Abstract
Mesenchymal stem cells (MSCs) are adult stem cells which show differentiation capabilities toward various cell lineages. We have already used MSCs for treatments of osteoarthritis, bone necrosis and bone tumor. For this purpose, culture expanded MSCs were combined with various ceramics and then implanted. Because of rejection response to allogeneic MSC implantation, we have utilized patients' own MSCs for the treatment. Bone marrow is a good cell source of MSCs, although the MSCs also exist in adipose tissue. When comparing osteogenic differentiation of these MSCs, bone marrow MSCs show more extensive bone forming capability than adipose MSCs. Thus, the bone marrow MSCs are useful for bone tissue regeneration. However, the MSCs show limited proliferation and differentiation capabilities that hindered clinical applications in some cases. Recent advances reveal that transduction of plural transcription factors into human adult cells results in generation of new type of stem cells called induced pluripotent stem cells (iPS cells). A drawback of the iPS cells for clinical applications is tumor formation after their in vivo implantation; therefore it is difficult to use iPS cells for the treatment. To circumvent the problem, we transduced a single factor of either SOX2 or NANOG into the MSCs and found high proliferation as well as osteogenic differentiation capabilities of the MSCs. The stem cells could be combined with bioceramics for clinical applications. Here, we summarize our recent technologies using adult stem cells in viewpoints of bone tissue regeneration.
Introduction
Stem cells are very different from other cell types because they can show high proliferation activity in which they can renew themselves through cell division even after long periods of in vitro culture [Citation1–3]. They can also become various types of tissue—specific cells such as neural, hepatic, bone, cartilage, vascular cells and more. There are two types of stem cells, one is embryonic stem cells (ES cells) [Citation4, Citation5] and the other is somatic or adult stem cells. Embryonic stem cells are isolated from embryos that develop after in vitro fertilization. Several days after fertilization, the embryo forms cellular clumps called blastocyst. The blastocyst includes a layer of cells that forms the exterior surface of the inner cell mass. The cells of the inner cell mass are the source of ES cells. These cells can be removed and cultured for long periods without differentiation. In 1998, Thomson et al at the University of Wisconsin developed a number of human embryonic stem cell lines [Citation6]. Because embryos are sacrificed to obtain the stem cells, there are ethical issues to use the ES cells for basic as well as clinical research.
In contrast to the ES cells, somatic or adult stem cells can be found in various adult tissues. Especially, bone marrow is a well-characterized source of adult stem cells such as hematopoietic stem cells and mesenchymal stem cells (MSCs) [Citation7–10]. Although the adult stem cells can show high proliferation as well as differentiation capability towards various tissue specific cells [Citation11–14], these capabilities are limited. Even though the cells have limitations, they are derived from adult tissue, such as bone marrow which can be obtained with needle aspiration; this reduces the ethical problems. Furthermore, they already have been used for cell-based therapies to treat disease, which is also referred to as regenerative medicine [Citation15–17].
In addition to these ES and adult stem cells, in 2006, Japanese researchers made a breakthrough by establishing conditions that induce a variety of adult cells to be reprogrammed and show a stem cell state [Citation18]. This new type of stem cells is called induced pluripotent stem cells (iPS cells) [Citation18–21]. In this review, we focus on adult stem cells, especially MSCs and iPS cells.
Allogenic versus autogenic MSCs
As for cell implantation, there are four different cell types: autogenic (same individual), syngeneic (genetically identical individuals), allogenic (genetically different individuals but same species) and xenogenic (different species) cells. The usage of allogenic cells instead of a patient's own cells offers great advantages in avoiding the risks of morbidity for taking cells from the patient. In particular, the cells of allogenic MSCs are reported to modulate cellular immunity and survive after in vivo implantation [Citation22–25]. In this regard, we have carried out the allogenic implantation experiments. We obtained MSCs from three strains of rats: F344 (RT1lv), ACI (RT1a) and LEW (RT1 l), which had approximately the same weight (nearly 130 g). RT1a, RT11 and RT1lv represent the haplotype in the RT1 region which contains the MHC class I genes. ACI rat is a major antigen mismatch to F344 rat, which means their histocompatibility antigens strongly differ [Citation26]. LEW rat is a minor antigen mismatch to F344 rat, which means histocompatibility antigens partly differ. Bone marrow was collected from each femora, and adherent fibroblastic cells were subcultured in the presence of dexamethasone, β-glycerophosphate and ascorbic acid 2-phosphate [Citation27, Citation28]. All the MSCs grew well and about 10 days later, calcified areas could be detected. The areas were positive for alkaline phosphatase, indicating that the cells can show osteogenic differentiation.
Each of three different MSCs was combined with porous hydroxyapatite (HA) ceramics and subcutaneously implanted into F344 rats [Citation29]. After 8 weeks implantation, the implants were harvested and subjected to micro-computed tomography (CT) analyses followed by assay for alkaline phosphatase activity. As seen in figure , only MSCs from F344 rats showed high ALP activity, when the recipient rats were not treated with immunosuppressant. The CT images showed areas of high (white), medium (gray), and low (black) intensities [Citation30]. We defined the white, gray, and black areas as hydroxyapatite, newly formed bone (arrows), and fibrovascular tissue with fat cells, respectively. Only MSCs from F344 rats showed gray areas (bone formation: arrows) inside the pore regions of HA ceramics. In contrast, the allogenic cells were either rejected or not rejected if the donor rats were treated with the immunosuppressant (+). As seen in the figure , in the presence of immunosuppressant (+), all the MSCs from 3 different donors showed high ALP activity as well as new bone formation [Citation30].
Figure 1 In vivo osteogenic assay of syngeneic/allogenic MSCs. Bone marrow was collected from three strains of rats (F433, ACI and Lew). The bone marrow was cultured primarily and the proliferated MSCs were combined with porous hydroxyapatite (HA) ceramics and implanted subcutaneously into recipient F344 rat. F344 rats were treated with immunosuppressant of FK506 (+) or saline (−). The MSCs/HA composites were extirpated and used for ALP activity assay (upper figures) as well as μCT analyses (lower figures). White areas and gray areas in uCT images indicate the HA ceramics and new bone formation, respectively.
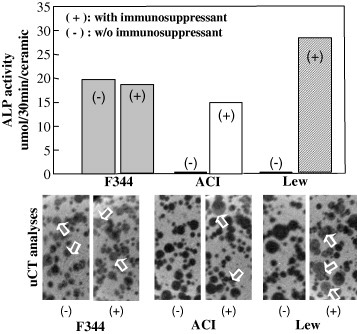
Syngeneic cells can be thought of as autogenic cells when F344 rats were used as donor and recipient. Therefore our model is a straightforward experiment that promises the proliferation/differentiation capability of autogenous MSCs or allogenic cells in the presence of immunosuppressant. In other words, not only major mismatched but also minor mismatched MSCs could not escape immunorejection. However, when the recipient rats were treated with immunosuppressant, the alloresponses were completely circumvented and the implanted cells finally exhibited osteogenic differentiation.
These results indicate that the administration of an immunosuppressant might enable the use of allogenic MSCs for a wide variety of tissue/organ regeneration. It is well known that the immune system protects the body from infection, and when the immune system is suppressed, infections are more likely to occur. Therefore, clinical application of allogenic MSCs with an immunosuppressant might depend on the severity of the disease and is not feasible to use in bone diseases which are usually not lethal for the patients.
Osteogenic differentiation capabilities of MSCs from bone marrow and adipose tissues
In addition to the bone marrow MSCs (BMSCs), adipose tissue has been considered as a source for MSCs. Same as the bone marrow MSCs, the adipose tissue-derived mesenchymal stem cells (AMSCs) have also been shown to differentiate into many lineages, including chondrogenic [Citation31], osteogenic [Citation32–34], myogenic [Citation35] and other types of tissue specific cells [Citation36–39]. The main advantages of adipose tissue include the huge amount of fatty tissue to be available in cosmetic surgery such as liposuction. The advantage is expected to be practical cell source for regenerative medicine. However, it is not well investigated whether AMSCs have a similar potential for osteogenesis, as do MSCs from other types of tissue, especially bone marrow derived mesenchymal stem cells. We obtained bone marrow and adipose tissue from same F344 rats and expand the number of MSCs from both tissues [Citation40].
As seen in figure , when MSCs from adipose and bone marrow tissues were cultured in the presence of dexamethasone and beta-glycerophosphate, BMSCs showed much higher calcium uptake and ALP activity than those of AMSCs [Citation40]. To observe and quantify the in vivo bone-forming capability of both MSCs, we implanted MSCs/HA composites. After implantation, they were examined by micro-CT analysis. There were more massive areas of NB (gray areas) in the BMSCs/HA composite; however, we could not detect bone areas in adipose MSCs/HA composites. Therefore, osteogenic differentiation capability of the bone marrow MSCs (BMSCs) is much superior to that of adipose MSCs (AMSCs) [Citation40].
Figure 2 In vitro/in vivo osteogenic assay of MSCs from bone marrow and adipose tissue. MSCs were cultured in the presence of dexamethasone, beta-glycerophosphate and ascorbic acid (upper figures) for in vitro osteogenic assay. To examine in vivo osteogenic activity of the MSCs, the MSCs/HA composites were implanted for 8 weeks and harvested. The implants were used for μCT analyses (lower figures). White areas and gray areas indicate the HA ceramics and new bone formation, respectively. The MSCs used were bone marrow MSCs (BMSCs) or adipose MSCs (AMSCs).
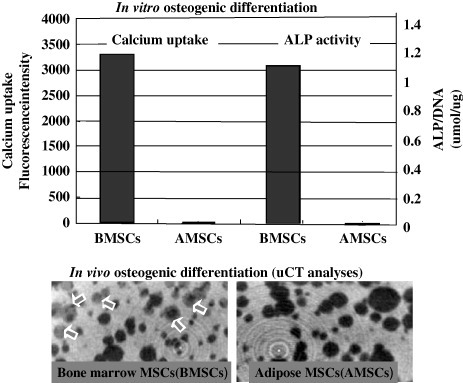
Clinical applications of bone marrow MSCs
As described above, allogenic MSCs could not survive and did not show osteogenic differentiation after their implantation. In addition, a feasibility of the use of bone marrow MSCs for osteogenic cell source is clear, thus we decided to use patient's bone marrow for expansion of MSCs [Citation10]. Since 2001, we have treated patients with osteoarthritis, bone tumors and osteonecrosis by the implantation of the bone marrow MSCs/various ceramics composites [Citation15–17].
To obtain MSCs, we aspirated about 10 ml of the patient's bone marrow by needle. We added 3 ml of the fresh marrow into two 75 cm2 plastic culture flasks and cultured it with a medium containing 15% serum. At each medium change (3 times/week), any non-adherent hematopoietic cells were removed, leaving only adherent cells in the dish. After about 10 days, the semi-confluent adherent cells were collected after trypsinization and further cultured in other flasks [Citation15]. After 4 to 8 days, the shape of most cells was fibroblastic and negative for hematopoietic markers (CD14, 34 and 45) but positive for markers present in mesenchymal cells (CD13, 29 and 90). The MSCs were trypsinized to prepare a cell suspension (5×105 cells ml−1), in which HA ceramics were soaked and further cultured for 2 weeks in a medium supplemented with beta-glycerophosphate, vitamin C, and dexamethasone. To monitor cell proliferation and capacity for differentiation, some of the trypsinized cells were also cultured in a culture plate and in another ceramics. Mineralization (asterisks in figure ) and alkaline phosphatase (ALP) activity of the cells were marked on the plate. For bone tumor treatment, after adequate curettage of the tumors, blocks and granules of cultured bone were carefully gathered to fill the bone defects [Citation15–17].
Figure 3 Proliferation and mineralization of human MSCs. MSCs were cultured in the presence of dexamethasone, beta-glycerophosphate and ascorbic acid. Asterisks show the mineralized areas.
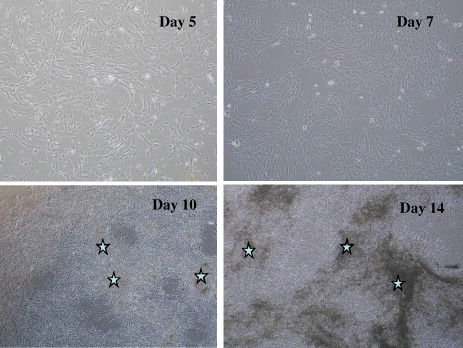
Although bone marrow MSCs are presently considered as one of the most promising stem cells for cellular therapy because of their expansion and differentiation potentials, the cells lose their potentials after several passages. The loss of potentials shows the limitation or impractical treatments in some clinical cases. These situations need more efficient stem cells for clinical applications. The most potential cells having stemness is the ES cells [Citation4, Citation5]; however, their ethical concern and their use in the patients as allogenic implantation have severe problems in clinical applications. In order to circumvent the problems of ES cells, iPS cells might be useful in clinical situations because of reduced ethical issues and possible cell source for autogenous implantation [Citation18–20].
Generation of iPS cells (transduction of plural factors into adult cells)
Several months after the establishment of mouse iPS cells [Citation18], human iPS cells were generated by the same research group [Citation20]. They have transduced four factors of OCT3, SOX2, KLF4 and MYC into human adult cells including skin fibroblasts. Interestingly, at the same time, US researchers reported the human iPS generation by transduction of OCT3, SOX2, NANOG and LIN28 [Citation21]. The efficiency of that process was low—one iPS line could be established from 5 to 10 thousand cells treated. Despite the low efficiency, the process is usable, because it is possible to obtain millions of iPS cells from the mother iPS cell. As the iPS cells could be generated from adult human skin cells, it could be used to generate patient-specific cell lines.
It is noteworthy that human adult MSCs could also be used for iPS generation. As seen in figure , we could generate iPS cells after transduction of transcription factors into adult MSCs. The iPS cells expressed human ES cell-specific surface antigens and transcription factors, such as stage-specific embryonic antigen (SSEA)-3, SSEA-4, OCT3/4 and NANOG. The iPS cells could differentiate into the cells of three germ layers after formation of three-dimensional cell aggregates called embryoid body. Further differentiation was done by the culture of the embryoid body on the dish surface. As seen in figure , we could detect beta-tubulin (a marker of ectoderm), alpha-smooth muscle actin (alpha-SMA, mesoderm), vimentin (mesoderm and parietal endoderm) and SOX17 (endoderm). After in vivo implantation of the iPS cells, we found tumor mass which demonstrated the existence of bone/cartilage tissues (mesoderm), gut-like epithelium (endoderm) and neuroepithelial rosettes (ectoderm). These data imply that the iPS cells have capability to differentiate into various cells of three germ layers.
Figure 4 Characterization of iPS cells from MSCs. Immunocytochemistry of SSEA-3, SSEA-4, OCT3/4 and NANOG for the iPS colony. Embryoid body formation of iPS cells. Differentiation of the embryoid body after attachment on the culture dish. Immunocytochemistry of beta-tubulin, vimentin, alpha-SMA and SOX17.
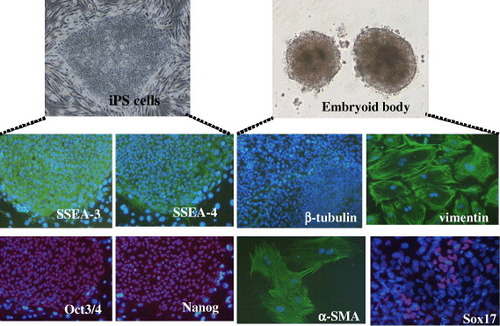
Maintenance of MSCs function (transduction of a single factor into MSCs)
MSCs could differentiate into various tissue-specific cells including osteoblasts/chondrocytes. Therefore, we have utilized the MSCs from patients of osteoarthritis, bone tumor and osteonecrosis for treatments. However, as mentioned above, after several passages of the cultured MSCs, their proliferation and differentiation capabilities decrease drastically. In this regard, iPS cells can be generated from patients and the cells could show more extensive proliferation and differentiation capabilities than those of MSCs. Because of the excellent capabilities of the iPS cells, therapeutical approaches using the iPS cells might be ideal for the patient's treatments. In spite of this advantage of the iPS cells, a big problem for clinical application is that the cells could show tumor (teratoma) formation after in vivo implantation. This problem hindered our use of the iPS cells, but we could use MSCs which have no tumorgenic properties.
Although we could use patient's MSCs for the treatments, the MSCs show the decrease in proliferation/differentiation capabilities, especially after several passages. To solve this problem, we tried to transduce a single factor into the MSCs. Transcription factors of SOX2 and NANOG are essential for pluripotency and self-renewal of embryonic stem cells [Citation41–44]. Stem cells from fetal tissues, which had great growth and plasticity properties, also expressed these transcription factors [Citation45, Citation46]. We therefore introduced either SOX2 or NANOG gene into the MSCs. We found that SOX2-expressing MSCs showed consistent proliferation and osteogenic capability in culture media containing basic fibroblast growth factor (bFGF) compared to control cells (figure , left) [Citation47]. We also found that NANOG-expressing cells, even in the absence of bFGF, had much higher capabilities for expansion and osteogenesis than control cells (figure , right) [Citation47]. These results demonstrate an effective way to maintain proliferation and differentiation potentials, i.e. self-renewal of MSCs. We also confirmed the absence of teratoma formation after in vivo implantation of the SOX2 or NANOG expressing MSCs.
Figure 5 Proliferation and osteogenic activities of SOX2 or NANOG-expressing human MSCs. Retrovirus containing SOX2 (left figures) or NANOG (right figures) gene was transduced into MSCs and then cultured in the presence of 2% fetal bovine serum. SOX2-expressing cells were also cultured in media containing the bFGF protein (10 ng ml−1). The cells were seeded at 1×104 cells per well in a 6-well plate, and the cell number was counted at every passage (4 to 10 passages). The passage number of the cells is indicated. Lower figures show the results of ALP activity measurement (ALP activity/ugDNA).
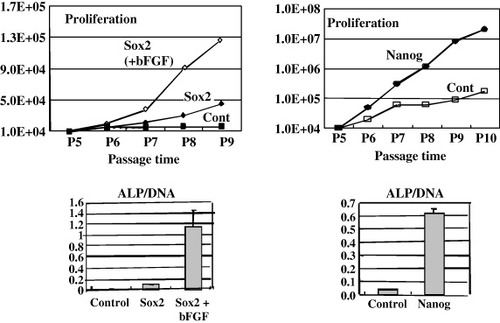
Concluding remarks
Regenerative medicine and tissue engineering have been rapidly developing toward practical use. Various kinds of cells could be used for this purpose and we have employed the MSCs/ceramic composites for regeneration of damaged bone tissue. To advance the regenerative medicine, knowledge of the potentials of MSCs concerning the proliferation and differentiation is essential. Our experiences indicate that bone marrow derived MSCs could be ideal candidates for therapeutical applications; furthermore the potential could be enhanced by transduction of a single transcription factor. These findings indicate the importance of transcription factors expression for maintenance of proliferation/differentiation capabilities in the adult stem cells.
Acknowledgments
This work was partly supported by the Japan Science and Technology Agency (JST) and the New Energy and Industrial Technology Development Organization (NEDO). We thank our colleagues of Tissue Engineering Group, Research Institute for Cell Engineering, National Institute of Advanced Industrial Science and Technology. We also thank orthopedic surgeons at the Department of Orthopedics, Nara Medical University.
References
- BeckerA JMcCullochEATillJ E 1963 Nature 197 452 http://dx.doi.org/10.1038/197452a0
- SiminovitchLMcCullochE ATillJ E 1963 J. Cell. Comput. Physiol. 62 327 http://dx.doi.org/10.1002/jcp.1030620313
- EvansMKaufmanM 1981 Nature 292 154 http://dx.doi.org/10.1038/292154a0
- MartinG 1981 Proc. Natl Acad. Sci. USA 78 7634 http://dx.doi.org/10.1073/pnas.78.12.7634
- BradleyAEvansMKaufmanMRobertsonE 1984 Nature 309 255 http://dx.doi.org/10.1038/309255a0
- ThomsonJ A et al 1998 Science 282 1145 http://dx.doi.org/10.1126/science.282.5391.1145
- FriedensteinA JPetrakovaK VKurolesovaA IFrolovaG D 1968 Transplanation 6 230
- OwenM 1985 Bone and Mineral Research vol 3 PeckW A Oxford Elsevier Science p 1
- CaplanA I 1991 J. Ortho. Res. 9 641 http://dx.doi.org/10.1002/jor.1100090504
- OhgushiHCaplanA I 1999 J. Biomed. Mater. Res. 48 913 http://dx.doi.org/10.1002/(SICI)1097-4636(1999)48:6<913::AID-JBM22>3.0.CO;2-0
- ProckopD J 1997 Science 276 71 http://dx.doi.org/10.1126/science.276.5309.71
- PittengerM F et al 1999 Science 284 143 http://dx.doi.org/10.1126/science.284.5411.143
- ReyesMLundTLenvikTAguiarDKoodieLVerfaillieC M 2001 Blood 98 2615 http://dx.doi.org/10.1182/blood.V98.9.2615
- JiangY et al 2002 Nature 418 41 http://dx.doi.org/10.1038/nature00870
- OhgushiH et al 2005 Biomaterials 26 4654 http://dx.doi.org/10.1016/j.biomaterials.2004.11.055
- MorishitaTHonokiKOhgushiHKotobukiNMatsushimaATakakuraY 2006 Artif. Organs 30 115 http://dx.doi.org/10.1111/j.1525-1594.2006.00190.x
- TadokoroMKanaiRTaketaniTUchioYYamaguchiSOhgushiH 2009 J. Pediatr. 154 924 http://dx.doi.org/10.1016/j.jpeds.2008.12.021
- TakahashiKYamanakaS 2006 Cell 126 663 http://dx.doi.org/10.1016/j.cell.2006.07.024
- OkitaKIchisakaTYamanakaS 2007 Nature 448 313 http://dx.doi.org/10.1038/nature05934
- TakahashiK et al 2007 Cell 131 861 http://dx.doi.org/10.1016/j.cell.2007.11.019
- YuJ et al 2007 Science 318 1917 http://dx.doi.org/10.1126/science.1151526
- ArinzehT L et al 2003 J. Bone Joint Surg. Am. A 85 1927
- BartholomewA et al 2002 Exp. Hematol. 30 42 http://dx.doi.org/10.1016/S0301-472X(01)00769-X
- RasmussonIRingdenOSundbergBLe BlancK 2005 Exp. Cell Res. 305 33 http://dx.doi.org/10.1016/j.yexcr.2004.12.013
- AggarwalSPittengerM F 2005 Blood 105 1815 http://dx.doi.org/10.1182/blood-2004-04-1559
- GillT J IIIKunzH WMisraD NHassettA L 1987 Transplantation 43 773
- ManiatopoulousCSodekJMelcherA H 1988 Cell Tissue Res. 254 317 http://dx.doi.org/10.1007/BF00225804
- OhgushiHDohiYKatsudaTTamaiSTabataSSuwaY 1996 J. Biomed. Mater. Res. 32 333 http://dx.doi.org/10.1002/(SICI)1097-4636(199611)32:3<333::AID-JBM5>3.0.CO;2-T
- AkahaneMOhgushiHYoshikawaTSempukuTTamaiSTabataSDohiY 1999 J. Bone Mineral Res. 14 561 http://dx.doi.org/10.1359/jbmr.1999.14.4.561
- KotobukiNKatsubeYKatouYTadokoroMHiroseMOhgushiH 2008 Cell Transplant. 17 705 http://dx.doi.org/10.3727/096368908786092793
- EricksonG RGimbleJ MFranklinD MRiceH EAwadHGuilakF 2002 Biochem. Biophys. Res. Commun. 290 763 http://dx.doi.org/10.1006/bbrc.2001.6270
- CowanC MShiY YAalamiOChouY FMariCThomasRQuatroNContagC HWuBLongakerM T 2004 Nat. Biotechnol. 22 560 http://dx.doi.org/10.1038/nbt958
- HalvorsenY CWilkisonW OGimbleJ M 2004 Int. J. Obes. Relat. Metab. Disord. 24 S41
- HicokK CLaneyT TZhouY SHalvorsenY DHittD CCooperL FGimbleJ M 2004 Tissue Eng. 10 371 http://dx.doi.org/10.1089/107632704323061735
- MizunoHZukP AZhuMLorenzH PBenhaimPHedrickM H 2002 Plast. Reconstr. Surg. 109 199 http://dx.doi.org/10.1097/00006534-200201000-00030
- SaffordK MHiockK CSaffordS DHalvorsenY DWilkinsonW OGimbleJ MRiceH E 2002 Biochem. Biophys. Res. Commun. 294 371 http://dx.doi.org/10.1016/S0006-291X(02)00469-2
- RodriguezA M et al 2005 J. Exp. Med. 201 1397 http://dx.doi.org/10.1084/jem.20042224
- Planat-BernardVMenardCAndreMPuceatMPerezAGarcia-VeedugoJ MPenicaudLCasteillaL 2004 Circ. Res. 94 223 http://dx.doi.org/10.1161/01.RES.0000109792.43271.47
- SeoM JSuhS YBaeY CJungJ S 2005 Biochem. Biophys. Res. Commun. 328 258 http://dx.doi.org/10.1016/j.bbrc.2004.12.158
- HayashiOKatsubeYHiroseMOhgushiHItoH 2008 Calcif. Tissue Int. 82 238 http://dx.doi.org/10.1007/s00223-008-9112-y
- BoyerL A et al 2005 Cell 122 947 http://dx.doi.org/10.1016/j.cell.2005.08.020
- LohY H et al 2006 Nat. Genet. 38 431 http://dx.doi.org/10.1038/ng1760
- WangJRaoSChuJShenXLevasseurD NTheunissenT WOrkinS H 2006 Nature 444 364 http://dx.doi.org/10.1038/nature05284
- EpiskopouV 2005 Trends Neurosci. 28 219 http://dx.doi.org/10.1016/j.tins.2005.03.003
- GuillotP VGotherstromCChanJKurataHFiskN M 2007 Stem Cells 25 646 http://dx.doi.org/10.1634/stemcells.2006-0208
- IlancheranSMichalskaAPehGWallaceE MPeraMManuelpillaiU 2007 Biol. Reprod. 77 577 http://dx.doi.org/10.1095/biolreprod.106.055244
- GoM JTakenakaCOhgushiH 2008 Exp. Cell Res. 314 1147 http://dx.doi.org/10.1016/j.yexcr.2007.11.021