Abstract
Nanocrystalline SrAl2Si2O8 :Eu2+ phosphor layers were coated on nonaggregated, monodisperse and spherical SiO2 particles using a hydrothermal homogeneous precipitation. After annealing at 1100 °C, core-shell SiO2@SrAl2Si2O8 :Eu2+ particles were obtained. They were characterized with x-ray diffraction (XRD), scanning electron microscopy, transmission electron microscopy and photoluminescence techniques. XRD analysis confirmed the formation of SiO2 @SrAl2Si2O8 :Eu2+ particles; it indicated that the SrAl2Si2O8 :Eu2+ shells on SiO2 particles consisted of hexagonal crystallites. The core-shell phosphors obtained are well-dispersed submicron spherical particles with a narrow size distribution. The thickness of the coated layer is approximately 20–40 nm. Under ultraviolet excitation (361 nm), the particles emit blue light at about 440 nm due to the Eu2+ ions in their shells.
Introduction
Recently, much effort has been devoted to the design and controlled fabrication of nanostructured materials with functional properties. Among them, core-shell particles have attracted much interest because of their unique properties and outstanding performance. Different from single-component materials, the synthesis of core-shell particles has opened new directions for material research [Citation1–3]. Core-shell composites can be monodisperse spherical particles with good optical performance for applications. There are numerous methods of preparing core-shell-structured materials including layer-by-layer self-assembly [Citation1, Citation4], coprecipitation [Citation5], sol–gel process [Citation6, Citation7], surface reaction [Citation8] and metalorganic vapor phase epitaxy (MOCVD) [Citation9]. These wet chemical techniques can offer the possibilities of controlling homogeneity, phase purity, size distribution, surface area and microstructure uniformity of the powder.
Novel light emitting diodes (LEDs) have resulted in new requirements for the phosphor materials [Citation10]. The desired properties of the phosphor particles include a perfect spherical shape, narrow size distribution (0.5–2 μm) and absence of agglomeration [Citation11]. Spherical morphology allows achieving high brightness, high spatial resolution, high packing density and low light scattering [Citation12, Citation13].
The size of silica particles can be controlled from nanometers to micrometers using the Stöber process. These particles are frequently used in core-shell materials either as cores or as shells [Citation8, Citation14, Citation15]. If the silica spheres could be coated with phosphor layers then a core-shell phosphor material with spherical morphology would be obtained and the size of the phosphor particles could be controlled by the silica cores. Furthermore, because silica is cheaper than most phosphor materials, which often employ expensive rare-earth elements as activators and/or host components, core-shell phosphor materials should be cheaper than pure phosphors.
SrAl2 Si2 O8 :Eu2+ is a potential blue phosphor suitable for LEDs and other luminescent devices. It is conventionally prepared in a solid-state reaction at high temperature. However, it is very difficult to control the particle size and crystal structure under these conditions [Citation10, Citation16]. Hydrothermal homogeneous precipitation is one of the ‘soft’ chemical methods, which was successfully employed to prepare phosphor particles with suitable morphology. This method can work at lower temperatures compared with the solid-state reaction.
In this work, we obtained monodisperse and spherical core-shell SiO2 @SrAl2 Si2 O8 :Eu2+ phosphors by hydrothermal homogeneous precipitation. The thus produced particles have advantages of low processing temperature, high monodispersity and purity of the product. We characterized the structure, morphology and photoluminescent properties of these core-shell phosphors.
Experimental section
The starting materials were tetraethoxysilane (TEOS, 99%, Tianjin Fuchen Chemical Reagent Factory), Al(NO3)3 ⋅9H2 O (analytical reagent=A.R., Shanghai Zhenxin Reagent Factory), SrCO3 (A.R., Tianjin Fuchen Chemical Reagent Factory), NH4 OH (25 wt%, A.R., Guangzhou Chemical Reagent Factory), urea (A.R., Guangzhou Chemical Reagent Factory), HNO3 (A.R., Guangzhou Chemical Reagent Factory), ethanol (A.R., Tianjin Youngda Chemical Reagent Company Ltd) and Eu2 O3 (99.99%).
Synthesis of silica cores
Monodispersed silica spheres of 560 nm size were synthesized by hydrolysis of TEOS in an alcohol medium, in the presence of water and ammonia, with the Stöber [Citation17] process. In a typical experiment, 17.2 ml of TEOS, 36 ml of deionized H2 O and 196 ml of NH3⋅H2O were added into 152 ml of absolute ethanol and stirred at room temperature for 4 h, which produced a white silica colloidal suspension. The silica particles were centrifugally separated from the suspension and washed with ethanol three times. They were then dried at 60 °C for 10 h resulting in silica spheres.
Synthesis of core-shell structured SiO2@SrAl2Si2O8:Eu2+ phosphors
The core-shell SiO2 @SrAl2 Si2 O8 :Eu2+ particles were prepared with a hydrothermal homogeneous precipitation method which is schematically illustrated in figure . Firstly, Eu2 O3 and SrCO3 powders were dissolved in a minimum amount of nitric acid. They were then dried and dissolved in deionized water to form strontium nitrate and europium nitrate solution. Secondly, certain amounts of SiO2 particles were ultrasonically dispersed in the stock solution containing a certain amount of TEOS, 0.1mol l-1 Al(NO3)3, 0.049 mol l-1 Sr(NO3)2 and 0.5 mol l-1(NH2)2CO. The dispersions were then sealed in a 50 ml Teflon-lined stainless autoclave and maintained at 160 °C for 24 h. After the autoclave was naturally cooled to room temperature, the precipitate was washed with distilled water three times and dried at 160 ° C in air ambient. Then, the samples were heated to 1100 °C at a heating rate of 5 °C min-1 and held at this temperature for 2 h in an activated carbon reducing atmosphere.
Characterization
X-ray diffraction (XRD) analysis was carried out using a Rigaku Model D/max-II B x-ray diffractometer with Cu Kα1 (λ=0.154 05 nm) radiation at a 0.02° (2θ) min-1 scanning steps. The sample morphology was studied with scanning electron microscopy (SEM, Philips XL-30) and transmission electron microscopy (TEM, Philips TECNAI 10). The particle size and its distribution were evaluated with a nano particle size analyzer (Malvern Zetasizer Nano ZS). Photoluminescence was measured with a HITACHI F-4500 fluorescence spectrophotometer using a Xe lamp as the excitation source.
Results and discussion
XRD analysis
Figure shows the XRD patterns of bare SiO2 particles, SiO2@SrAl2Si2O8:Eu2+particles and SrAl2Si2O8:Eu2+ powders, as well as the standard reference data for SrAl2Si2O8 (JCPDS No. 35-0073). For bare SiO2 particles, no sharp peaks are observed as shown in figure (a) and only a broad feature is seen at 2θ=22.0°, which is characteristic of amorphous SiO2 (JCPDS No. 29-0085). For the SiO2@SrAl2Si2 O8:Eu2+ particles (figure (b)), all the diffraction peaks of crystalline SrAl2Si2O8 are present beside the amorphous SiO2 band, suggesting that the coatings of SrAl2Si2O8:Eu2+ have well crystallized on the surface of amorphous silica particles. This pattern coincides well with that of pure SrAl2Si2O8:Eu2+ powder (figure (c)) and the standard data for SrAl2Si2O8.
Morphology of the core-shell particles
Figure (a) shows an SEM image of bare SiO2 particles. The particles are well separated and spherical with an average size of 560 nm. On the contrary, the SrAl2Si2O8:Eu2+ powder samples contain aggregates sized between 10 and 100 μm (figure (b)). After being coated by one layer of SrAl2Si2O8:Eu2+, the resulting SiO2@SrAl2Si2O8:Eu2+ particles maintain the morphology of the silica particles. The composite particles are still spherical and non-aggregated, they have rough surfaces and are slightly larger than the bare SiO2 particles owing to the additional layer of SrAl2Si2O8:Eu2+. After annealing at 1200 °C, the silica spheres have partly collapsed and agglomerated (figure (d)).
Figure 3 SEM images of the (a) bare SiO2 particles, (b) SrAl2Si2 O8:Eu2+ powders, (c) core-shell SiO2@SrAl2Si2 O8:Eu2+ particles annealed at 1100 °C, and (d) core-shell SiO2@SrAl2Si2 O8:Eu2+ particles annealed at 1200 °C.
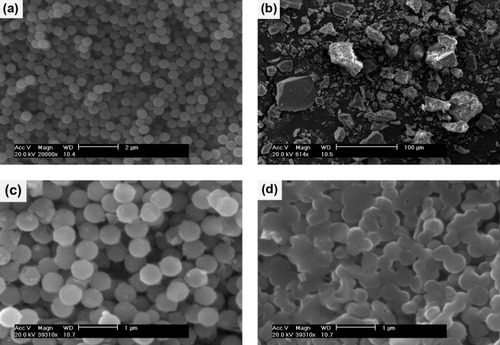
Figure presents TEM images of silica particles before and after coating with SrAl2Si2O8:Eu2+. The surface of the bare SiO2 particles is very smooth (figure (a)), and the core-shell structure of the SiO2@SrAl2Si2 O8:Eu2+ particles can be clearly seen because of the different electron absorption in the core and the shell (figure (b)). The cores are black spheres with an average diameter of 560 nm, which is similar to the bare SiO2 particles in figure (a), whereas the shells have a gray appearance and an average thickness of 20–40 nm.
Figure 4 TEM images of the (a) bare SiO2 particles and (b) core-shell SiO2@SrAl2Si2O8:Eu2+ particles annealed at 1100 °C.
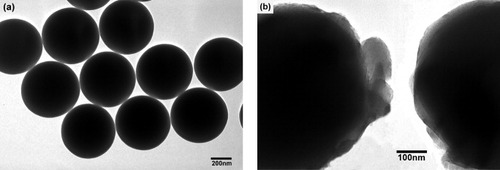
The average particle sizes and standard deviations of the bare SiO2 and core-shell SiO2@SrAl2 Si2O8:Eu2+ particles are summarized in table 1. After coating one layer of SrAl2Si2O8:Eu2+ on SiO2 particles, the average particle size increased from 560 to 586 nm with a narrow particle size distribution (figure ). Compared with the bare SiO2 particles, the standard deviation of the coated samples increased from 5 to 6%. However, the SiO2@SrAl2Si2 O8:Eu2+ particles are still monodisperse and nonaggregated.
Luminescence properties of SiO2@SrAl2Si2O8:Eu2+ particles
Figure presents room-temperature photoluminescence excitation and emission spectra of the SiO2@SrAl2 Si2O8:Eu2+ particles and SrAl2Si2O8:Eu2+ powders. Both the excitation and emission bands can be assigned to the well-known Eu2+ transitions between the ground state 8S7/2(4f7) and the excited states of the 4f65d1 configuration. The excitation spectra consist of a broad feature between 250 and 400 nm with the maximum at 360 nm (figure , spectra (a) and (b)), which is attributed to the charge-transfer band between Eu2+ and O2−. The UV-excitation of the SiO2@SrAl2Si2O8:Eu2+ particles at room temperature yielded bright blue luminescence peaking at 442 nm (figure , spectrum (c)). The spectrum is broad (half-width ∼100 nm) but symmetric. It indicates one emission mechanism, which can be assigned to Eu2+. The luminescence of SiO2@SrAl2Si2O8:Eu2+ particles is slightly weaker than that of the SrAl2Si2O8:Eu2+ powders, which were also annealed at 1100 °C. It is known that the quantum yield of doped nanoparticles is usually lower than that of the corresponding bulk material because of numerous defects on the nanocrystal surface [Citation18]. The coating of the core-shell particles is so thin (nanometer scale) that it contains numerous surface defects which enhance the non-radiative relaxation.
Figure 6 Photoluminescence excitation and emission spectra of SiO2@SrAl2Si2O8:Eu2+ particles (a) and (c), and SrAl2Si2O8:Eu2+ powders (b) and (d), respectively.
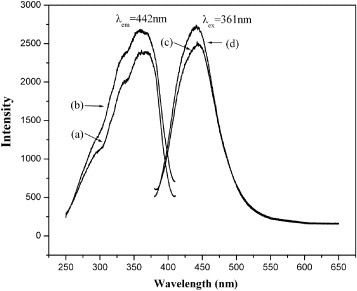
The sharp Eu2+ peaks do not appear in figure , which means that the excited state of the 4f6 5d configuration is lower in energy than the lowest excited state of the 4f7 configuration in the SrAl2Si2O8 matrix. Furthermore, the characteristic emission peak of Eu3+is not observed. This may be explained by that Eu3+ was mostly reduced to Eu2+.
Conclusions
In this work, a blue phosphorescent material, core-shell SiO2@SrAl2Si2O8:Eu2+ particles, was prepared with a uniform size distribution using a simple hydrothermal homogeneous precipitation process followed by annealing at 1100 °C. The obtained core-shell structured SiO2@SrAl2Si2O8:Eu2+ particles maintain spherical morphology and sub-micrometer size. Upon UV excitation, they show luminescence typical of SrAl2Si2O8:Eu2+. The photoluminescence excitation and emission spectra reveal that Eu2+ did incorporate into SrAl2Si2O8 and maintain its luminescent properties.
Acknowledgments
This work was financially supported by the National Natural Science Foundations of China (Nos. 20671042 and 50872045) and the Natural Science Foundation of Guangdong Province (Nos. 05200555 and 7005918).
References
- CarusoF 2001 Adv. Mater. 13 11 http://dx.doi.org/10.1002/1521-4095(200101)13:1<11::AID-ADMA11>3.0.CO;2-N
- LuYYinYLiZ YXiaY 2002 Nano Lett. 2 785 http://dx.doi.org/10.1021/nl025598i
- SchartlW 2000 Adv. Mater. 12 1899 http://dx.doi.org/10.1002/1521-4095(200012)12:24<1899::AID-ADMA1899>3.0.CO;2-T
- SuryanarayananVNairA STomR TPradeepT 2004 J. Mater. Chem. 14 2661 http://dx.doi.org/10.1039/b404408h
- LiuGHongG 2005 J. Solid State Chem. 178 1647 http://dx.doi.org/10.1016/j.jssc.2005.03.010
- SchuetzPCarusoF 2002 Chem. Mater. 14 4509 http://dx.doi.org/10.1021/cm0212257
- LinCKongDLiuXWangHYuMLinJ 2007 Inorg. Chem. 46 2674 http://dx.doi.org/10.1021/ic062318j
- Salgueirino-MaceiraVSpasovaMFarleM 2005 Adv. Funct. Mater. 15 1036 http://dx.doi.org/10.1002/adfm.200400469
- ChangK WWuJ J 2005 Adv. Mater. 17 241 http://dx.doi.org/10.1002/adma.200400708
- ShiYLiuZ SWangJ GWangL XJingX P 2009 J. Lumin. 129 50 http://dx.doi.org/10.1016/j.jlumin.2008.07.015
- WangHYuMLinCLiuXLinJ 2007 J. Phys. Chem. C 111 11223 http://dx.doi.org/10.1021/jp072541h
- JingXIrelandTGibbonsCBarberD JSilverJVechtAFernGTrowgaPMortonD C 1999 J. Electrochem. Soc. 146 4656 http://dx.doi.org/10.1149/1.1392689
- JiangY DWangZ LZhangFParisH GSummersC J 1998 J. Mater. Res. 13 2950 http://dx.doi.org/10.1557/JMR.1998.0403
- LiuSHanM 2005 Adv. Funct. Mater. 15 961 http://dx.doi.org/10.1002/adfm.200400427
- HardikarV VMatijevićE 2000 J. Colloid Interface Sci. 221 133 http://dx.doi.org/10.1006/jcis.1999.6579
- FrédéricCAlainGPierreBDanielleGThierryL MPhilippeDStéphaneJ 2008 J. Solid State Chem. 181 1456 http://dx.doi.org/10.1016/j.jssc.2008.03.011
- StöberWFinkABohnE 1968 J. Colloid Interface Sci. 26 62 http://dx.doi.org/10.1016/0021-9797(68)90272-5
- FangY PXuA WDongW F 2005 Small 1 967 http://dx.doi.org/10.1002/smll.200500086