembly and phase separation. Each method follows a different path to the end product, with different results. Electrospinning has been used to fabricate fibers from both natural macromolecules and synthetic biodegradable polymers [Citation5–12]. Self-assembly is a method by which individual molecules organize spontaneously into a well-defined and stable hierarchical structure [Citation13–15]. The first two methods can both successfully yield fibers within the range of collagen fibers [Citation16], but do not generate a well-defined macropore structure. Phase separation, on the other hand, has generated nano-fibers (figure ) in the same range as natural extracellular matrix collagen and allows for the inclusion of these more-complex structural elements [Citation17–19]. An artificial extracellular matrix with an interconnected pore structure has the potential to accommodate cells, provide nutrient transport and guide growth and subsequent tissue regeneration.
Figure 1 Scanning electron micrograph of nano-fibrous PLLA scaffold, prepared from 2.5% (w/v) PLLA/THF solution at a gelation temperature of 8 °C: (a) image at a low magnification; (b) image at a high magnification (reprinted with permission from [Citation18] ©1999 John Wiley and Sons Inc.).
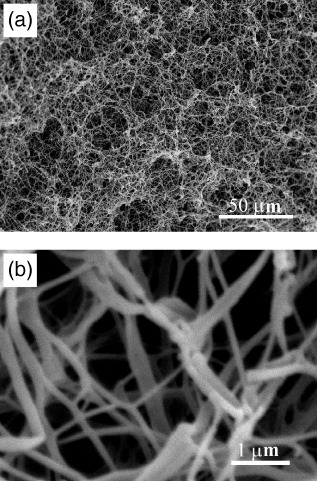
A biomimetic approach has been developed in which partially carbonated hydroxyapatite is grown onto previously fabricated, porous, polymer scaffolds using simulated body fluid (SBF) treatment [Citation20]. To improve this biomimetic process, various materials and processing parameters have been investigated to determine which combination leads to ideal apatite formation [Citation21]. This work is ongoing, but poly (L-lactide) scaffolds treated with SBF yielded a uniform distribution of apatite particles, and are therefore a system of interest (figure ) [Citation19].
Figure 2 Scanning electron micrographs of nano-fibrous PLLA/HA scaffold treated in SBF for (a) 4 days and (b) 8 days (reprinted with permission from [Citation19] ©2006 John Wiley and Sons Inc.).
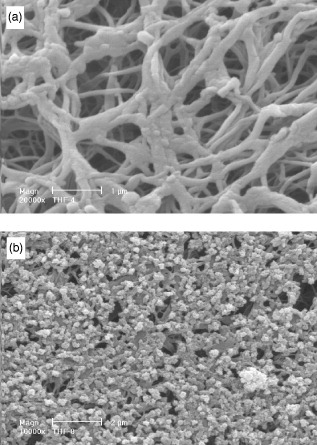
Cell response to nano-engineered scaffolds
Three-dimensional nano-fibrous scaffolds resulted in improved protein adsorption, an important part of the cell/surface interaction. This is not surprising, since it is known that the interaction of collagen fibers with integrins signals the expression of osteoblast phenotype genes such as osteocalcin (OCN) and bone sialoprotein (BSP) [Citation22, Citation23]. Scaffolds with nano-fibrous pore walls adsorbed four times more serum proteins than scaffolds with solid pore walls and selectively enhanced the adsorption of fibronectin and vitronectin. Additionally, nano-fibrous scaffolds led to a greater than 1.7-fold increase in osteoblastic cell attachment compared to solid-walled scaffolds [Citation24]. In a separate study, biomineralization was enhanced substantially on the nano-fibrous scaffolds compared to solid-walled scaffolds, and this was confirmed by von Kossa staining, measurement of calcium contents and scanning electron microscopy (figure ) [Citation25]. In support of this finding, osteoblasts cultured on the nano-fibrous scaffolds exhibited higher alkaline phosphatase activity and an earlier and enhanced expression of the osteoblast phenotype versus solid-walled scaffolds. These results demonstrate that a biomimetic scaffold incorporating a nano-fibrous architecture has great potential for tissue engineering.
Figure 3 Scanning electron microscope views of neonatal mouse osteoblasts on (a) solid-walled scaffold and (b, c) nano-fibrous scaffolds (reprinted with permission from [Citation25] ©2007 Elsevier Ltd).
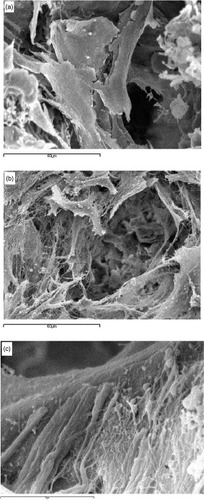
Compared with solid-walled scaffolds, nano-fibrous scaffolds supported substantially more new bone tissue formation in vivo. This was verified by micro-computed tomography measurement, von Kossa, Goldner's trichrome, Runx2 and bone sialoprotein (BSP) staining [Citation26].
Composite scaffolds of PLLA/HA led to improvement in cell survival over plain PLLA scaffolds [Citation27]. This is due to the fact that PLLA/HA scaffolds adsorbed 1.4 times the amount of serum protein and higher levels of fibronectin and vitronectin compared to PLLA controls, demonstrating that composite scaffolds are advantageous for bone tissue engineering.
Multiple non-chemical factors have a role in tissue development. For example, the physical support of ECM is an important part of bone development. By mimicking the physical features of the ECM, our group has been able to positively affect cell differentiation leading to subsequent tissue formation. To date, our nano-fibrous matrices have led to improved cellular behavior including osteoblastic cell morphology, cytoskeleton and adhesion structure, proliferation and differentiation versus flat films [Citation28], mouse embryonic stem cell (ESC) differentiation and mineralization versus flat films and thin fibrous constructs [Citation29], rhBMP-7 induced human amniotic fluid-derived stem cell (hAFSC) differentiation versus solid-walled scaffolds [Citation30], human bone marrow-derived mesenchymal stem cells (hMSCs) development along osteogenic and chondrogenic routes [Citation31].
Biomolecule delivery
The potential of using biodegradable polymer scaffolds as a vehicle for biologic factor delivery has been widely investigated by our group and others. Two basic approaches have been used to this point. The first is to directly incorporate the factors into the biodegradable scaffold itself, so that as biodegradation proceeds, the agent is released [Citation32–34]. The second approach is to incorporate either micro- or nano-spheres of degradable polymers with defined degradation rates, incorporated with the given factor, into the scaffold. These spheres then degrade, releasing the agent into the biologic environment in a controlled fashion [Citation35–37].
The first method was used with bFGF incorporated into a synthetic polymer matrix used as delivery devices for hepatocyte transplantation [Citation38]. Implantation of these devices increases angiogenesis into the device and increases hepatocyte engraftment. For example, specimens from the bFGF group showed a 2.5-fold increase in hepatocyte engraftment as compared with the control group. Similarly, the incorporation of bFGF in gelatin matrix also enhanced bone regeneration [Citation39].
The second method has been investigated by our group for its ability to better control protein release [Citation40]. The results illustrate the feasibility of achieving local delivery of proteins by a microsphere encapsulation technique. A bone morphogenetic protein (rhBMP-7) was released from nano-sphere-immobilized nano-fibrous scaffolds (figure ) and actively induced new bone formation [Citation41].
Figure 4 Scanning electron microscope views of nano-fibrous/macroporous PLLA scaffold incorporating PLGA microspheres (reprinted with permission from [Citation41] ©2007 Elsevier Ltd).
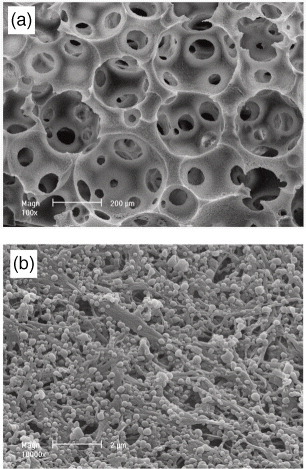
Similarly, platelet-derived growth factor-BB (PDGF-BB) was released from PLGA microspheres in PLLA nano-fibrous scaffolds [Citation42]. Sustained release was controlled through differing the molecular weight of the microspheres. The released PDGF-BB maintained its biological activity. PLGA microspheres in nano-fibrous scaffolds have been shown to control the release of rhPDGF-BB in vitro and were assessed in vivo in a soft tissue wound repair model in rats [Citation43]. The percentage of tissue invasion into microsphere-containing scaffolds was higher in the PDGF groups when compared to controls.
Moving forward
Tissue engineered scaffolds can serve as vehicles for the delivery of cells to the regeneration site in vivo. Nano-scale engineering of scaffold architecture is utilized to mimic the natural ECM, creating an artificial ECM that more actively direct cell growth and differentiation. Biologic cues are also incorporated into scaffold design in order to mimic the developmental process to further control cell behavior and tissue regeneration. These strategies can be utilized to investigate microenvironmental cues in development and to maximize scaffold function in tissue engineering and regenerative medicine.
References
- LangerRVacantiJ P 1993 Science 260 920 http://dx.doi.org/10.1126/science.8493529
- HenchL LPolakJ M 2002 Science 295 1014 http://dx.doi.org/10.1126/science.1067404
- MaP X 2008 Adv. Drug Deliv. Rev. 60 184 http://dx.doi.org/10.1016/j.addr.2007.08.041
- WeiGMaP X 2008 Adv. Funct. Mater. 18 3568 http://dx.doi.org/10.1002/adfm.200800662
- BolandE DWnekG ESimpsonD GPawlowskiK JBowlinG L 2001 J. Macromol. Sci. Pure Appl. Chem. 38 1231 http://dx.doi.org/10.1081/MA-100108380
- LuuY KKimKHsiaoB SChuBHadjiargyrouM 2003 J. Control. Release 89 341 http://dx.doi.org/10.1016/S0168-3659(03)00097-X
- MatthewsJ AWnekG ESimpsonD GBowlinG L 2002 Biomacromolecules 3 232 http://dx.doi.org/10.1021/bm015533u
- NairL SBhattacharyyaSLaurencinC T 2004 Expert Opin. Biol. Ther. 4 659 http://dx.doi.org/10.1517/14712598.4.5.659
- XuC YInaiRKotakiMRamakrishnaS 2004 Biomaterials 25 877 http://dx.doi.org/10.1016/S0142-9612(03)00593-3
- YangFMuruganRWangSRamakrishnaS 2005 Biomaterials 26 2603 http://dx.doi.org/10.1016/j.biomaterials.2004.06.051
- YoshimotoHShinY MTeraiHVacantiJ P 2003 Biomaterials 24 2077 http://dx.doi.org/10.1016/S0142-9612(02)00635-X
- ZongX HRanS FKimK SFangD FHsiaoB SChuB 2003 Biomacromolecules 4 416 http://dx.doi.org/10.1021/bm025717o
- BallP 1994 Nature 367 323 http://dx.doi.org/10.1038/367323a0
- WhitesidesG MGrzybowskiB 2002 Science 295 2418 http://dx.doi.org/10.1126/science.1070821
- ZhangS G 2002 Biotechnol. Adv. 20 321 http://dx.doi.org/10.1016/S0734-9750(02)00026-5
- RenekerD HChunI 1996 Nanotechnology 7 216 http://dx.doi.org/10.1088/0957-4484/7/3/009
- ChenV JMaP X 2004 Biomaterials 25 2065 http://dx.doi.org/10.1016/j.biomaterials.2003.08.058
- MaP XZhangR Y 1999 J. Biomed. Mater. Res. 46 60 http://dx.doi.org/10.1002/(SICI)1097-4636(199907)46:1<60::AID-JBM7>3.0.CO;2-H
- WeiGMaP X 2006 J. Biomed. Mater. Res. 78A 306 http://dx.doi.org/10.1002/jbm.a.30704
- ZhangRMaP X 1999 J. Biomed. Mater. Res. 45 285 http://dx.doi.org/10.1002/(SICI)1097-4636(19990615)45:4<285::AID-JBM2>3.0.CO;2-2
- ZhangRMaP X 2004 Macromol. Biosci. 4 100 http://dx.doi.org/10.1002/mabi.200300017
- MizunoMFujisawaRKubokiY 2000 J. Cell. Physiol. 184 207 http://dx.doi.org/10.1002/1097-4652(200008)184:2<207::AID-JCP8>3.0.CO;2-U
- XiaoGWangDBensonM DKarsentyGFranceschiR T 1998 J. Biol. Chem. 273 32988 http://dx.doi.org/10.1074/jbc.273.49.32988
- WooK MChenV JMaP X 2003 J. Biomed. Mater. Res. A 67 531 http://dx.doi.org/10.1002/jbm.a.10098
- WooK MJunJ HChenV JSeoJBaekJ HRyooH MKimG SSomermanM JMaP X 2007 Biomaterials 28 335 http://dx.doi.org/10.1016/j.biomaterials.2006.06.013
- WooK MChenV JJungH MKimT IShinH IBaekJ HRyooH MMaP X 2009 Tissue Eng. A 15 2155 http://dx.doi.org/10.1089/ten.tea.2008.0433
- WooK MSeoJZhangRMaP X 2007 Biomaterials 28 2622 http://dx.doi.org/10.1016/j.biomaterials.2007.02.004
- HuJLiuXMaP X 2008 Biomaterials 29 3815 http://dx.doi.org/10.1016/j.biomaterials.2008.06.015
- SmithL ALiuXHuJWangPMaP X 2009 Tissue Eng. A 15 1855 http://dx.doi.org/10.1089/ten.tea.2008.0227
- SunHFengKHuJSokerSAtalaAMaP X 2010 Biomaterials 31 1133 http://dx.doi.org/10.1016/j.biomaterials.2009.10.030
- HuJFengKLiuXMaP X 2009 Biomaterials 30 5061 http://dx.doi.org/10.1016/j.biomaterials.2009.06.013
- BonadioJSmileyEPatilPGoldsteinS 1999 Nat. Med. 5 753 http://dx.doi.org/10.1038/10473
- KanematsuAYamamotoSOzekiMNoguchiTKanataniIOgawaOTabataY 2004 Biomaterials 25 4513 http://dx.doi.org/10.1016/j.biomaterials.2003.11.035
- KimHKimH WSuhH 2003 Biomaterials 24 4671 http://dx.doi.org/10.1016/S0142-9612(03)00358-2
- LangerR 1998 Nature 392 5
- CooperA I 2000 J. Mater. Chem. 10 207 http://dx.doi.org/10.1039/a906486i
- HileD DAmirpourM LAkgermanAPishkoM V 2006 J. Control. Release 66 177 http://dx.doi.org/10.1016/S0168-3659(99)00268-0
- LeeHCusickR ABrowneFHo KimTMaP X 2002 Transplantation 73 1589 http://dx.doi.org/10.1097/00007890-200205270-00011
- AkagawaYKuboTKoretakeKHayashiKDoiKMatsuuraAMoritaKTakeshitaRYuanQTabataY 2009 J. Prosthodont. Res. 53 41 http://dx.doi.org/10.1016/j.jpor.2008.08.009
- WeiGPettwayG JMcCauleyL KMaP X 2004 Biomaterials 25 345 http://dx.doi.org/10.1016/S0142-9612(03)00528-3
- WeiGJinQGiannobileW VMaP X 2007 Biomaterials 28 2087 http://dx.doi.org/10.1016/j.biomaterials.2006.12.028
- WeiGJinQGiannobileW VMaP X 2006 J. Control. Release 112 103 http://dx.doi.org/10.1016/j.jconrel.2006.01.011
- JinQWeiGLinZSugaiJ VLynchS EMaP X 2008 PLoS One 3 e1729 http://dx.doi.org/10.1371/journal.pone.0001729