Abstract
Gelatins were crosslinked with organic acids and treated with alkali to impart to them endothelialization and anti-thrombogenic properties. These matrices were characterized by biochemical and physicochemical techniques. The amounts of residual amino groups in the matrices decreased with increasing crosslinker concentration. The matrices with the highest crosslinking densities showed excellent endothelial cell adhesion and proliferation. In addition, the adhesion of platelets and formation of fibrin networks on the matrices were suppressed with increasing crosslinker concentration. The matrices also exhibited excellent biodegradability, and the degradation rate decreased with increasing crosslinking density. All the organic acid-crosslinked alkali-treated gelatins showed excellent anti-thrombogenic and endothelialization properties, superior to those of glutaraldehyde-crosslinked alkali-treated gelatins.
1. Introduction
Circulatory diseases are the second most common cause of death in Japan. Cardiovascular devices such as coronary stents, heart valves and artificial blood vessels have been used for the treatment of these diseases. Once these devices are inserted in the body, their surfaces come in contact with blood [Citation1, Citation2]. To prevent thrombus formation on such devices, many types of biomaterials with biomimetic, non-fouling and biomolecule-immobilized surfaces have been developed [Citation3–Citation6]. These surfaces have excellent hemocompatibility, but inhibit adsorption of cell adhesion proteins, and therefore endothelial cells cannot adhere to them. To overcome this disadvantage, collagen [Citation7], growth factor [Citation8] and cell adhesion peptide [Citation9] were immobilized on these surfaces to promote endothelial cell adhesion, proliferation and migration. However, these surfaces do not show anti-thrombogenic properties. Therefore, a novel biomaterial that has anti-thrombogenic properties and yet allows endothelial cell adhesion/proliferation is required for long-term stability when implanted in cardiovascular tissue.
Gelatin is known as a biopolymer with excellent biocompatibility and biodegradability. In addition, gelatin chains contain cell adhesion motifs, such as arginine–glycine–aspartic acid (RGD) sequences, thereby improving its biological behavior over polymers that lack these cell-recognition sites [Citation10]. However, gelatin is rarely used in clinical applications, because aqueous gelatin solutions have a sol–gel transition point around human body temperature. Various chemical crosslinkers, such as glutaraldehyde [Citation11, Citation12], carbodiimide [Citation13] and epoxy [Citation14] compounds, have been used to overcome this problem. However, gelatin hydrogels prepared using these chemicals are cytotoxic and induce chronic inflammation. Recently, we have developed the organic acid-derived crosslinkers disuccinimidyl tartrate (DST), disuccinimidyl malate (DSM) and trisuccinimidyl citrate (TSC) [Citation15–Citation18]. These crosslinkers contain substituted carboxyl groups of organic acid with active esters and form biopolymer matrices via a reaction between the biopolymer and the active ester groups of the crosslinker. The resulting matrices have been shown to possess high mechanical strength and cytocompatibility [Citation19–Citation21].
Here, we report three types of organic acid-crosslinked gelatins exhibiting endothelialization and anti-thrombogenic properties. These gelatins were prepared with various crosslinking densities, and their biochemical and physicochemical properties (including the water content, concentration of the residual amino groups in the matrices, endothelialization, anti-thrombogenic properties and biodegradability) were investigated.
2. Materials and methods
2.1. Materials
AlGelatin (beMatrix) derived from porcine skin was provided by Nitta Gelatin Inc. (Osaka, Japan). AlGelatin with carboxyl groups generated by hydrolysis of the residual amide groups present in the asparagine and glutamine residues has an isoelectric point of 5. Tartaric acid, malic acid, citric acid, 25% glutaraldehyde (GA) tetrahydrofuran (THF), N-hydroxysuccinimide (NHS), dimethyl sulfoxide (DMSO), lactic acid and 2,4,6-trinitrobenzenesulfonic acid (TNBS) were purchased from Wako Pure Chemical Industries, Ltd (Osaka, Japan). N,N'-Dicyclohexylcarbodiimide (DCC) was purchased from Tokyo Chemical Industry Co., Ltd (Tokyo, Japan). Collagenase was purchased from Nacalai Tesque (Kyoto, Japan). All chemical reagents were used without further purification.
2.2. Synthesis of the organic acid-derived crosslinkers
DST, DSM and TSC were prepared according to the previously reported methods [Citation15–Citation18]. Briefly, tartaric acid, malic acid or citric acid, respectively, was dissolved in THF, and subsequently NHS and DCC were added to this mixture. After stirring the mixture for 30 min, it was concentrated to remove THF. The resulting mixture was recrystallized from ethanol to yield DST, DSM or TSC. Characterization of the resultant crosslinkers by proton nuclear magnetic resonance spectroscopy (1H-NMR; JEOL EX-300) yielded the following results:
DST: 1H-NMR (DMSO-d6) δ = 2.8 (s, 8H, CH2 × 4), 4.8–4.9 (d, 2H, CH × 2), 6.73 (d, 2H, OH × 2). C12H12 N2O10: calculated C 41.90, H 3.52, N 8.14; found C 42.22, H 3.47, N 8.33.
DSM: 1H-NMR (DMSO-d6) d = 2.8 (s, 8H, CH2 × 4), 3.1–3.3 (m, 2H, CH2), 4.8–4.9 (m, 1H, CH), 6.73 (d, 1H, OH). C12H12 N2O9: calculated C 43.91, H 3.68, N 8.53; found C 43.76, H 3.51, N 8.40.
TSC: 1H-NMR (DMSO-d6) d = 2.8 (s, 12H, CH2 × 4), 3.4 (s, 4H, CH2 × 2), 7.2 (s, 1H, OH). C18H17 N3O13: calculated C 44.73, H 3.55, N 8.69; found C 44.83, H 3.45, N 8.58.
2.3. Preparation of the matrices
AlGelatin was dissolved in DMSO containing 10% (w/w) of lactic acid to obtain a 10% (w/v) solution. DST, DSM and TSC solutions of various concentrations were prepared in DMSO containing 10% of lactic acid and were sterilized by filtration with a 0.2 μm pore filter (Mille-LG, Millipore, Billerica, MA). GA-crosslinked AlGelatin (AlGelatin–GA) was prepared without lactic acid. The crosslinker solutions were added to the AlGelatin solutions such that the mixing ratios of succinimidyl (NHS) groups of the crosslinker to the amino (NH2) groups of the AlGelatin were 0.5, 1 and 2. Each AlGelatin and crosslinker solution was stirred and then poured into a mould with a 1 mm silicone rubber spacer between two glass plates for 24 h at 25 °C (figure ). The matrices were subsequently immersed in excess sterilized water for 3 days at 4 °C to remove the solvent and reaction by-products from the matrix. The gelatin matrices were then punched out in a disc-like shape (10 mm diameter, 1 mm thickness). For the cell culture experiments, the matrices were prepared under sterile conditions.
2.4. Characterization of the matrices
The water content of the matrices was determined using the following equation: where Ww and Wd are the weights of the matrix when immersed in water and after freeze-drying, respectively. The residual amino groups in the matrices were determined using a spectrophotometric method with TNBS [Citation22]. Briefly, 2 ml of a 4% sodium hydrogen carbonate solution, 2 ml of a 0.1% TNBS solution and 1 ml of water were added to 10 μg of dried matrix, followed by incubation for 2 h at 37 °C. Subsequently, 5 ml of 6 M hydrochloric acid solution was added, followed by heating for 2 h at 70 °C to hydrolyze the matrix. The absorbance values of the mixed solutions were measured at 340 nm using a plate reader (GENiosA-5082, Tecan Group Ltd, Männedorf, Switzerland).
2.5. Culture of endothelial cells on the matrices
Human umbilical vein endothelial cells (HUVECs) were purchased from Lonza (Walkersville, Maryland, USA) and used to evaluate endothelialization on the sterilized matrices. HUVECs were cultured in basal endothelial medium (EBM-2) supplemented with 2% fetal bovine serum (FBS), human fibroblast growth factor-basic (hFGF-B), epidermal growth factor (EGF), R3-insulin-like growth factor (R3-IGF-1) and gentamicin, following the manufacturer's protocol (Lonza). Matrices were placed on 48-well plates and pre-incubated in the culture medium for 24 h. The HUVECs (2 × 104 cells) were then seeded onto each matrix and incubated at 37 °C with 5% CO2. After culturing for 1 and 7 days, the HUVECs seeded onto the matrices were washed twice with 1 ml of 0.1 M phosphate buffered saline (PBS, pH 7.4). The adhering HUVECs on each matrix were counted using WST-8 (Dojindo, Kumamoto, Japan), a colorimetric assay reagent for cellular dehydrogenase activity; its absorbance at 450 nm is proportional to the dehydrogenase activity in the cell. To confirm the morphology of the HUVECs on the surface, each substrate was removed from the plate after incubation. The HUVECs were fixed in 10% formalin neutral buffer solution for 60 min and permeabilized in 0.2% Triton-X 100 in PBS for 2 min. They were then incubated in Alexa Fluor 488 phalloidin diluted in PBS for 60 min and in 0.1% 4',6-diamidino-2-phenylindole (DAPI) in PBS for 10 min in the dark at room temperature. They were subsequently observed using an IX71 inverted fluorescence microscope (Olympus Co., Japan).
2.6. Evaluation of the anti-thrombogenic properties
The anti-thrombogenic properties of the matrices were evaluated according to established methods [Citation23, Citation24]. Each matrix was pre-incubated in 0.1 M PBS for 24 h, and then placed in a tube and immersed in 1.0 ml of whole rat blood (male Wistar rats, 12–16 weeks old, Charles River, Japan) for 15 min at 37 °C. After incubation, the matrix was removed and gently rinsed three times with PBS. The matrix was then fixed with 10% neutral buffered formalin and subjected to critical point drying using tert-butyl alcohol. All experiments were carried out with the approval of the Animal Care and Use Committee of the National Institute for Material Science.
2.7. Enzymatic degradation of the matrices
Enzymatic degradation tests on the matrices were performed using a previously reported method [Citation25, Citation26]. Disc-like matrices were immersed in 5 ml of 0.1 M tris-hydrochloric acid buffer solution (pH 7.4, 2.5 mM calcium chloride) containing 30 units ml−1 of collagenase at 37 °C. After various immersion times, the matrices were weighed and the percentage of weight loss was calculated.
2.8. Scanning electron microscopy (SEM)
SEM observations were carried out using a JSM-5600 microscope (JEOL, Tokyo, Japan). The sample was coated with platinum using an ESC-101 SEM sample coating system (Elionix, Tokyo, Japan).
2.9. Statistical analysis
The results are expressed as the mean ± standard deviation (SD). Statistical comparisons were made using a two-tailed Student's t-test with Microsoft Office Excel 2010.
3. Results
3.1. Water content of the matrices
To investigate the effects of the crosslinker concentration on crosslinking density, the water content of the matrices was evaluated and the results are shown in figure . The water content for all the crosslinked matrices, except for that with GA, shows minimum values with equimolar amounts of the active ester and amino groups (NHS/NH2 = 1). According to the Flory–Rehner equation, the crosslinking density of the matrix increases with decreasing water content. In addition, at the same crosslinker concentration, a lower water content was obtained for DST, followed by TSC and DSM. In contrast, the water content of the AlGelatin–GA matrix consistently decreased with increasing GA concentration.
3.2. Determination of the residual amino groups in the matrix
Figure shows the concentration of the residual amino groups in AlGelatins prepared with different crosslinker concentrations. The concentration of the residual amino groups in the AlGelatin–DST, –DSM and –TSC matrices decreased with increasing crosslinker concentration, and only a few residual amino groups were detected at high crosslinker concentrations. On the other hand, the concentration of the residual amino groups in the AlGelatin–GA matrix decreased much more gradually with GA concentration.
3.3. Adhesion and proliferation of HUVECs on the matrix
Figure shows the surface density of HUVECs on the AlGelatin–DST, –DSM, –TSC and –GA matrices with different crosslinker concentrations after being cultured for 1 and 7 days. HUVECs were not observed on the AlGelatin–GA matrix. Conversely, high HUVEC densities were detected on the organic acid-crosslinked AlGelatins, after 1 or 7 days of culture. On the AlGelatin–DST where the DST concentration represents a ratio of NHS/NH2 = 1, the HUVEC density reached a maximum of 3234 ± 1509 cells cm−2. A similar tendency was observed for the AlGelatin–DSM and –TSC matrices. At the same crosslinker concentration (NHS/NH2 = 1), the density of cells on AlGelatin–TSC was the highest compared with those on AlGelatin–DST and –DSM after 1 day of culture. After culturing for 7 days, the density of HUVECs grew 8–23 times, and the relationship between the crosslinker concentration and HUVEC density was nearly the same as that after culturing for 1 day. To examine the effect of FBS on cell adhesion, HUVECs were cultured in culture medium without FBS on AlGelatin–DST as an example. On the AlGelatin–DST where the DST concentration represents a ratio of NHS/NH2 = 1, the density of HUVECs reached a maximum of 2244 ± 150 cells cm−2, whereas it was 1775 ± 199 cells cm−2 for NHS/NH2 = 0.5 and 1589 ± 311 cells cm−2 for NHS/NH2 = 2. HUVEC densities were similar with or without FBS in culture medium. Figure shows the morphology of the HUVECs on the AlGelatin–DST, –DSM, –TSC and –GA matrices with different crosslinker concentrations. The morphology of the cell proliferation was confirmed to correspond to the density of cells on all the matrices. After culturing for 1 day, round-shaped HUVECs were observed on the AlGelatin–DST, –DSM and –TSC matrices with a low crosslinker concentration (NHS/NH2 = 0.5), while stretched HUVECs were observed on the matrices with high crosslinker concentrations (NHS/NH2 = 1). However, after 7 days of culture, all the HUVECs were stretched, regardless of the crosslinker concentration.
3.4. Anti-thrombogenic properties of the matrices
Figure shows the AlGelatin–DST, –DSM and –TSC matrices with different crosslinking densities after immersion in rat whole blood. The AlGelatin–GA matrices were employed as a control. Considerable thrombus formation was observed on the surfaces of all the AlGelatin–GA matrices and on the AlGelatin–DST, –DSM and –TSC matrices with low crosslinker concentrations (NHS/NH2 = 0.5). However, no thrombus formation was observed on the AlGelatin–DST, –DSM and –TSC matrices with a high crosslinker concentration (NHS/NH2 1). Figure shows SEM images of the surfaces of the AlGelatin–DST, –DSM, –TSC and –GA matrices after the thrombus formation test. Platelets and red blood cells impregnated in the fibrin network were observed on the surfaces of the AlGelatin–GA and AlGelatin–DST, –DSM and –TSC matrices with a low crosslinker concentration (NHS/NH2 = 0.5). On the other hand, no fibrin networks or platelet adhesion was detected on the surfaces of the AlGelatin–DST, –DSM and –TSC matrices with high crosslinker concentrations (NHS/NH2 1).
3.5. Degradation behavior of the matrices
To evaluate the enzymatic degradation behavior, AlGelatin–DST, –DSM, –TSC and –GA matrices with different crosslinking densities were immersed in a collagenase solution. Then their remaining weight was measured and the results are presented in figure . All the AlGelatin matrices are gradually digested by collagenase, with the stability of the AlGelatin–DST, –DSM and –TSC matrices toward enzymatic degradation varying in the order NHS/NH2 = 1 > 2 > 0.5. When the crosslinker concentration is NHS/NH2 = 1, the degradation time for AlGelatin–DST is 90 min and for AlGelatin–DSM and –TSC is 75 min. The degradation of AlGelatin–DST was the slowest of all the matrices examined in this study. On the other hand, the degradation time for the AlGelatin–GA matrices was elevated with increasing GA concentration.
Figure 8 Residual weight of the AlGelatin–DST (a), –DSM (b), –TSC (c) and –GA (d) matrices with different crosslinker concentrations after immersion in a collagenase solution. The crosslinker concentrations of the NHS or CHO/NH2 groups are 0.5 (•), 1 (△) and 2 (▪). Error bars represent the standard deviations (n = 3).
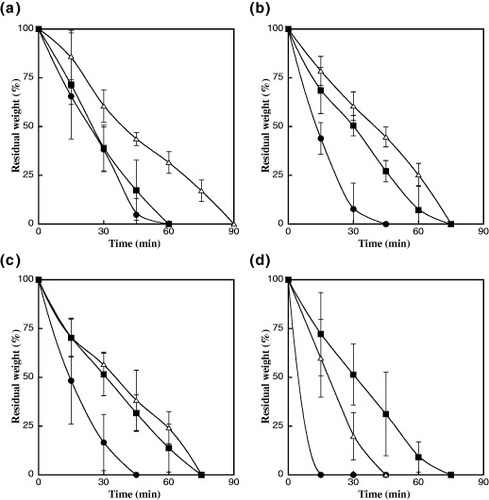
4. Discussion
Anti-thrombogenic and endothelialization properties are important surface characteristics of blood-contacting materials. To design materials with such properties, we hypothesized that their surfaces should have both a negative charge and a cell adhesive sequence. On the basis of this concept, we fabricated organic acid-crosslinked AlGelatins with various crosslinking densities and evaluated their anti-thrombogenic and endothelialization properties. The water content of the gelatins increased in the crosslinker order DSM > TSC > DST. The excess active ester groups in the crosslinker are hydrolyzed to the corresponding carboxyl groups, and thus, the surface of the matrices is assumed to be negatively charged.
Platelets and fibrin networks were observed on all the AlGelatin–GA matrices and the AlGelatin–DST, –DSM and –TSC matrices with a low crosslinker concentration (NHS/NH2 = 0.5; see figures –). Because AlGelatin has a cell adhesion sequence (such as RGD), plates adhered and fibrin networks formed on the surface of these matrices. However, no platelets or fibrin networks were observed at high DST, DSM or TSC concentrations (NHS/NH2 1). This observation results from the introduction of the negatively charged carboxyl groups generated from the hydrolyzed active ester groups of the organic acid-derived crosslinker. In figure , it can be seen that almost all the amino groups react with the active ester groups of the organic acid-derived crosslinker at high crosslinker concentration. This result suggests the formation of an AlGelatin network that bears active ester groups. Through this process, negatively charged groups were introduced into the AlGelatin matrices. There are two types of reactivity for the active ester groups in DSM and TSC. It is thought that the highly reactive esters participate in matrix formation, while the less-reactive esters contribute to the generation of the carboxyl groups. Furthermore, AlGelatin has an isoelectric point of 5. Therefore, the anionic charge of AlGelatin also contributes to the expression of anti-thrombogenic properties by the matrices.
In general, hydrophilic surfaces inhibit cell adhesion because they reduce the adsorption of certain cell proteins on the surface of the matrix [Citation27, Citation28]. In this study, the number of HUVECs on the organic acid-crosslinked AlGelatins peaked with equimolar amounts of NHS and amino groups (NHS/NH2 = 1), regardless of the type of organic acid crosslinker. Correspondingly, the water content of the AlGelatin matrix with NHS/NH2 = 1 was much lower than that of the AlGelatin matrix with any other crosslinker concentration. Therefore, the density of the cell adhesion sequence derived from AlGelatin on the matrix at NHS/NH2 = 1 is thought to be much higher than that with other crosslinker concentrations. In addition, HUVECs have not been observed on the AlGelatin–GA matrix for any GA concentration, even after incubation for 7 days. This result implies that unreacted aldehyde or hydrolyzed GA in the matrix exhibited cytotoxicity and inhibited cell proliferation.
The biodegradability of the organic acid-crosslinked AlGelatins was also studied in vitro. Collagenase (pI = 5.2) cleaves a unique peptide bond site in the triple-helical structure of gelatin and induces fragmentation between X and glycine in the sequence proline-X-glycine, where X is any amino acid [Citation29]. All the matrices were degraded after immersion into a collagenase solution, with the organic acid-crosslinked AlGelatins with NHS/NH2 = 1 showing the highest stability against enzymatic degradation. Interestingly, there was no significant difference in the crosslinking density between NHS/NH2 = 0.5 and 2; however, the degradation rate was higher for NHS/NH2 = 0.5 than 2 in vitro. We speculate that the matrices with a high crosslinker concentration (NHS/NH2 = 2) are degraded slowly because of the negatively charged surface, which causes an electrostatic repulsion with the collagenase in the in vitro environment. Figure shows the anti-thrombogenic, endothelialization and degradation properties of the organic acid-crosslinked AlGelatins. When the NHS/NH2 ratio is greater than 1, the resulting AlGelatin matrices possess anti-thrombogenic, endothelialization and collagenase-durable properties because of the introduction of the carboxyl groups derived from the active ester groups of the organic acid-derived crosslinker. In a previous study, we confirmed that crosslinked gelatin remained for 8 weeks in rat body [Citation25]. Owing to these properties, these matrices have potential as coating materials for cardiovascular devices such as coronary stents, heart valves and artificial blood vessels.
5. Conclusions
We have developed biodegradable organic acid-crosslinked AlGelatins that possess both anti-thrombogenic and endothelialization properties. In particular, the organic acid-crosslinked AlGelatins with the highest crosslinking density exhibit excellent adhesion and proliferation of endothelial cells; they also inhibit platelet adhesion and fibrin network formation. The AlGelatin matrices were durable against collagenase when the NHS/NH2 ratio of the matrices was greater than 1. These results indicate that the AlGelatin matrices with anti-thrombogenic and endothelialization properties have great potential for cardiovascular applications.
Acknowledgments
We thank Ms M Ueno of the Biomaterials Unit of Nano-Life Field at the National Institute for Materials Science of Japan for the technical support. This research was supported by the Japan Society for the Promotion of Science (JSPS) through its Funding Program for World-Leading Innovation R&D on Science and Technology (FIRST Program). This research was also financially supported in part by a Grant-in-Aid from the National Institute of Biomedical Innovation of Japan and the World Premier International Research Center (WPI) Initiative on Materials Nanoarchitectonics, MEXT, Japan.
References
- VeselyI 2005 Circ. Res. 97 743 10.1161/01.RES.0000185326.04010.9f
- SchmidtC EBaierJ M 2000 Biomaterials 21 2215 10.1016/S0142-9612(00)00148-4
- SagnellaSMai-NgamK 2005 Colloids Surf. B 42 147 10.1016/j.colsurfb.2004.07.001
- ShinHJoSMikosA G 2003 Biomaterials 24 4353 10.1016/S0142-9612(03)00339-9
- IshiharaK 2000 Sci. Technol. Adv. Mater. 1 131 10.1016/S1468-6996(00)00012-7
- IshiharaKOshidaHEndoYUedaTWatanabeANakabayashiN 1992 J. Biomed. Mater. Res 26 1543 10.1002/jbm.820261202
- TamadaYIkadaY 1994 J. Biomed. Mater. Res. 28 783 10.1002/jbm.820280705
- ItoYShu QinLImanishiY 1991 Biomaterials 12 449 10.1016/0142-9612(91)90141-V
- CholletC 2009 Biomaterials 30 711 10.1016/j.biomaterials.2008.10.033
- YoungSWongMTabataYMikosA G 2005 J. Control. Release 109 256 10.1016/j.jconrel.2005.09.023
- BigiACojazziGPanzavoltaSRubiniKRoveriN 2001 Biomaterials 22 763 10.1016/S0142-9612(00)00236-2
- GoughJ EScotchfordC ADownesS 2002 J. Biomed. Mater. Res. 61 121 10.1002/jbm.10145
- TsaiC CHuangR NSungH WLiangH C 2000 J. Biomed. Mater. Res. 52 58 10.1002/1097-4636(200010)52:1%3C58::AID-JBM8%3E3.0.CO;2-0
- Van WachemP B 1999 J. Biomed. Mater. Res. 47 270 10.1002/(SICI)1097-4636(199911)47:2%3C270::AID-JBM18%3E3.0.CO;2-D
- TaguchiTSaitoHIwasashiMSakaneMOchiaiN 2009 J. Bioact. Compat. Polym. 24 546 10.1177/0883911509349688
- KakinokiSTaguchiTSaitoHTanakaJTateishiT 2007 Eur. J. Pharm. Biopharm. 66 383 10.1016/j.ejpb.2006.11.022
- SaitoHMurabayashiSMitamuraYTaguchiT 2007 Biomacromolecules 8 1992 10.1021/bm070122k
- SaitoH 2007 Acta Biomater. 3 89 10.1016/j.actbio.2006.08.003
- MatsudaMInoueMTaguchiT 2012 J. Bioact. Compat. Polym. 27 31 10.1177/0883911511434426
- MatsudaMUenoMEndoYInoueMSasakiMTaguchiT 2012 Colloids Surf. B 91 48 10.1016/j.colsurfb.2011.10.030
- InoueMSasakiMNakasuATakayanagiMTaguchiT 2012 Adv. Healthcare Mater. 1 573 10.1002/adhm.201200001
- BubnisW AOfner IiiC M 1992 Anal. Biochem. 207 129 10.1016/0003-2697(92)90513-7
- SerizawaTYamaguchiMMatsuyamaTAkashiM 2000 Biomacromolecules 1 306 10.1021/bm000006g
- MatsusakiMKamezawaTShimozuruTKuratsuJ IKishidaAAkashiM 2003 J. Biomed. Mater. Res. B 66 429 10.1002/jbm.b.10030
- InoueMSasakiMTaguchiT 2010 Polym. Degrad. Stab. 95 2088 10.1016/j.polymdegradstab.2010.06.029
- SaitoH 2004 Mater. Sci. Eng. C 24 781 10.1016/j.msec.2004.08.039
- NuttelmanC RHenryS MAnsethK S 2002 Biomaterials 23 3617 10.1016/S0142-9612(02)00093-5
- LeeJ HGoA KOhS HLeeK EYukS H 2005 Biomaterials 26 671 10.1016/j.biomaterials.2004.03.009
- SakuraiY 2009 Biosci. Biotechnol. Biochem. 73 21 10.1271/bbb.80357