Abstract
An in situ microscopic observation of the magnetic orientation process of feeble magnetic fibers was carried out under high magnetic fields of up to 10 T using a scanning laser microscope. In the experiment, carbon fibers and needle-like titania fibers with a length of 1 to 20 μm were used. The fibers were observed to gradually orient their axes parallel to the direction of the magnetic field. The orientation behavior of the sample fibers was evaluated on the basis of the measured duration required for a certain angular variation. As predicted from the theoretical consideration, it was confirmed that the duration required for a certain angular variation normalized by the viscosity of the fluid is described as a function of the fiber length. The results obtained here appear useful for the consideration of the magnetic orientation of materials suspended in a static fluid.
Introduction
Magnetic orientation techniques using the magnetic anisotropy of crystals have been attracting considerable attention in material processing control applications. When small crystals with magnetic anisotropy are placed in magnetic fields, magnetic torque acts on the crystals. The crystals are then rotated so as to align their maximum magnetic susceptibility axes parallel to the direction of the magnetic fields. In the case of feeble magnetic materials, namely, diamagnetic and paramagnetic ones, the use of high magnetic fields makes it possible to control their structure even if the material has only a slight magnetic anisotropy. In materials processing, the control of the material orientation seems effective for improving their properties, including their mechanical properties and electrical conductivity. Widely used conventional techniques for this purpose involve mechanical orientation. However, this technique is effective only near the surface of materials. Therefore, in the case of bulk materials, it is difficult to obtain entirely uniform material. Moreover, in some cases, the fine structures of crystals may be destroyed by this method. On the other hand, in the case of magnetic orientation, magnetic torque acts on the crystals without any direct mechanical contact and are effective even deeply within bulk materials. Therefore, this technique is expected to eliminate the problems of mechanical orientation.
The application of magnetic orientation for the fabrication of feeble magnetic materials has already been investigated in many materials, such as polymers, ceramics, and inorganic and composite materials [Citation1–4]. However, the behaviors of such materials in practical surroundings or solvents when they are magnetically oriented are not well understood. As a result, alignment control by magnetic fields is currently being carried out under excessively strenuous conditions in many cases. This causes problems with cost in practical industrial applications because of the need for excessive equipment investment and reductions in manufacturing efficiency. For the practical application of alignment control by magnetic fields, further understanding of the magnetic orientation behavior is required to optimize the conditions and improve the efficiency.
There are some theoretical approaches to evaluate the degree of orientation or the behavior of materials suspended in certain surroundings. Some of them statistically treat the distribution of the direction of particles under a thermodynamical equilibrium. The evaluation of the behavior of single particles during a magnetic orientation process has also been reported [Citation5–7]. Such theoretical discussions seem to contribute to the optimization of the process conditions if they are supported by comparable experiments under practical conditions. However, the microscopic observation of the orientation process of feeble magnetic materials under practical conditions is quite difficult. The reasons for this are as follows: the feeble magnetic materials used in such cases are approximately several micrometers in size; for the magnetic orientation of feeble magnetic materials of this size, high magnetic fields attained by superconducting magnets are required; the space in which high magnetic fields are generated is limited to the inside of a metal cylinder (magnet bore) with a diameter of approximately 100 mm at room temperature; the magnetic materials cannot be used under high magnetic fields; therefore, conventional microscopes cannot be used.
Recently, we developed a periscope system for in situ high-resolution observations under high magnetic fields in combination with a confocal scanning laser microscope [Citation8]. This apparatus enables optical observations under high magnetic fields with a resolution of at least 800 nm. To contribute to the understanding of the magnetic orientation process of feeble magnetic materials, microscopic observations of the magnetic orientation process of feeble magnetic fibers were carried out under high magnetic fields of up to 10 T using this periscope system.
Experimental
Carbon fibers and needle-like titania fibers with a length of 1 to 20 μm were used as samples of feeble magnetic materials that have magnetic anisotropy. The magnetization easy axis of these samples was parallel to their long axis. These fibers were mixed with silicone oil and suspended in it using an ultrasonic homogenizer. The viscosity of the silicone oils used here ranged from 5 to 50 Pa•s. The concentration of fibers in the silicone oil was adjusted to 0.1 wt%. Thus, the obtained sample slurry was poured into the glass optical cell and used for the in situ optical observation. The inner size of the optical cell used here was 8.6 mm in width, 8.6 mm in depth, and 20 mm in height.
A conduction-cooled type of the superconducting magnet, JMTD-10C13E-NC manufactured by JASTEC Co., Ltd., was used to apply the magnetic field. This magnet has a room-temperature bore of 100 mm in diameter. The magnetic field of up to 13 T can be applied at the center of the bore axis. For the in situ microscopic observation of the magnetic orientation process of feeble magnetic material fibers under high magnetic fields, a periscope system with the scanning laser microscope was used. In this system, the laser light source is set outside of the superconducting magnet to avoid the effect of the magnetic field on the function of the scanning laser microscope. The laser was introduced into the inside of the magnet using the optical extension unit, the periscope. A schematic illustration of the experimental system is shown in figure . The bore axis of the superconducting magnet was set horizontally. The optical cell that contains the slurry was set in front of the objective lens and was observed from the side using a mirror.
Under the initial condition, the angle of the long axis of the fibers was randomly distributed in the slurry. The fibers were then rotated three-dimensionally when the optical cell was introduced into the magnetic field. If the observation of the magnetic orientation process started under such a condition, it would be very difficult to evaluate the rotation process because the information obtained using the laser microscope would be limited in two dimensions in order to observe the time dependence of the fiber rotation. Therefore, in this experiment, first, we put the optical cell in the magnetic field for some time to align all fibers parallel to the magnetic field. We then rotated the optical cell 90° and began the observation. To do this, the initial angle of the fiber axis was uniformly perpendicular to the magnetic field, and the rotation of the fibers was limited to two dimensions.
Results and discussion
Figure shows the observation results of the behavior of needle-like titania fibers suspended in 5 Pa•s silicone oil under a 10 T magnetic field. At the beginning, the directions of the fibers were almost perpendicular to the direction of the magnetic field, as shown in figure (a). The fibers were then gradually rotated by magnetic torque and finally aligned parallel to the direction of the magnetic field. Similar results were obtained in experiments under other conditions.
Figure 2 Results of in situ observation of magnetic orientation process of needle-like titania fibers under a 10 T magnetic field.
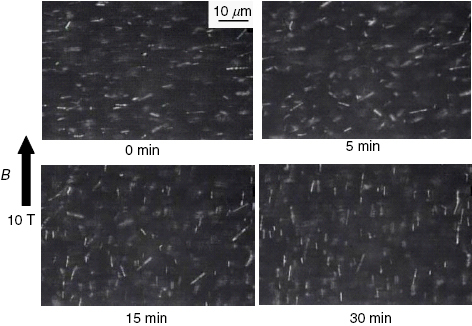
Let us assume that the fiber is cylindrical. The angle between the fiber axis and the direction perpendicular to the magnetic field is defined as θ. Therefore, at the beginning of the observation, the angle θ is zero, and, after the magnetic orientation is completed, it will be 90°.
When Δχ represents the anisotropy of the magnetic susceptibility of the fiber, that is, the difference in the volume magnetic susceptibilities between the axial direction and the radial direction of the fiber, the magnetic torque acting on the fiber, Tm, and the viscous resistance, Tv, are expressed as follows:
(1)
(2)
where V is the volume of the fiber, B is the magnetic flux density, μ0 is the permeability in vacuum, and ξR is the rotational friction coefficient and is a direct function of the viscosity. The following relationship is known for the rotational diffusion coefficient, DR [Citation9],
(3)
where kB is the Boltzmann constant, T is the temperature, L is the fiber length, and s is the length-to-diameter ratio. Therefore, the relation between ξR and the viscosity, η, is expressed as
(4)
The inertia term is negligibly small in the system treated here; therefore, the motion of rotation is described by the balance between the magnetic torque and the viscous resistance. Thus, the time, t, required to rotate the fiber from θ0 to θ is expressed by solving the relationship Tm=Tv as
(5)
To confirm this relationship, the duration required for the rotation from 20° to 80° was measured for each fiber from the observation results. Thus, the measured duration was normalized by the viscosity of silicone oil, and the results are discussed relative to the data obtained in fluids of different viscosities and plotted in figure against the length of fiber. Figure (a) shows the results for carbon fibers, and figure (b) shows those for needle-like titania fibers. The length of each fiber is measured from the observation results. Even though the results are slightly scattered in the case of low-viscosity fluids owing to the convection of fluid and the sedimentation of fibers, the required rotation time normalized by the viscosity was distributed similarly against the length of the fiber. The solid lines in the figures represent the results of the fitting based on equation (5). The results obtained using silicone oil of 50 Pa•s were used for the fitting in the case of carbon fibers; for needle-like titania fibers, results obtained using 10 Pa•s fluid were used. The diameters of the fibers were determined by averaging the values measured in the observation. The resulting value was 0.8±0.2 mm. Consequently, in this calculation, a constant value of 0.8 μm was used for all fibers. Therefore, the volume, V, depends on the length of the fiber. The distribution of the data follows the fitting curve well. It was confirmed that the duration required for a certain angular variation normalized by the viscosity of the fluid is expressed as a function of fiber length. It is also possible to estimate the anisotropy of the magnetic susceptibility using this plot and the fitted curve. The values of the magnetic anisotropy obtained in these experiments are shown in the figures. For comparison, curves simulated using different Δχ values are also shown in the figures. The results obtained here may be useful for the optimization of the magnetic orientation condition of feeble magnetic materials in a practical process.
Conclusion
In this study, to contribute to the understanding of the magnetic orientation process of feeble magnetic materials, in situ microscopic observations of the magnetic orientation process of feeble magnetic fibers were carried out under high magnetic fields of up to 10 T. As a result, we succeeded in observing the behavior of carbon fibers and needle-like titania fibers with lengths of 1 to 20 μm during the magnetic orientation process. Then, the duration required to rotate from 20° to 80° was measured for each fiber. As predicted from theoretical considerations, the duration of time required for a certain angular variation normalized by the viscosity of the fluid is described as a function of the fiber length. The results obtained here appear useful for the consideration of the magnetic orientation condition for materials suspended in a static fluid. In this study, the dispersion density of fibers was diluted considerably more than it is in a practical process. In concentrated slurry, the interaction between fibers, such as friction or physical contact, should be taken into account. Further investigation will pave the way to industrial applications.
References
- KimuraT 2003 Polym. J. 35 823 http://dx.doi.org/10.1295/polymj.35.823
- FujiwaraMChidiwaTTanimotoY 2000 J. Phys. Chem. B 104 8075 http://dx.doi.org/10.1021/jp994469w
- SuzukiT SSakkaYKitazawaK 2001 Adv. Eng. Mater. 3 490 http://dx.doi.org/10.1002/1527-2648(200107)3:7<490::AID-ADEM490>3.0.CO;2-O
- HoriiS et al 2003 Japan. J. Appl. Phys. 42 7018 http://dx.doi.org/10.1143/JJAP.42.7018
- KimuraTYamatoMKoshimizuWKoikeMKawaiT 2000 Langmuir 16 858 http://dx.doi.org/10.1021/la990761j
- MichaudBBeaugnonESulpiceATournierRClaverieJ 2000 Mat. Trans. JIM 41 962
- KimuraTYoshinoMYamaneTYamatoMTobitaM 2004 Langmuir 20 5669 http://dx.doi.org/10.1021/la049347w
- HirotaNOdeT 2006 Rev. Sci. Instrum. 77 036107 http://dx.doi.org/10.1063/1.2173071
- TiradoM MMartinezC Lde la TorreJ G 1984 J. Chem. Phys. 81 2047 http://dx.doi.org/10.1063/1.447827