Abstract
The effect of the hydrophobic group content in gelatin on the bonding strength of novel tissue–penetrating tissue adhesives was evaluated. The hydrophobic groups introduced into gelatin were the saturated hexanoyl, palmitoyl, and stearoyl groups, and the unsaturated oleoyl group. A collagen casing was employed as an adherend to model soft tissue for the in vitro determination of bonding strength of tissue adhesives composed of various hydrophobically modified gelatins and disuccinimidyl tartrate. The adhesive composed of stearoyl-modified gelatin (7.4% stearoyl; 10Ste) and disuccinimidyl tartrate showed the highest bonding strength. The bonding strength of the adhesives decreased as the degree of substitution of the hydrophobic groups increased. Cell culture experiments demonstrated that fluorescein isothiocyanate-labeled 10Ste was integrated onto the surface of smooth muscle cells and showed no cytotoxicity. These results suggest that 10Ste interacted with the hydrophobic domains of collagen casings, such as hydrophobic amino acid residues and cell membranes. Therefore, 10Ste–disuccinimidyl tartrate is a promising adhesive for use in aortic dissection.
1. Introduction
Adhesives for soft tissues have been used since 1960 for blood vessels, dural substitutes, soft tissue extremity transection, nephrotomy closure and as a substitute for sutures [Citation1]. An ideal tissue adhesive should bond adequately to tissue [Citation2], be nontoxic and eventually degrade in vivo. Three main types of tissue adhesives have been used: cyanoacrylate monomer [Citation3], biopolymer-aldehyde [Citation4–Citation6] and fibrin adhesives [Citation7]. Cyanoacrylate monomer adhesive rapidly cures through anionic polymerization in the presence of a weak base. This adhesive spreads rapidly on wound surfaces and achieves a strong bond to tissue; however, the resulting polymer is hard compared with soft tissue, and inflammation is induced by the formaldehyde generated by the hydrolysis of the cyanoacrylate polymer. Gelatin-resorcinol-formaldehyde (GRF) glue is a typical biopolymer-aldehyde adhesive. It exhibits excellent bonding strength; however, toxicity and inflammation caused by the residual formaldehyde have been reported [Citation4–Citation6]. Fibrin adhesive is prepared by mixing fibrinogen with thrombin, and shows excellent biocompatibility. However, the bonding strength is lower for fibrin than other types of tissue adhesives. Therefore, the current tissue adhesives have problems either with bonding strength or biocompatibility.
Numerous novel crosslinkers such as N-hydroxysuccinimide-activated poly(l-glutamic acid) [Citation8], polyethylene glycol [Citation9] and organic acid derivatives with active ester groups [Citation10–Citation14] have been developed for tissue adhesives. Tissue adhesives composed of a biopolymer and these crosslinking reagents exhibit excellent adhesive properties compared with fibrin adhesive. Adhesives composed of polyamine [Citation15] and polymeric micelles, dextran derivatives bearing aldehyde groups [Citation16], and multifunctionalized chondroitin sulfate with methacrylate and aldehyde groups [Citation17] have also been reported. They have some advantages compared with clinically used adhesives, although they were not developed as tissue-penetrating adhesives.
Generally, the bonding mechanisms of tissue adhesive can be controlled by the crosslinking density, tissue penetration and bulk (gel) strength. Most studies have focused on the control of the crosslinking density and the bulk (gel) strength. In addition, the modification of polymers with hydrophobic groups, such as cholesterol and oleoyl groups, effectively enhances the physical crosslinking of liposomes or cells by the anchor effect [Citation18–Citation21].
In our previous study, we reported that the adhesive properties of adhesive composed of disuccinimidyl tartrate (DST) and gelatin modified with cholesteryl groups (ChG-DST) was improved by tissue penetration [Citation22, Citation23]. The substitution degree of gelatin amino groups by cholesteryl groups was 10%. Cholesteryl groups were employed as a typical hydrophobic group which anchors into lipid bilayer, i.e., cell membrane and liposome. Based on this background, we assumed that the penetration ability of tissue would be further increased with increasing flexibility of the introduced hydrophobic group. Therefore, we have focused on the development of a novel tissue-penetrating tissue adhesive. We have synthesized hydrophobically modified gelatins (HMGs) by modifying the primary amino groups of gelatin with various hydrophobic groups, including the saturated hexanoyl (Hx; C6), palmitoyl (Pam; C16) and stearoyl (Ste, C18) groups, and the unsaturated oleoyl (Ole; C18) group. Gelatin is obtained by the thermal denaturation or the physical and chemical degradation of collagen; therefore, it can be biodegraded by collagenase during wound healing [Citation24]. We have prepared various kinds of tissue adhesives by combining the HMG with a DST crosslinker, and evaluated their bonding strength against a collagen casing as a model of soft tissue. Additionally, we exposed HMG to smooth muscle cells (SMCs), which are found in blood vessels, and observed the integration behavior to examine the penetration of adhesives into the cell membrane.
2. Materials and methods
2.1. Materials
Gelatin (type A from porcine, MW = 20000) was kindly donated by Nitta Gelatin Inc. (Osaka, Japan). Triethylamine (TEA), dehydrated dimethylsulfoxide (DMSO), ethanol, 2,4,6-trinitrobenzensulfonic acid, HCl, tartaric acid, tetrahydrofuran (THF), fluorescein isothiocyanate isomer-I (FITC), N-hydroxysuccinimide and 10% formalin neutral buffer solution were purchased from Wako Pure Chemical Industries, Ltd (Osaka, Japan) and used without further purification. Hexanoyl chloride, palmitoyl chloride, stearoyl chloride and oleoyl chloride were purchased from Aldrich Chemical Co. (Missouri, USA). Dicyclohexylcarbodiimide was purchased from Kokusan Chemical Co., Ltd (Tokyo, Japan). Human aortic smooth muscle cells (SMCs), SmGM®-2 Bulletkit® and ReagentPackTM were purchased from Lonza (Basel, Switzerland). 4′,6-Diamidino-2-phenylindole (DAPI) and Cell Counting Kit-8 were purchased from Dojindo Laboratories (Kumamoto, Japan).
2.2. Synthesis and characterization of HMG
HMG was synthesized by the nucleophilic substitution reaction of the fatty acid ester with the primary amine of gelatin. A mixture of gelatin (60 g) and TEA (6 ml) in DMSO (594 ml) was stirred at room temperature. Either 10 or 50% of the gelatin amino groups were substituted with a hydrophobic group, by adding a prescribed quantity of the fatty acid to the gelatin solution. The mixture was stirred under N2 atmosphere at room temperature overnight. Three equal volumes of cold ethanol were added, and the HMG precipitate was washed with cold ethanol (3 × 500 ml). The HMG was dried and its composition was confirmed by Fourier-transform infrared absorption (FTIR-8400S, Shimadzu, Kyoto, Japan) via the characteristic hydrocarbon bands at 2926 cm−1 (CH2, asymmetric stretch) and 2853 cm−1 (CH2, symmetric stretch). The removal of the remaining unreacted fatty acid was confirmed by proton nuclear magnetic resonance (1H-NMR; AL300, JEOL, Tokyo, Japan) of the supernatant ethanol.
The degree of substitution was estimated by the spectrophotometric 2,4,6-trinitrobenzensulfonic acid (TNBS) method [Citation25]. A mixture of 0.05% HMG (0.1 ml), 4% NaHCO3 (0.1 ml) and 0.1% TNBS (0.1 ml) was placed in a 48-well plate and then incubated at 37 °C for 2 h. The reaction was halted by the addition of 1 N HCl (0.1 ml). The absorbance was measured at 340 nm using a microplate reader (GENios A-5082, Tecan Japan, Kanagawa, Japan).
2.3. Preparation and characterization of DST
DST was prepared using a previously reported method [Citation13]. Tartaric acid (0.033 mol) was dissolved in THF, and then N-hydroxysuccinimide (0.07 mol) and dicyclohexylcarbodiimide (0.083 mol) were added. The resulting mixture was stirred for 15 min at room temperature and then filtered. The filtrate was concentrated by removing THF by rotary evaporation under reduced pressure. The final mixture was recrystallized twice using ethanol to yield pure DST. The DST was characterized by 1H-NMR: 1H-NMR (DMSO-d6) δ = 2.8 (s, 8H, succinimidyl ester CH2 × 4), 3.1–3.3 (m, 2H, CH2), 4.8–4.9 (m, 1H, CH), 6.73 (d, 1H, OH).
2.4. Measurement of bonding strength
The bonding strength was measured by a previously reported method [Citation22]. Figure shows the collagen casing fixed on a plastic plate surface (Aron Alpha, Toagosei, Japan) that was used to determine the bonding strength. The test specimen was first partially masked with a silicone rubber mold (10 × 10 × 0.5 mm). A dispersion of the adhesives and the DST powder in the HMG solution was applied to the top of the collagen casing. A second specimen was then placed on the first test specimen. A 50 g weight was applied to the bonded collagen casings for various times at 37 °C, and the bonding strength was measured at 25 °C using a tensile machine (TA-XT2i, Stable Microsystems, UK). Three samples were tested to determine the bonding strength of each adhesive (n = 3).
2.5. Direct observation of HMG integration onto cell membrane
FITC-labeled gelatin with or without hydrophobic groups was prepared by a previously reported method [Citation23]. The reaction occurred between the amino groups of gelatin or HMG and the FITC isothiocyanate group. The fluorescence of FITC was maintained by performing the reaction in the dark. Gelatin or HMG (1.0 g) was dissolved in DMSO (3.4 ml), and then TEA (0.1 ml) and FITC (10% with respect to the gelatin amino groups) in DMSO (1.5 ml) were added. Light was excluded, and the reaction mixture was kept in N2 atmosphere at room temperature overnight. FITC-labeled gelatin or FITC-labeled HMG was precipitated in cold ethanol and washed three times with ethanol. The unreacted FITC was detected by fluorescence spectroscopy. The FITC-labeled gelatin or FITC-labeled HMG was dried at room temperature and fluorescence emission analysis was used to confirm the presence of the label.
SMCs were precultured at 37 °C and in 5% CO2 for 3 days in SM-basal medium supplemented with human epidermal growth factor, human fibroblast growth factor, gentamicin/amphotericin-B (GA-1000) and insulin, without fetal bovine serum (FBS) in 48-well plates (75 000 cells cm−2). SMCs were used at passage 6 and were washed three times with phosphate-buffered saline (PBS) and treated with 0.01 g l−1 FITC-labeled HMG in PBS for 5 min at 37 °C. The concentration of HMG was fixed at a lower value than the critical aggregation concentration. The anchor effects of HMG were observed by washing the cells three times with PBS and then fixing them in 10% formalin neutral buffer solution for 10 min at 4 °C. The cells were washed three times with PBS and the nuclei were labeled with DAPI. The cells were then soaked in PBS and observed under a fluorescence microscope (IX71, Olympus Corporation, Japan).
2.6. Effect of HMG treatment on cell viability
SMCs were seeded in 48-well plates (75 000 cells cm−2) and precultured in medium without FBS for 3 days. SMCs were used at passage 6 and washed three times with PBS and treated with 0.01 g l−1 gelatin, HMG and 0.1% TritonX-100 for 5 min at 37 °C. After the cells were washed three times with PBS, the number of cells was counted according to the protocol for Cell Counting Kit-8. The data were normalized and treated as the cell viability. The samples were tested three times.
2.7. Statistical analysis
Statistical analysis was carried out using Microsoft Excel's statistical function for Student's t-test. A difference was considered significant when p < 0.05. The data are presented as mean ± standard deviation (SD).
3. Results
3.1. Preparation of HMG
HMG was synthesized by introducing various hydrophobic groups to gelatin through the nucleophilic substitution reaction of the fatty acid ester with the primary amines of gelatin (figure ). The hydrophobic groups were hexanoyl (C6, Hx), palmitoyl (C16, Pam), stearoyl (C18, Ste) and oleoyl (C18:1, Ole). The corresponding HMGs are denoted as 10Hx, 10Pam, 10Ste, 10Ole, 50Hx, 50Pam, 50Ste and 50Ole, and the degree of substitution was measured as 7.1, 7.8, 7.4, 7.2, 42.4, 38.9, 41.2 and 44.2%, respectively. The characteristic signal of the hydrophobic carbon at 2926 cm−1 (CH2, asymmetric stretch) and 2853 cm−1 (CH2, symmetric stretch) was confirmed by FTIR spectroscopy. These results indicated that hydrophobic groups were introduced into the gelatin molecule. Figure shows that HMG can be crosslinked with DST by the reaction between the residual amino groups of HMG and the active ester groups of DST. Hydrogel formation was confirmed by mixing the HMGs with DST at a 1:1 ratio of the succinimidyl groups/amino groups, indicating that HMGs can be used as a component of tissue adhesives.
3.2. Measurement of bonding strength
The bonding strength of the tissue adhesives, which contained the original gelatin or HMG with various amounts of the hydrophobic groups, was measured on a shear tensile test system (figure ). Collagen casing was used as an adherend to model soft tissue. The concentration of the original gelatin or HMG solution and the ratio of the succinimidyl groups/amino groups were fixed at 70% w/v and 1:1, respectively. The bonding time was 10 min. Figure (a) shows that the highest bonding strength was observed when 10Ste–DST was applied to the collagen casing (p < 0.05). No significant difference in bonding strength was observed for the original gelatin, 10Hx, 10Pam and 10Ole. Similar experiments were performed using HMG with high hydrophobic group content. The bonding strength of the adhesives with high hydrophobic group content (50Hx, 50Pam, 50Ste and 50Ole) was significantly lower than that of adhesives with a low hydrophobic group content (10Hx, 10Pam, 10Ste and 10Ole). Furthermore, the effect of 10Ste or 10Ole content on the bonding strength with the collagen casing was analyzed. Various amounts of 10Ste or 10Ole were mixed with gelatin, and the bonding strength was evaluated to elucidate the effect of these HMGs on the bonding strength. Figure (b) shows that the bonding strength increased with both the 10Ste and the 10Ole content in the gelatin solution. Therefore, HMG with low hydrophobic group content effectively enhanced the bonding strength with the collagen casing.
Figure 3 Effect of hydrophobic groups on bonding strength against collagen casing. (a) Effect of hydrophobic groups on the bonding strength of HMG with various substitution ratios. White bar shows gelatin with DST. (b) Effect of 10Ste or 10Ole content on the bonding strength of tissue adhesives. The concentration of HMG and the succinimidyl group/amino group ratio was fixed at 70% w/v, and 1:1, respectively. Data are shown as the mean ± SD of three samples (∗p < 0.05).
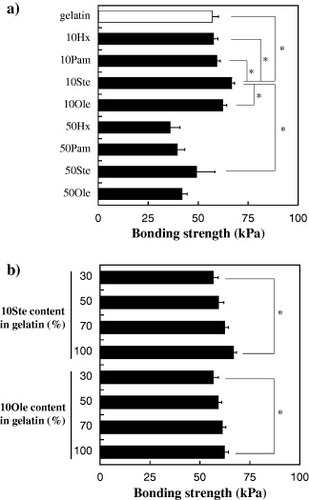
3.3. Direct observation of HMG integration onto cell membranes
SMCs are abundant in blood vessels; therefore the effect of the hydrophobic groups in HMGs–DST adhesives on cells in blood vessels was examined by exposing SMCs to HMGs. Their integration with the SMC cell membranes was observed by using FITC-labeled gelatin or HMG. This experiment was performed using a serum-free medium to observe the interaction between the FITC-labeled HMG and SMCs directly [Citation20]. SMCs were precultured in the serum-free medium for 3 days at 37 °C. The SMCs were then incubated with FITC-labeled gelatin or FITC-labeled HMG for 5 min at 37 °C. The cells were washed three times with PBS, and the FITC-labeled gelatin or HMG was directly observed under a fluorescence microscope. Figure shows the fluorescence images after the cells were exposed to FITC-labeled gelatin or HMG. The FITC-labeled 10Ste and 10Ole were clearly observed on the surface of the SMCs (figures (d) and (e)), indicating that hydrophobic groups were anchored to the surface of the cell membrane. However, only a small amount of FITC-labeled gelatin was observed on the surface of SMCs (figure (a)). FITC-labeled 10Hx and 10Pam also showed no significant FITC fluorescence (figures (b) and (c)) meaning that FITC-labeled 10Hx and 10Pam was not anchored into the cell membrane.
Figure 4 Fluorescence microscopy images of SMCs treated with FITC-labeled (a) gelatin, (b) 10Hx, (c) 10Pam, (d) 10Ste and (e) 10Ole. Blue: nuclei; green: FITC-labeled gelatin or HMG. Scale bar represents 100 μm.
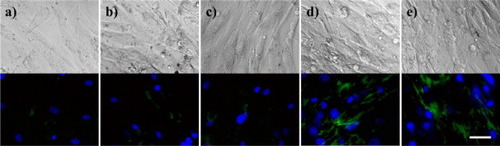
The effect of HMGs on cell viability was investigated by determining the number of cells using water-soluble tetrazolium salt (WST-8) as an emission substrate. The SMCs were precultured in medium without serum and separately treated with 0.01 g l−1 of gelatin, 10Ste and 10Ole for 5 min at 37 °C. The control was 0.1% TritonX-100 because it is a typical surfactant that dissolves cell membranes. It has been reported that hydrophobic groups similar to the oleoyl group rapidly anchor to the cell membrane [Citation18, Citation20]. No significant difference was observed for the SMCs treated with gelatin, 10Ste and 10Ole compared with TritonX-100 (figure ), indicating that little cell injury was induced by hydrophobic group of 10Ste and 10Ole.
4. Discussion
We have developed novel tissue-penetrating tissue adhesives composed of HMGs and DST and evaluated their bonding properties against collagen casing. The bonding strength measurements (figure (a)) showed that 10Ste had the highest bonding strength compared with gelatin and the other HMGs (10Hx and 10Pam). The bonding strength increased with the carbon chain length in HMG containing saturated hydrocarbons. These results show that the Ste group interacted with the hydrophobic domains of the collagen casing, such as hydrophobic amino acid residues. Although 10Ste and 10Ole had the same chain length, the bonding strength of the 10Ste–DST adhesive was significantly higher than that of 10Ole–DST. This result can be explained by the lower thermal denaturation temperature (Td) of 10Ole (31.0 °C) than 10Ste (36.3 °C), and therefore the lower bulk strength of 10Ole. In contrast, the bonding strength of HMGs with high hydrophobic group content (50Hx, 50Pam, 50Ste and 50Ole) was lower than that of HMGs with low hydrophobic group content (10Hx, 10Pam, 10Ste and 10Ole). This was caused by the intermolecular and intramolecular self-assembly and aggregation of hydrophobic groups at a high hydrophobic group content. The high bonding strength values of 10Ste–DST and 10Ole–DST were obtained at 100% content (figure (b)). On the other hand, the maximum value was previously obtained at 70% in the case of ChG-DST [Citation22, Citation23]. This difference in bonding strengths might be attributed to the hydrophobicity of substituted groups. Self-assembly of cholesteryl groups led to localized phase separation of gel at 70% content, and then bulk strength decreased. We have compared our adhesives with a commercial aldehyde adhesive using a collagen casing. The bonding strength of 10Ste–DST was significantly higher than that of the commercial adhesive (p < 0.05; data not shown).
We predicted that when HMG–DST adhesives are applied to blood vessel, the hydrophobic groups interact with both the matrix components, such as collagen and the cellular components. This was confirmed by exposing SMCs to FITC-labeled HMGs. Figure shows that FITC-labeled 10Ste and 10Ole were integrated into the surface of SMC, indicating that the 10Ste and 10Ole effectively anchor into the SMC cell membrane. Moreover, HMGs did not show cytotoxicity even though the hydrophobic groups anchored into the cell membrane (figure ). Galembeck et al [Citation26] reported that hemolysis was detected at 200 μM of poly(oxyethylene nonylphenol) with nine ethylene oxide units (C9E9). However, no hemolysis was detected in poly(oxyethylene nonylphenol) with longer polyethylene glycol units, such as C9E20 and C9E100. The hydrophile–lipophile balance (HLB) values of C9E9, C9E20 and C9E100 were 10.6, 14.5 and 22.6, respectively. From this result, it was obvious that the high lipophilicity resulted in hemolysis. In this study, the degree of substitution between hydrophobic groups and amino groups of gelatin was less than10%. It may be a reasonable value to conclude that 10Ste and 10Ole have no cytotoxicity.
The bonding strength of HMG–DST adhesives against porcine arterial media was measured in a preliminary experiment. The results were similar to those using the collagen casing; the 10Ste–DST adhesive showed excellent bonding strength compared with the other HMG–DST adhesives or the commercial aldehyde adhesive. This suggests that the Ste group attached to the gelatin molecule interacts with both the hydrophobic amino acid residues of collagen and other kinds of extracellular matrix components, but also with the SMC cell membrane in arterial media. Therefore, we believe that 10Ste–DST is a promising tissue adhesive for use in aortic dissection.
The HMGs, particularly 10Ste, interact with hydrophobic domains, such as hydrophobic amino acid residue and cell membrane. The collagen molecules in extracellular matrix made up three strand structures, which are located outside hydrophobic amino acid residues. Because of the steric structure of collagen, long alkyl chains of HMG show hydrophobic interaction with the hydrophobic amino acid residues of collagen [Citation27]. A collagen-like structure, which introduced the hydrophobic group, forms a stable structure by a hydrophobic interaction [Citation28–Citation30]. Figure shows a proposed bonding mechanism of the 10Ste–DST adhesive with arterial media. The bonding strength is governed by the crosslinking density, tissue penetration, and bulk (gel) strength. The anchoring of 10Ste into cell membrane and the hydrophobic amino acid residue may improve the tissue penetration of the adhesive, which explains why stearoyl groups effectively enhance the bonding strength.
5. Conclusions
We have synthesized gelatin modified with various saturated or unsaturated hydrocarbons. 10Ste–DST showed superior bonding strength against collagen casing, compared with gelatin–DST or the other HMG–DST adhesives. Cell culture experiments demonstrated that 10Ste/10Ole was anchored into the SMC cell membrane without showing cytotoxicity. Therefore, 10Ste–DST is a promising adhesive for use in aortic dissection.
Acknowledgments
We thank Ms A Tsubokawa and Ms T Kojima of the International Center for Materials Nanoarchitectonics (MANA), National Institute for Materials Science (NIMS), for their technical support. This work was financially supported in part by the Japan Society for the Promotion of Science (JSPS) through the ‘Funding Program for World-Leading Innovative R&D on Science and Technology (FIRST Program)’, initiated by the Council for Science and Technology Policy (CSTP), MANA, and the World Premier International Research Center (WPI) Initiative on Materials Nanoarchitectonics, MEXT, Japan.
References
- De MunckJ 2005 J. Dental Res. 84 118 10.1177/154405910508400204
- SungH WHuangD MChangW HHuangR NHsuJ C 1999 J. Biomed. Mater. Res. 46 520 10.1002/(SICI)1097-4636(19990915)46:4%3C520::AID-JBM10%3E3.0.CO;2-9
- TsengY CHyonS HIkadaYShimizuYTamuraKHitomiS 1990 J. Appl. Biomater. 1 111 10.1002/jab.770010203
- BingleyJ A 2000 Ann. Thorac. Surg. 69 1764 10.1016/S0003-4975(00)01250-9
- ChaoH HTorchianaD F 2003 J. Card. Surg. 18 500 10.1046/j.0886-0440.2003.00304.x
- FukunagaSKarckMHarringerWCremerJRheinCHaverichA 1999 Eur. J. Cardiothorac. Surg. 15 564 10.1016/S1010-7940(99)00084-6
- DareE V 2009 Tissue Eng. A 15 2285 10.1089/ten.tea.2008.0228
- IwataHMatsudaSMitsuhashiKItohEIkadaY 1998 Biomaterials 19 1869 10.1016/S0142-9612(98)00095-7
- WallaceD G 2001 J. Biomed. Mater. Res. 58 545 10.1002/jbm.1053
- TaguchiT 2006 Mater. Sci. Eng. C 26 9 10.1016/j.msec.2005.03.006
- IwasashiMSakaneMSaitoHTaguchiTTateishiTOchiaiN 2009 J. Biomed. Mater. Res. A 90 543 10.1002/jbm.a.31905
- TaguchiT 2004 Mater. Sci. Eng. C 24 775 10.1016/j.msec.2004.08.037
- TaguchiT 2007 J. Nanosci. Nanotechnol. 7 742 10.1166/jnn.2007.515
- TaguchiTSaitoHIwasashiMSakaneMOchiaiN 2009 J. Bioact. Compat. Polym. 24 546 10.1177/0883911509349688
- MurakamiY 2007 J. Biomed. Mater. Res. A 80A 421 10.1002/jbm.a.30911
- ArtziNShazlyTCrespoCRamosA BChenaultH KEdelmanE R 2009 Macromol. Biosci. 9 754 10.1002/mabi.200800355
- WangD A 2007 Nature Mater. 6 385 10.1038/nmat1890
- ItoMTaguchiT 2009 Acta Biomater. 5 2945 10.1016/j.actbio.2009.04.015
- RaoZInoueMMatsudaMTaguchiT 2011 Colloids Surf. B 82 196 10.1016/j.colsurfb.2010.08.038
- KatoKItohCYasukouchiTNagamuneT 2004 Biotechnol. Prog. 20 897 10.1021/bp0342093
- RaoZTaguchiT 2011 Polym. Degrad. Stab. 96 1111 10.1016/j.polymdegradstab.2011.03.002
- MatsudaMInoueMTaguchiT 2012 J. Bioact. Compat. Polym. 27 31 10.1177/0883911511434426
- MatsudaMUenoMEndoYInoueMSasakiMTaguchiT 2012 Colloids Surf. B 91 48 10.1016/j.colsurfb.2011.10.030
- OhuchiEImaiKFujiiYSatoHSeikiMOkadaY 1997 J. Biol. Chem. 272 2446 10.1074/jbc.272.4.2446
- BubnisW AOfnerC M 1992 Anal. Biochem. 207 129 10.1016/0003-2697(92)90513-7
- GalembeckEAlonsoAMeirellesN C 1998 Chem. Biol. Interact. 113 91 10.1016/S0009-2797(98)00006-4
- BretscherL EJenkinsC LTaylorK MDeRiderM LRainesR T 2001 J. Am. Chem. Soc. 123 777 10.1021/ja005542v
- CejasM A 2008 Proc. Natl Acad. Sci. USA 105 8513 10.1073/pnas.0800291105
- YuY CBerndtPTirrellMFieldsG B 1996 J. Am. Chem. Soc. 118 12515 10.1021/ja9627656
- YuY CTirrellMFieldsG B 1998 J. Am. Chem. Soc. 120 9979 10.1021/ja981654z