Abstract
The physical properties of agarose gel prepared under strong magnetic fields were investigated. The storage modulus was measured by the reflection method with an ultrasonic pulse. The measurement results of the gel's elasticity indicate that agarose gel has anisotropic properties. The elasticity and its anisotropy depend on the concentration of the gel and the magnetic field to which it is exposed. The experimental results indicate that the anisotropic network structure of the gel is induced by the exposure to the magnetic field during gelation. The gelation mechanism under a magnetic field is discussed.
Introduction
Polysaccharide agarose consists of many sequences of 1,3-linked β-D-galactopyranose and 1,4-linked 3,6 anhydro-α-L-galactopyranose units [Citation1]. The aqueous solution of agarose undergoes a thermally reversible sol-gel phase transition. Agarose is classified into several types according to the melting temperature and elasticity. The transition temperature of a typical agarose solution is about Tg=30 ∘C for gelation. The following gelation model, based on the increase in the hydrophobic property of the agarose chains, has been suggested to govern the cooling process [Citation2]. The random coil shape of the agarose molecule changes into an α-helix with decreasing temperature. Two helices intertwine to form a double helix. Many double helices self-align and self-assemble to form crystalline regions. Linkages among these crystalline regions are generated and enhanced to yield a gel at the phase transition temperature. The agarose gel has a sieve structure that corresponds to the linkages.
We have reported that the physical properties of agarose gel formed under strong magnetic fields change. Such a gel shows an increase in the melting temperature [Citation3, Citation4], marked changes in its anisotropic properties [Citation5, Citation6], birefringence and anisotropic shrinkage, and an anisotropic increase in the electrophoresis velocity of DNA inside the gel. The results of the birefringence measurement indicated that the agarose molecule was ordered perpendicularly to the magnetic flux to which it was exposed. Other experimental results also revealed that the agarose molecules and crystalline regions were magnetically oriented. It was concluded that the cause of these anisotropic changes in the optical and physical properties of the gel was the magnetic orientation of the crystalline regions.
Because the crystalline regions, which have an anisotropic storage modulus, are ordered magnetically, an anisotropic change in elasticity should be observed in gels formed under magnetic field. Significant information should be obtained from the linkage density of the network structure of the gel. In this study, the elastic properties of magnetically ordered agarose gel were measured in order to elucidate its magnetically induced network structure.
Experimental
The raw material used was agarose type-L with a gel strength of 4500 kg m−2, supplied by Wako Chemicals. It is a special-grade material used in electrophoresis. Agarose powder and distilled water were mixed at concentrations of up to 5.0 wt.% and stirred at room temperature. The thermal cycle, comprised of a heating and a cooling period, was repeated 5 times to ensure homogenization before measurement. The gel-formation temperature was controlled by siphoning temperature-controlled water through the water jacket set in the bore of the magnet. Superconducting magnets (JMTD-10T100NC-mkII and JMTD-13T100EF3, JASTEC) were used to apply strong magnetic fields. The inhomogeneity of the fields in the gelation space was less than 3% within a distance of ± 25 mm from the center of the magnets. The sample gels were prepared in a polycarbonate case at a constant cooling rate of −0.37 K min−1 during the gelation process, under zero or strong magnetic fields of up to 13 T.
In general, the viscoelastic property of gels is measured using a rheometer or ultrasonic wave propagation [Citation7, Citation8]. We adopted the latter method, taking account of the in situ measurement in the magnet bore in the near future. Our method has some advantages: the size of the measurement system is small to be easily set in the magnet bore, nondestructive measurement is repeatable, and it is easy to control the sample temperature. The viscoelastic property is expressed by the complex modulus m=m′+m″. The real part, m′, is the storage modulus, and it refers to the elasticity, i.e. the hardness. The imaginary part, m″, is the loss modulus or viscosity.
The elasticity was investigated by measuring the velocity of one 3.2 MHz ultrasonic wave cycle at a constant temperature of 10.0 or 12.0 °C. The ultrasonic wave propagated through the gel, and the first signal was detected directly at the hydrophone. The wave was reflected twice by the inner walls of the sample case, and the second signal was detected after a delay. The elasticity was determined as
(1)
where ρ is the density of the gel, c is the sonic velocity (calculated for a gel thickness of d=10 mm) and t is the delay time between the first and second signals, typically 13 s. The sonic velocity was measured through the gel along the direction perpendicular and parallel to the magnetic flux to which the gel was exposed.
Results and discussion
Random and ordered gels were prepared in zero magnetic field and in a magnetic field of 13 T, respectively. The elasticity was measured as a function of the agarose concentration, as shown in figure . The elasticity of the random gel was proportional to the agarose concentration. This linear relationship suggests that the linkage density is proportional to the gel concentration. The same tendency was recognized in all of the gels examined. In the case of the ordered gels, changes in elasticity were observed depending on the direction of the magnetic field, as expected. The elasticity increased along the direction perpendicular to the magnetic field, while it decreased along the direction parallel to the magnetic field. It is noteworthy that the elasticity of the random gels took an intermediate hardness value between the anisotropic values of the ordered gels. The experimental results suggest that the linkage density was negligibly influenced, on the whole, by the exposure to the strong magnetic field, but that the structure of the gel changed to an anisotropic linkage structure.
Figure 1 Storage modulus, m′, of agarose gel at 12.0 °C as a function of concentration. The solid and broken curves denote the m′ values for random gel formed in 0 T and ordered gel formed in 13 T, respectively. The m′ values were measured along the directions perpendicular (open circle) and parallel (closed square) to the magnetic field.
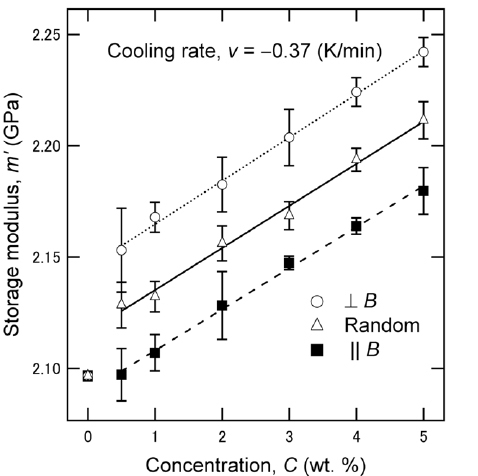
Figure describes the dependence of the elasticity on the strength of the magnetic field. The elasticity along the direction perpendicular to the magnetic field, m′⊥, increased by 0.8% with increasing magnetic field. However, it became saturated at around 6 T. Meanwhile, the elasticity along the direction parallel to the field, m′||, decreased by −0.5% with decreasing magnetic field. It became saturated at around 4 T. It was found that the anisotropic elasticity increased with increasing magnetic field and became saturated at 4–6 T. This saturation tendency was in agreement with the result of the birefringence measurement [Citation2]. Therefore, it is considered that the change in elasticity correlates with the molecular ordering induced by strong magnetic fields. The average elasticity, m′av, was evaluated using the following equation:
Figure 2 The storage modulus, m′, of 2 wt.% agarose gel at 10.0 °C as a function of the magnetic field. The m′ values were measured along the directions perpendicular (open circle) and parallel (closed square) to the magnetic field. The open triangles represent the average storage moduli.
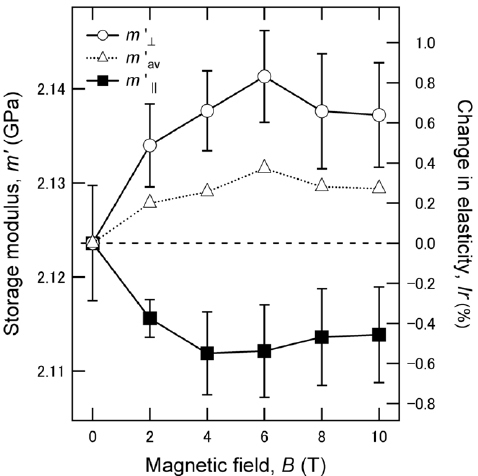
Conclusion
The elasticity of agarose gel was investigated using ultrasonic wave propagation. Gel formed in a strong magnetic field showed an anisotropic change in its elasticity. The increased and decreased in the elasticity were observed along the directions perpendicular and parallel to the magnetic flux, respectively. The anisotropy between them increased with increasing magnetic field. The magnetic-field-induced change in elasticity is thought to correlate with the gel structure, particularly the alignment of the domains.
Acknowledgments
This work was partially supported by a Grant-in-Aid for Scientific Research for Priority Areas (No 15085204, Area 767) from MEXT of Japan and the by JSPS Asian Core Program, ‘Construction of the World Center for Electromagnetic Processing of Materials’.
References
- ArnottSFulmerAScottW E 1974 J. Mol. Biol. 90 269 http://dx.doi.org/10.1016/0022-2836(74)90372-6
- HayashiAKinoshitaKYasuedaA 1980 Polymer J. 12 447 http://dx.doi.org/10.1295/polymj.12.447
- MatsumotoYYamamotoIYamaguchiMShimazuYIshikawaF 1997 Japan. J. Appl. Phys. 36/10B L1397 http://dx.doi.org/10.1143/JJAP.36.L1397
- YamamotoIMatsumotoYYamaguchiMShimazuYIshikawaF 1998 Physica B 246–247 408 http://dx.doi.org/10.1016/S0921-4526(97)00948-4
- YamamotoISaitoSMakinoTYamaguchiMTakamasuT 2006 Sci. Technol. Adv. Mater. 7 322 http://dx.doi.org/10.1016/j.stam.2006.02.003
- YamamotoI YamaguchiM 2004 Proc. Joint Symp. on Magneto-Science 2004 106
- RossK APyrak-NolteL J CampanellaO H 2006 Food Hydrocolloids 20 79 http://dx.doi.org/10.1016/j.foodhyd.2005.01.007
- ToubalMNongaillardB RadziszewskiEBoulenguerP LangendorffV 2003 J Food Eng. 58 1 http://dx.doi.org/10.1016/S0260-8774(02)00325-4