Abstract
Nanobiomaterials can play a central role in regenerative medicine and tissue engineering by facilitating cellular behavior and function, such as those where extracellular matrices (ECMs) direct embryonic stem (ES) cell morphogenesis, proliferation, differentiation and apoptosis. However, controlling ES cell proliferation and differentiation using matrices from natural sources is still challenging due to complex and heterogeneous culture conditions. Moreover, the systemic investigation of the regulation of self-renewal and differentiation to lineage specific cells depends on the use of defined and stress-free culture conditions. Both goals can be achieved by the development of biomaterial design targeting ECM or growth factors for ES cell culture. This targeted application will benefit from expansion of ES cells for transplantation, as well as the production of a specific differentiated cell type either by controlling the differentiation in a very specific pathway or by elimination of undesirable cell types.
Introduction
Soluble factors, cell–cell and cell–matrix interactions are most important effectors of stem cell fate [Citation1]. Among them, cell–matrix interaction plays a fundamental role in regulating cell proliferation, migration, differentiation and survival [Citation2]. Both biologically derived and synthetic materials have been explored as an extracellular matrix (ECM) in regenerative medicine and tissue engineering. In general, materials from natural sources (e.g. collagen, laminin or fibronectin) are advantageous for cell culture because of the presence of cell recognizable receptors, known as integrin-dependent interaction. Moreover, in integrin-independent interaction some carbohydrates can specifically recognize cell surface molecules (e.g. galactose can act as a specific ligand for asialoglycoprotein receptor (ASGPR) on hepatocytes) [Citation3, Citation4]. However, complexities associated with natural materials, including complex structural composition, purification, immunogenicity and pathogen transmission have driven the development of synthetic biomaterials for use as 2D or 3D extracellular microenvironments to mimic the regulatory characteristics of natural ECMs and ECM-bound growth factors [Citation4, Citation5]. The designing of artificial ECM should enable more efficient and scalable culture of ES cells, as well as greater control over material properties and tissue responses [Citation6, Citation7]. Currently, engineered ECM using recombinant technology has gained much attention for construction of novel artificial matrix due to simple and cheap production and purification protocols.
Until recently there has been a huge effort on ES cell research; however, this field is facing many unsettled problems, including necessity of huge cell number for transplantation, enzymatic stress during repeated passages, presence of xenogenetic compounds, expensive culture conditions and necessity of cell sorting for specific lineage of cells [Citation8]. The use of defined and selective culture conditions containing synthetic substrates can be an alternative to overcome such type of problems and to make the ES cell system practical for routine research and clinical applications.
This review summarizes some of the most recent developments on the construction of novel ECM using genetically engineered adhesion molecules or growth factors for ES cell culture. It is expected that development of a new recombinant ECM for tissue engineering and regenerative medicine will play a significant role in providing an alternative to organ or tissue transplantation, both of which are now suffering from a limited supply of normal cells from embryo. So far, relatively less effort has been made on ECM to regulate ES cells or induced pluripotent (iPS) cell proliferation and differentiation.
Pluripotency
Pluripotency is characterized by the ability of a stem cell to self-renew indefinitely while maintaining the capacity to differentiate into derivatives of all three germ layers (ectoderm, endoderm and mesoderm). Pluripotency is the key for ES cells as they can be used for appropriate cell proliferation, cell cycle regulation, gene expression and differentiation. Oct3/4, Nanog and Sox2 are three important transcription factors that can control the maintenance of pluripotency of mouse ES cells by inhibiting differentiation to three germ layers [Citation10–12]. For the maintenance of pluripotency, mouse ES cells can be cultured on a feeder layer of mouse embryonic fibroblasts (MEF) or incubated with MEF derived soluble or immobilized form of leukocyte inhibitory factor (LIF) (figures (a) and (b)) [Citation6]. The standard tests of pluripotency are embryoid body formation in vitro, chimera formation into the recipient blastocyst and teratoma formation after injection into immunocompromised mice (figure (c)) [Citation9]. Although the molecular basis of pluripotency has been studied mostly using mouse ES cells, recent high-throughput studies of target genes to which Oct3/4 and Nanog bind in both mouse and human ES cells suggest that many of the important downstream genes are regulated by common mechanisms in the two species [Citation13]. Compared with the generation of ectoderm, endoderm, mesoderm and germ cells for demonstration of pluripotency, little is known about molecular mechanisms that govern ES cell differentiation. This might be due to lack of homogeneous and defined culture condition and generation of suitable single cell culture.
Figure 1 Characterization of the pluripotency of ES cells on artificial extracellular matrix using recombinant fusion protein of E-cadherin and Fc-region of IgG. (a) Leukocyte inhibitory factor (LIF)-dependent activation of STAT3 blocks ES cell differentiation and promotes self-renewal. Oct3/4 is the most important transcription factor in this pathway. (b) Immunocytochemical labeling shows undifferentiated mouse ES cells with positive reactivity for Oct3/4 on artificial ECM (left side) and colony of undifferentiated mouse ES cells on conventional culture system (right side). (c) H&E staining of teratomas as a standard test of pluripotency suggesting the potential of ES cells on artificial matrix into ectoderm, endoderm and mesoderm [Citation7].
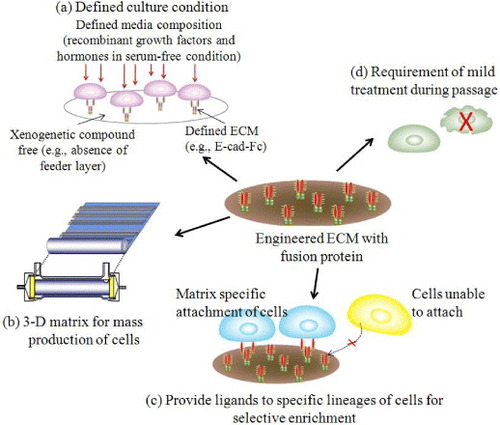
Extracellular microenvironment
Tissue formation, function and regeneration after damage is the result of a balanced interaction of numerous individual cell fate processes, each of which is induced by an array of signals originating from the extracellular environment. The extracellular microenvironment, which surrounds each cells and comprises the molecular signals, has three important effectors: (i) insoluble natural matrix molecule (collagen, laminin, elastin or fibronectin), (ii) soluble macromolecules (growth factors, chemokines and cytokines) and (iii) proteins on surfaces of neighboring cells (cadherins) (figure ). Thus ultimate fate of a cell to proliferate, differentiate, migrate, apoptose or perform other specific functions is a coordinated response to the molecular interactions with these ECM effectors [Citation4]. Naturally derived ECM has proved effective in many basic and clinical applications. However, the need for synthetic matrices for tissue-specific cell biological investigations is inevitable to gain more control over the material and thus over the cellular behavior they induced.
Figure 2 Interactions of stem cells with extracellular microenvironments and its effect on cell behavior. Stem cell fate is influenced by coordinated interaction of soluble factors, extracellular matrix and signals from neighboring cells. Specific binding of signal molecules with cell-surface receptors induces complex intracellular signaling pathways with subsequent effect on gene expression, self-renewal, morphogenesis and differentiation [Citation1, Citation4].
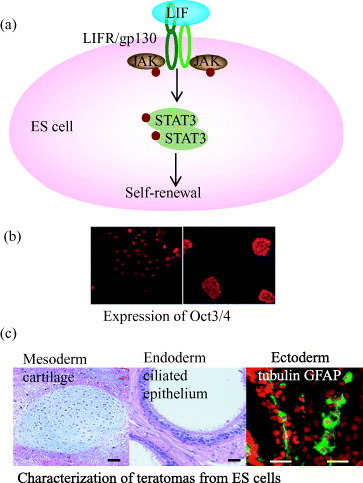
Natural extracellular matrices
Structurally, natural ECMs are gels composed of various protein fibers or fibrils interwoven within a hydrated network of glycosaminoglycan chains [Citation4]. Various amounts and types of collagens, laminin, elastin, fibronectin, vitronectin, or proteoglycans act as an extracellular matrix. Generally, heptotocytes adhere well to collagen or laminin ECMs, although they exhibit low levels of liver specific synthetic functions [Citation3]. Gelatin, which is extensively used in myocardial and neural tissue engineering, can provoke an unspecific inflammatory response [Citation15]. Recently, it has been shown that natural matrix molecule (fibronectin-coated collagen gel) can induce the directed differentiation of endoderm from mES cells without the use of activin or cell sorting [Citation16]. However, due to less control on structural composition of ECM, cell–matrix interaction and use of complex serum-supplementation, it was difficult to find the exact role of collagen gel on directed differentiation of endodermal cells. Moreover, there has been some controversy regarding matrigel for ES cell culture, which has been partially regarded as a potential contaminant. Furthermore, some of these components have no regulatory approval for use in human [Citation15].
Artificial extracellular matrices
In recent years, artificial ECMs gained special interest due to their excellent mechanical properties, processability and low cost [Citation17]. It is expected that synthetic ECMs can mimic many functions of the natural ECM including cellular 3D architecture, mechanical integrity to the new tissue and the space for the diffusion of nutrients and metabolites [Citation18]. Figure shows novel strategies for designing engineered ECM to overcome the problem of complex natural matrices. Ideally, these engineered matrix molecules have the potentiality to give selectivity to specific lineages of cells or tissues.
Figure 3 Design strategies for cell–matrix engineering based on our originally proposed concept to induce specific cellular behavior. The receptor-binding domain of naturally occurring proteins can be modified by recombinant technology. The most important components could be integrin dependent ligands (such as collagen, laminin or fibronectin), cell–cell adhesion molecules (such as cadherins or ICAM), binding sites for growth factor proteins (such as EGF, HGF and LIF) or small molecules (such as drugs or hormones) to make it easily accessible to cells, and also ligands for endocytosis (such as LDL, transferrin, or ASGP-R). The use of such recombinant approaches in ECM design may allow matrices to be selective for a specific cell or tissue in a homogeneous environment [Citation3, Citation5–7, Citation19, Citation23, Citation24, Citation26]. LN, laminin; FN, fibronectin; ICAM, intercellular adhesion molecule; LDL, low density lipoprotein.
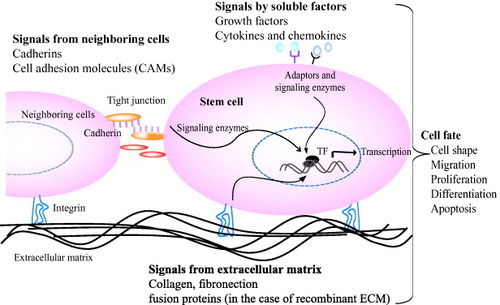
Extracellular matrices with immobilized fusion protein as ligand
The ECM provides bound multifunctional adhesion molecules, including fibronectin, vitronectin and laminin that guide the development and maintenance of cell function. The integrins function as the principle receptors of animal cells for many of these adhesion molecules [Citation4]. On the other hand, it has been known that E-cadherin mediated cell–cell interaction is essential for ES cell morphogenesis and maintenance of organized solid tissue [Citation20, Citation21]. Moreover, E-cadherin may fine-tune ES cell pluripotency by modulating intracellular β-catenin level or Nanog expression [Citation9]. E-cadherin can also regulate differentiation of ES cells by modulating its expression or expression of transcription factors [Citation22]. Nagaoka et al [Citation7, Citation19] showed that the use of artificial ECM in absence of natural adhesion molecules can support both undifferentiated and differentiated cells to function according to their native counterparts. Designing of artificial ECM with immobilized extracellular domain of E-cadherin as insoluble ligands not only facilitated efficient proliferation of mouse ES (mES) cells with scattering behavior [Citation23] but also improved cell attachment and differentiation of primary hepatocytes [Citation19]. In chimeric protein of E-cadherin extracellular domain and IgG-Fc region (abbreviated as E-cad-Fc) (figure ), the E-cadherin primarily attaches to ECM through Fc region that has the potentiality to stably adsorb to a plastic surface such as polystyrene and dimerize via the hinge region. On the other hand, the extracellular domain of E-cadherin holds cells through homophilic interaction and thereby, activates specific signaling pathways. Designing of this defined artificial matrix molecules can improve mechanical performance and help in finding the complexity of signaling in cell–ECM interactions [Citation7, Citation19]. Relevant studies based on synthetic ECM include (i) influence on cell migration in 2D or 3D cultures, in modified biopolymer matrices (e.g. poly (N-p-vinylbenzyl-4-O-β-D-galactopyranosyl-D-gluconamide); PVLA) [Citation24] and synthetic gels [Citation25] and (ii) signaling pathways in cell proliferation and differentiation [Citation23, Citation26]. These studies provide several examples that well controlled matrices can yield insight into basic cell biological principles.
Figure 4 Construction of chimera proteins. Generated segments of an extracellular domain of mouse E-cadherin, leukocyte inhibitory factor (LIF) or epidermal growth factor (EGF) and an IgG-Fc region were subcloned into a eukaryotic expression vector pRC/CMV via HindIII–NotI site and NotI–XbaI site, respectively [Citation5–7, Citation19]. ECM using chimera proteins has specific applications, several of which are listed in (a)–(c).
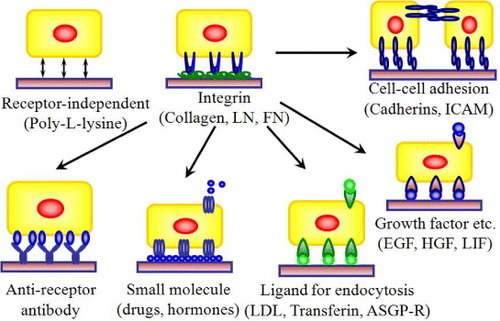
Extracellular matrices with immobilized fusion protein of soluble factor
The biological signals of growth factors and cytokines are mediated by two different forms, the secreted form and the cell membrane- or matrix-anchored form, which release different signal transduction cascades [Citation27]. Controlled release of growth factors from engineered ECM can facilitate analysis of cellular morphogenesis, cell–cell interaction and monitoring of signaling pathways [Citation19]. It has also been reported that artificial extracellular matrix with immobilized recombinant growth factors differently activate signal transduction molecules than their soluble forms together with different morphological changes in the cytoskeleton. Compared to soluble form, immobilized recombinant epidermal growth factor (EGF-Fc) and hepatocyte growth factor (AeHGF-Fc) showed more strong and stable activation of MAPK in A431 and Akt in HepG2 cells, respectively [Citation5, Citation28]. Besides, as growth factors are required in only very tiny quantities to elicit biological response, designing artificial matrices for controlled growth factor presentation is necessary. Recently, many studies showed the difficulties in using soluble LIF to control self-renewal of mES cells without differentiation [Citation29]. Considering these limitations together with analysis of cell behavior and function, recently it has been reported that matrix anchored form of LIF, i.e., LIF-Fc coated matrix, can maintain undifferentiated state of mES cells (figure ). Furthermore, when cultured on the co-immobilized surface with LIF-Fc and E-cad-Fc, mES cells showed undifferentiated state and pluripotency without additional LIF supplementation. This study showed that immobilized LIF and E-cadherin can maintain mES cells efficiently and that the immobilized form of LIF-Fc fusion protein is useful for the investigation of signaling pathways of LIF in the maintenance ES cell pluripotency [Citation6]. However, it is still unclear to control ES cell proliferation on artificial ECM using only LIF as a potential inhibitor of differentiation.
Culture of ES cells
Pluripotent stem cell lines can be derived from inner cell mass of blastocyst, which yield ES cells. Despite clear morphological differences and different growth factor requirements, human ES cells are thought to be equivalent to mES cells at molecular level [Citation9].
Current protocols of ES cell culture
So far, three specific culture conditions of mES cells for proliferation and differentiation were used: embryoid body (EB) formation [Citation30]; cultures on feeder cells [Citation31]; and cultures on matrices [Citation7]. Currently, the most popular protocol is to make an embryo-like structure known as the EB, in which cells form tight shiny colonies and most cell lineages differentiate spontaneously (figure ). The basic idea behind the EB protocol is to mimic the process of embryonic development [Citation13]. Most cell lineages, including the neuronal, mesoderm and hepatocyte lineages have been shown to be generated within the EB [Citation32–34]. However, the structure of the EB is far more heterogeneous than the actual embryo. Moreover, monitoring cell behavior and guided differentiation to specific cell lineages is not possible without the use of laborious cell sorting [Citation7, Citation13]. In contrast, monolayers of freshly isolated cells or cell lines are often used as feeder cells to support ES-cell activities. The maintenance of human ES cells still requires feeder cells because these cells do not respond to LIF [Citation35]. Under certain circumstances, a highly selective microenvironment is provided by feeder cells. Shiraki et al [Citation36] showed that embryonic stem cells that differentiate on M15 cells have the capability to differentiate into all three germ layers. However, the difficulties for using feeder cell lines include maintenance of stromal cell, undefined cultural condition, analysis of morphogenesis, differentiation of ES cells to multiple lineages (figure ) [Citation13]. Moreover, for therapeutic applications, feeder cells may be the source of pathogen transmission.
Figure 5 Comparison of two different culture conditions: colony formation and single cell culture. Embryoid body and cells on feeder layer favor the formation of colony of aggregated cells (left side) [Citation30, Citation31]. Cells on matrices (right side) favor single cell culture with homogeneous culture conditions [Citation7]. Specific merits and limitations are enumerated in the figure for these culture conditions. Scale bar: 100 μm.
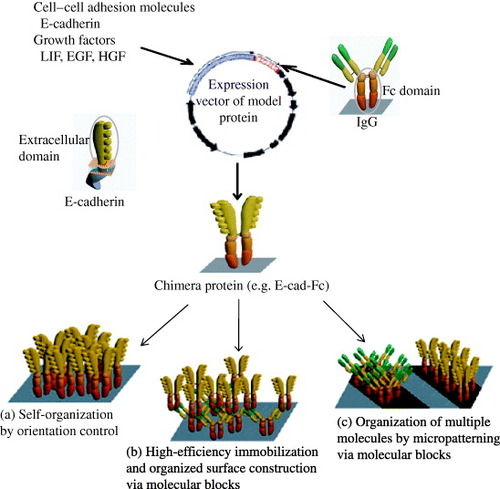
Single cell culture on matrices
Until recently few successes have been reported on proliferation of ES cells in single cell cultures with homogeneous environment. Nagaoka et al [Citation7] showed that mES cells cultured on E-cad-Fc coated surface had unique morphological characters (figure (a)), complete ES cell features, higher proliferative ability and transfection efficiency than those grown under conventional conditions. Furthermore, they require less LIF, probably due to the homogeneous exposure of cells to this cytokine (figures (b) and (c)). It has also been reported that cell–cell adhesion molecules in undifferentiated ES cell colonies can be distributed heterogeneously [Citation37]. These observations indicate that an aggregated colony formation with close cell–cell communications may generate a heterogeneous environment within the colonies, which potentially inhibit the proliferation of ES cells and the distribution of soluble factors. Nagaoka et al [Citation19] also showed that differentiated embryonic stem cells can be cultured and proliferate efficiently on recombinant ECM suggests the suitability of engineered ECM for differentiation of ES cells especially to mature hepatocytes (figure (d)) [Citation23, Citation28]. Moreover, the analysis of signal transduction on a discrete single cell is still difficult or impossible under the colony-forming conditions because intercellular cross talk cannot be discounted. Thus, the single cell culture may hold the key for detailed investigation of signaling pathways and cell responses to growth factors, cytokines and drugs using single cell based assays. Importantly, the establishment of an efficient ES cell culture system will allow investigation of many physiological mechanisms in development and cell behavior in vivo or in vitro.
Figure 6 Cell morphology, adhesion and transfection efficiency of mES cells and hepatocytes on the fusion protein-immobilized surfaces. (a) Morphological observation of mES cells (EB3) on a gelatin- or E-cad-Fc-coated surface. Scale bar: 50 μm. (b) The higher proliferative activity of EB3 cells on 5 μg ml−1 of E-cad-Fc coated matrix compared to 0.1% gelatin coated surfaces in the presence of LIF for 2 days. Even at lower concentration of immobilized LIF (LIF-Fc) at 55 units well−1, the ES cells showed higher proliferation ability on a co-immobilized surface than on a gelatinized surface. (c) Transfection efficiency of EB3 cells on two different matrices. The data indicate means±SEM. §:P<0.001 versus gelatinized plates. (d) Primary hepatocytes could adhere to E-cad-Fc-coated dish as well as collagen-coated surface after 4 h [Citation6, Citation7, Citation19].
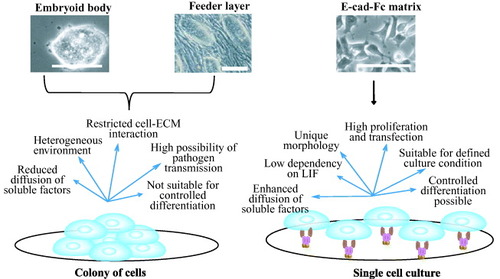
Development of fully defined culture conditions
For establishment of reproducible and optimal growth conditions for ES cell proliferation and differentiation, a defined culture condition with artificial extracellular matrix, recombinant soluble factors, and single cell culture will be the ultimate target. Moreover, ideal culture conditions can provide phenotypically and genetically stable cultures for appropriate, reproducible and directed differentiation. The purpose of using artificial extracellular matrices towards development of defined culture condition has many folds: (i) proliferation of cells on fully defined substrates for homogeneous culture condition, (ii) mimic the process of cell differentiation, (iii) generation of specific type of mature cells at a high purity and (iv) elimination of xenogenetic components from culture [Citation6, Citation7, Citation38]. Bearing these intractable fields of study in mind, continuous attempts have been made to proliferate and differentiate ES cell under homogeneous conditions without EB formation.
Serum/feeder layer free culture
Considering the systematic investigation of the regulation of self-renewal and the production of safer sources of cells for transplantation, a number of new culture methods for ES cells have recently been developed towards the elimination of xenogenetic components (e.g. fetal bovine serum, feeder layer of mouse embryonic fibroblast) for ES cell culture and differentiation. However, the complete elimination of xenogenetic compounds from ES cell culture not yet possible due to presence of serum, enzymes or natural matrices on which the cells grow [Citation32, Citation39–42]. Moreover, these methods generate aggregated undifferentiated cells, which can inhibit diffusion of soluble factors to cells and thereby, affect the pluripotency of the cultured cells. A critical next step would be the derivation of ES cells in defined-medium condition.
Repeated ES cell culture
Cell–cell and cell–matrix interactions of mES cells has presented several challenges in the attempt to detach the cells and seed again to a new tissue plate in a consistent manner without providing any significant stress. Passaging of mES cells can be achieved chemically, mechanically or enzymatically. Mechanical passaging by creating shear force generates colony of cells with variable size and distribution. Enzymatic passaging involves the treatment of cells with enzymes (e.g. trypsin, accutase or collagenase) to allow dissociation of the cells. Although passaging using enzymes generates single cells with uniform distribution, this method provide stress on cells by damaging cell surface molecules or DNA [Citation38]. Therefore, passaging technique involving chemical compounds could be one step forward to reach uniform dispersal and consistent plating of ES cells. In this regard, artificial extracellular matrix with recombinant proteins may be an attractive field to achieve fully defined xeno-free culture conditions.
Conclusion and future perspectives
In this article, we review nanobiomaterials as ECMs for mouse ES cell culture. The E-cadherin recombinant protein mediate ES cell adherence on ECM through homophilic interaction and generate homogeneous single cell cultures with high ES cell proliferation, transfection and diffusion of soluble factors. These advantageous features open a new area to use this system for directed differentiation into specific cell lineages (figure ). For ES-cell differentiation in monolayer cultures under chemically defined conditions, only a few successes have been reported. Ying et al [Citation32] showed that differentiation of ES cells to neuronal cells can be induced on gelatin-coated dishes with a conventional serum-free medium. Researchers also reported two serum-free conditions for definitive endoderm and visceral endoderm that are based on the serum-free medium in the presence of insulin, transferrin and bovine serum albumin [Citation22]. Under these conditions, the purity of the cells was achieved after laborious cell sorting using flow cytometry, which is practically impossible to billions of differentiated cells for therapeutic applications. Therefore, single cell culture on artificial extracellular matrix using the chimeric proteins of E-cadherin or growth factors could be the ultimate target to obtain homogeneous population of non-stressed differentiated cells at high efficiencies.
Figure 7 Future prospects of engineered ECM for ES cells. (a) The use of recombinant extracellular matrix together with defined media supplemented with recombinant proteins and elimination of xenogenetic components would be one step forward to develop defined culture condition. (b) Three-dimensional (3D) culture systems using recombinant ECM molecule and different combinations of growth factors and hormones would allow highly efficient and mass production of ES cells for therapeutic applications. (c, d) The guided differentiation of ES cells under fully defined culture condition with lineage-specific matrices would provide the opportunity for genesis of economically feasible, purified and non-stressed cells for proliferation and differentiation.
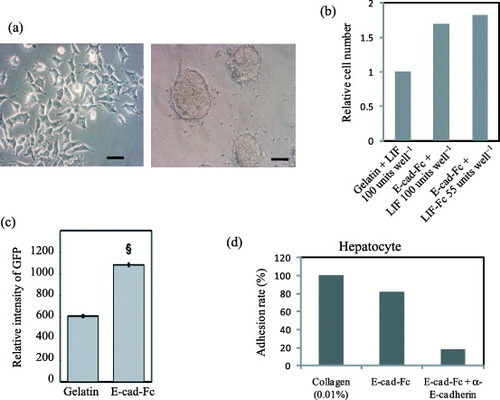
Acknowledgment
This work was supported in part by the ministry of education, culture, sports, science and technology.
References
- DischerD EMooneyD JZandstraP W 2009 Science 324 1673 http://dx.doi.org/10.1126/science.1171643
- HynesR O 1999 Trends Cell Biol. 9 M33 http://dx.doi.org/10.1016/S0962-8924(99)01667-0
- ChoC SSeoS JParkI KKimS HKimT HHoshibaTHaradaIAkaikeT 2006 Biomaterials 27 576 http://dx.doi.org/10.1016/j.biomaterials.2005.06.008
- LutolfM PHubbellJ A 2005 Nat. Biotechnol. 23 47 http://dx.doi.org/10.1038/nbt1055
- OgiwaraKNagaokaMChoC SAkaikeT 2005 Biotechnol. Lett. 27 1633 http://dx.doi.org/10.1007/s10529-005-2605-0
- NagaokaMHagiwaraYTakemuraKMurakamiYLiJDuncanS AAkaikeT 2008 J. Biol. Chem. 26 26468 http://dx.doi.org/10.1074/jbc.M805037200
- NagaokaMKoshimizuUYuasaSHattoriFChenHTanakaTOkabeMFukudaKAkaikeT 2006 PLoS One 1 e15 http://dx.doi.org/10.1371/journal.pone.0000015
- ChaseL GFirpoM T 2007 Curr. Opin. Chem. Biol. 11 367 http://dx.doi.org/10.1016/j.cbpa.2007.06.421
- ChouY FChenH HEijpeMYabuuchiAChenowethJ GTesarPLuJMckayR DGeijsenN 2008 Cell 135 449 http://dx.doi.org/10.1016/j.cell.2008.08.035
- NicholsJZevnikBAnastassiadisKNiwaHKlewe-NebeniusDChambersIScholerHSmithA 1998 Cell 95 379 http://dx.doi.org/10.1016/S0092-8674(00)81769-9
- MitsuiKTokuzawaYItohHSegawaKMurakamiMTakahashiKMaruyamaMMayedaMTamanakaS 2003 Cell 113 631 http://dx.doi.org/10.1016/S0092-8674(03)00393-3
- AvilionA ANicolisS KPevnyL HPerezLVivianNLovell-BadgeR 2003 Genes Dev. 17 126 http://dx.doi.org/10.1101/gad.224503
- NishikawaSJaktL MEraT 2007 Nat. Rev. Mol. Cell Biol. 8 502 http://dx.doi.org/10.1038/nrm2189
- KleinmanH KPhilpDHoffmanM P 2003 Curr. Opin. Biotechnol. 14 526 http://dx.doi.org/10.1016/j.copbio.2003.08.002
- AkhyariPKamiyaHHaverichAKarckMLichtenbergA 2008 Eur. J. Cardiothorac. Surg. 34 229 http://dx.doi.org/10.1016/j.ejcts.2008.03.062
- ParashuramaNNahmiasYChoC Hvan PollDTillesA WBerthiaumeFYarmushM L 2008 Stem Cells 26 474 http://dx.doi.org/10.1634/stemcells.2007-0303
- GuptaBPlummerCBissonIFreyPHilbornJ 2002 Biomaterials 23 863 http://dx.doi.org/10.1016/S0142-9612(01)00195-8
- PutnamA JMooneyD J 1996 Nat. Med. 2 824 http://dx.doi.org/10.1038/nm0796-824
- NagaokaMIseHAkaikeT 2002 Biotechnol. Lett. 24 1857 http://dx.doi.org/10.1023/A:1020905532227
- TakeichiM 1995 Curr. Opin. Cell Biol. 7 619 http://dx.doi.org/10.1016/0955-0674(95)80102-2
- BrieherW MYapA SGumbinerB M 1996 J. Cell Biol. 135 487 http://dx.doi.org/10.1083/jcb.135.2.487
- YasunagaMTadaSTorikai-NishikawaSNakanoYOkadaMJaktL MNishikawaSChibaTEraTNishikawaS 2005 Nat. Biotechnol. 23 1542 http://dx.doi.org/10.1038/nbt1167
- NagaokaMIseHHaradaIKoshimizuUMaruyamaAAkaikeT 2008 J. Cell Biochem. 103 296 http://dx.doi.org/10.1002/jcb.21406
- KobayashiAGotoMKobayashiKAkaikeT 1994 J. Biomater. Sci. Polym. Ed. 6 325 http://dx.doi.org/10.1163/156856295X00030
- GobinA SWestJ L 2002 FASEB J. 16 751
- KimS HAkaikeT 2007 Tissue Eng. 13 601 http://dx.doi.org/10.1089/ten.2006.0104
- TanakaYKimataKAdamsD HEtoS 1998 Proc. Assoc. Am. Phys. 110 118
- AzumaKNagaokaMChoC SAkaikeT 2009 Biomaterials 31 802 http://dx.doi.org/10.1016/j.biomaterials.2009.09.105
- YingQ LNicholsJChambersISmithA 2003 Cell 115 281 http://dx.doi.org/10.1016/S0092-8674(03)00847-X
- DoetschmanT CEistetterHKatzMSchmidtWKemlerR 1985 J. Embryol. Exp. Morphol. 87 27
- NakanoTKodamaHHonjoT 1994 Science 265 1098 http://dx.doi.org/10.1126/science.8066449
- YingQ LStavridisMGriffithsDLiMSmithA 2003 Nat. Biotechnol. 21 183 http://dx.doi.org/10.1038/nbt780
- DasguptaAHugheyRLancinPLarueLMogheP V 2005 Biotechnol. Bioeng. 92 257 http://dx.doi.org/10.1002/bit.20676
- ToyookaYTsunekawaNAkasuRNoceT 2003 Proc. Natl Acad. Sci. USA 100 11457 http://dx.doi.org/10.1073/pnas.1932826100
- ThomsonJ AKalishmanJGolosT GDurningMHarrisC PHearnJ P 1996 Biol. Reprod. 55 254 http://dx.doi.org/10.1095/biolreprod55.2.254
- ShirakiNHiguchiYHaradaSUmedaKIsagawaTAburataniHKumeKKumeS 2009 Biochem. Biophys. Res. Commun. 381 694 http://dx.doi.org/10.1016/j.bbrc.2009.02.120
- CuiLJohkuraKYueFOgiwaraNOkouchiYAsanumaKSasakiK 2004 J. Histochem. Cytochem. 52 1447 http://dx.doi.org/10.1369/jhc.3A6241.2004
- HoffmanL MCarpenterM K 2005 Nat. Biotechnol. 23 699 http://dx.doi.org/10.1038/nbt1102
- XuCInokumaM SDenhamJGoldsKKunduPGoldJ DCarpenterM K 2001 Nat. Biotechnol. 19 971 http://dx.doi.org/10.1038/nbt1001-971
- AmitMSharikiCMarguletsVItskovitz-EldorJ 2004 Biol. Reprod. 70 837 http://dx.doi.org/10.1095/biolreprod.103.021147
- SatoNMeijerLSkaltsounisLGreengardPBrivanlouA H 2004 Nat. Med. 10 55 http://dx.doi.org/10.1038/nm979
- JamesDLevineA JBesserDHemmati-BrivanlouA 2005 Development 132 1273 http://dx.doi.org/10.1242/dev.01706