Abstract
The corrosion resistance of any metallic material depends on the environment to which it is exposed. DMR-1700 steel is a material for structural applications that has been recently developed at Defence Metallurgical Research Laboratory by changing the chemistry of alloying elements. Therefore, a detailed understanding of its corrosion characteristics under different environmental conditions is essential. In the present paper, we report the results of a systematic corrosion study that was carried out on the new steel to determine the effect of the environment on the protective nature of the oxide scale that forms on its surface under different environmental conditions. Furthermore, the oxide scale as well as the resistance to pitting and crevice corrosion were studied in various environments. The surface morphologies of the corroded steels were observed under a scanning electron microscope (SEM) to determine the nature of the corrosion. On the basis of studies by different techniques, DMR-1700 steel is recommended for the manufacture of components used in various systems in conjunction with the application of an appropriate protective coating to improve its resistivity to corrosion.
Introduction
Iron and steels are the most versatile, least expensive and most widely used materials for the construction of many engineering systems. They are unequaled in their range of mechanical and physical properties with which they can be endowed by alloying and heat treatment. Their main disadvantage is that they have poor corrosion resistance in even relatively mild environments unlike stainless steels. Corrosion can reduce the load-carrying capacity of a component either by reducing its size or by pitting. Pitting not only reduces the effective cross section in the pitted region but also introduce stress raisers, which initiate cracks. Any technique that reduces or eliminates corrosion will extend the life of a component and increase its reliability. In addition to corrosion prevention methods, it is important to design and select materials for improving the overall corrosion performance of components. The three interrelated factors that drive the selection of materials for corrosion control are the corrosivity of the environment, the corrosion resistance of the material and the acceptable rate of attack.
Corrosion failures during service are likely if suitable materials with appropriate coatings are not selected for the fabrication of components of critical systems [Citation1–6]. Corrosion significantly affects the efficiency of systems [Citation2–5]. Failures can be minimized or reduced significantly by controlling corrosion. The possible methods of controlling corrosion are the application of coatings, linings, metal cladding and corrosion inhibitors, and alloying [Citation7–10]. Among these, the application of suitable coatings appears to be a promising method of corrosion control in terms of cost effectiveness and service life. DMR-1700 is a recently developed ultrahigh-strength low-alloy steel with improved mechanical properties compared with other aerospace-grade low-alloy steels [Citation11]. However, its corrosion characteristics have not been investigated in detail, although some corrosion studies have been carried out [Citation12–15].
In the present investigation, the effect of the environment on the corrosion characteristics of DMR-1700 steel is studied in detail, the properties of the steel under various environmental conditions are compared and the degradation mechanisms involved are considered. On the basis of the results of the investigation, we recommend the use of the material with a suitable protective coating for the fabrication of components for various applications. In the study, the surface morphologies of the corroded steels in different environments were observed using a scanning electron microscope (SEM) to determine the nature of corrosion. We also successfully developed a high-performance coating for DMR-1700 steel to enhance its resistance against corrosion. The developed coating is discussed, and its application is recommended for effective protection against corrosion. The use of such an advanced material with a high-performance coating will help enhance the efficiency of systems by reducing failures during service.
Experimental
The chemical composition of the recently developed DMR-1700 steel studied in the present investigation is shown in table 1.
Chemical composition of different steels (wt.%).
Disc specimens of 14 mm diameter and 3 mm height were machined from steel rods and then polished to an 800 grit surface finish. Subsequently, the samples were cleaned with distilled water and acetone. All the corrosion studies were carried out in aerated 3.5% NaCl, 0.5 M Na2SO4 and 0.5 M H2SO4 solutions at room temperature; hereafter referred as marine, industrial and chemical environments, respectively. A standard three-electrode cell consisting of the steel as a working electrode, a saturated calomel reference electrode (SCE) and a graphite counter electrode was used to perform the polarization experiments. A magnetic stirrer was used to stir the solutions. The specimens were fixed in a specimen holder and were exposed to the testing environment in such a way that the exposed surface area of each specimen was 1 cm2. A computer-controlled EG & G Potentiostat (Model 273A) was used with corrosion analysis software supplied by Princeton Applied Research Corporation (PARC), USA to record the potentiodynamic impedance, the cyclic polarization and the isolated electrode potential as a function of time for the steel in different environments. AC impedance plots were recorded using a 5210 lock-in amplifier, a Model 273A potentiostat, Model 398 impedance software and a computer. Subsequently, an SEM was used to determine the nature of corrosion in the various environments.
Different compositions of epoxy polyamines followed by a polyurethane (PU) top coating and a 96% zinc-rich coating were applied on DMR-1700 steel specimens. Initially the machined specimens were polished to a 600 grit surface finish, cleaned with water and degreased with alcohol followed by acetone. Various coatings were applied immediately after sandblasting (SA 2 1/2) using an airless spray gun. A PU top coating was applied on different epoxy-based primers [Chemguard (CG), Grandpoly (GP), Koroguard (KG), Multiguard (MG)] and on a single layered 96% zinc-rich coating [zinga (ZG)] after 24 h of drying (figure ). A three-layer 96% zinc-rich coating was also applied on a separate DMR-1700 steel specimen. The second layer was applied after allowing the first layer to dry for 1 h, and a third layer was subsequently applied after drying for another hour. AC impedance spectroscopy was used to test all the coatings including the 96% zinc rich coating applied on DMR-1700 steels (100 μ m) after exposure to a marine environment for a specific number of days.
Results
Potential-time characteristics
The variation of isolated electrode potential as a function of time for DMR-1700 steel in different environments at room temperature is shown in figure . The potential initially rapidly decreases in marine and industrial environments and then slowly decreases. This indicates that the steel is initially unable to form a protective oxide scale but after exposure for a few seconds, a stable oxide scale is formed on its surface; however, it is not protective under marine and industrial environmental conditions. In contrast, the potential in the chemical environment initially decreased and then remained constant. This is attributed to the formation of an initially non-protective oxide scale over its surface that then stabilized. This type of behaviour has been reported for various materials on which no protective oxide scale forms under various environmental conditions [Citation16–21]. Generally, an increase in potential indicates the formation of a passivation film and a steady potential indicates that the film remains intact and protective. A decrease in potential indicates breaks in the film, the dissolution of the film or no film formation. To obtain good corrosion resistance, the potential should increase as a function of time under the chosen environmental conditions. Thus, the present results clearly show that effective measures must be carried out to exploit DMR-1700 steel for a variety of applications.
Potentiodynamic polarization studies
The corrosion potential (Ecorr), current density (Icorr), and corrosion rate measured for the steel in various environments by the potentiodynamic polarization technique are presented in table 2.
Corrosion characteristics of DMR-1700 steel in different environments at room temperature.
The potentiodynamic polarization plots recorded for the steel in various environments are shown in figure . It is observed that the steel is not able to form passivation layer on its surface in the marine environment. However, a passivation layer is formed in industrial and chemical environments, although it is not stable over a long time; the passivating tendency disappears in both environments after some time. In essence, the polarization behaviour of the steel is the same in the industrial and chemical environments and different in the marine environment.
Figure 3 Potentiodynamic polarization curves of the steel in various environments at room temperature.
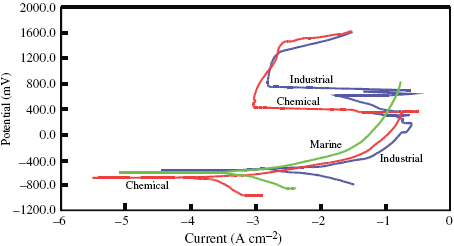
The values of Ecorr shifted to the more electronegative side in the industrial environment compared with the marine environment, whereas in the chemical environment, Ecorr shifted to the more electropositive side. However, it is not possible to interpret on the basis of Ecorr data. Icorr is lowest in the marine environment, moderate in the industrial environment and a high Icorr is observed in the chemical environment. The value of Icorr is directly proportional to the corrosivity of the environment in which the steel is tested. Therefore, the chemical environment is the most corrosive followed by the industrial and marine environments, which is clearly reflected in the corrosion rates.
The corrosion rate of DMR-1700 is less in the marine environment than in the industrial environment. The difference is about 50 mpy. However, the corrosion rate is much higher in the chemical environment. The corrosion rate is minimum in the marine environment and increases moderately in the industrial environment. The corrosion rate of steel is nearly 25 times higher in the chemical environment than in the marine environment. The chemical environment is highly corrosive and causes the steel to degrade at a significantly higher rate. In essence, the effect of the environment or its corrosiveness on DMR-1700 steel is as follows:
Pitting corrosion characteristics
The cyclic polarization curves of the steel recorded in different environments at room temperature are shown in figure . The reverse scan of the steel had a lower potential than the forward scan and the scanning results did not intersect for the marine and industrial environments, indicating that the steel is susceptible to pitting and crevice corrosion in both the environments. The area of the loop between the forward and reverse scans is greater for the marine environment than for the industrial environment. DMR-1700 may, therefore, be more susceptible to pitting and crevice corrosion in the marine environment. For the chemical environment, the reverse scan of the steel coincided with the forward scan and no loop was formed. This indicates that the chemical environment does not cause the steel to undergo either pitting or crevice corrosion. Some other type of corrosion must cause the high corrosion rate observed in the chemical environment. Thus, the results clearly reveal that the steel is susceptible to both pitting and crevice corrosion due to the fact that the marine and industrial environments contain sodium chloride and sodium sulphate, respectively. However, the susceptibility to pit and crevice formation varies from one environment to another, as mentioned above. Among the three environments, the marine environment causes the steel to undergo the greatest amount of pitting and crevice corrosion, whereas in the industrial environment, the steel undergoes least pitting and crevice corrosion, as evidenced from the low area of the loop. In essence, the steel undergoes pitting and crevice corrosion in the marine and industrial environments. Therefore, it is essential to apply a high-performance coating that increases resistance to pitting and crevice corrosion and enhances the service life.
Surface morphology studies
The surface morphologies of the steel after corrosion in different environments at room temperature are shown in figure . Pits can be seen on the surface of specimens corroded in the marine and industrial environments indicating that the steel undergoes pitting corrosion in these environments. However, the degree of susceptibility to pit formation is different in marine and industrial environments as mentioned earlier. Pitting is more extensive and localized in the marine environment than the industrial environment (figure ). In the case of specimens corroded in the chemical environment, the corrosion is uniform across the surface indicating that uniform corrosion is the mode of degradation in this environment. These results are in good agreement with the cyclic polarization data and suggest the necessity of applying high-performance corrosion-resistant coatings to eliminate failures during service because pitting and crevice corrosion are highly critical in all systems.
AC impedance studies
Corrosion assessment.
Impedance measurements are one of the most useful and informative methods of corrosion assessment. The response of a corroded specimen to the application of a small-amplitude signal depends on the frequency of the signal. The magnitude and phase shift at each frequency was noted and the response of the corroded cell was expressed as a combination of resistive, capacitive and inductive components. The impedance behaviour of a specimen can be expressed either in Bode plots of impedance Z as a function of frequency or in Nyquist plots of Z''(ω) as a function of Z'(ω), where ω=2πf. The difference between the low and high frequencies gives the charge transfer resistance, which is inversely proportional to the corrosion rate [Citation16–21]. AC impedance plots recorded for the steel in different environments at room temperature are shown in figure . The resistance of the film that forms on the surface of DMR-1700 steel is greatest in the marine environment followed by the industrial environment. The lowest resistance is observed for the steel in the chemical environment. The charge transfer resistance measured from the Nyquist plots (figure ) confirms the high corrosion rate of DMR 1700 steel in the chemical environment and the low corrosion rate in the marine environment. The phase variation as a function of frequency (figure ) further confirms the high corrosion rate in the chemical environment and the low rate in the marine and industrial environments.
Development of high-performance protective coatings.
AC impedance spectroscopy is an extremely useful technique for developing a variety of coatings for different applications by allowing their evaluation in the laboratory. The data is useful for quantitatively estimating the in situ inhomogenity of coatings and their protection ability. Coatings can be tested periodically (over time) to evaluate the long-term performance of a particular coating for a specific application. The impedance behavior of a 96% zinc-rich coating applied on DMR-1700 steel over a period of one year is shown in figure . As can be seen, the coating applied on the steel provided very good resistance even after 376 days of direct exposure to the marine environment. Other coatings (different compositions of epoxy primers and PU) applied on the steel did not exhibit good resistance. Hence, a 100-μm-thick 96% zinc-rich coating applied after sandblasting using a spray gun enhances the life of DMR-1700 steel significantly under marine environmental conditions. The coating is also expected to provide good protection for the steel in other environments. However, further research is needed to test the coating in other environments.
Discussion
The isolated electrode potential as a function of time for the steel in different environments revealed that the low-alloy steel DMR-1700 is not able to form a protective oxide scale over its surface. The results also suggest that the formation of a protective oxide scale depends on the environment to which the steel is exposed. The different corrosion rates observed in the various environments considered in the present investigation provide further evidence for this fact. The corrosion rate is high for the steel in the chemical environment and low in the marine environment as expected. This is due to the fact that the chloride ions present in the marine environment form the oxide scale on the surface of the steel causing damage to selected regions, thereby resulting in pitting. Similarly, the sulphur-containing compound does not allow the formation of a protective oxide scale and damages the formed oxide scale by reacting with it, thus resulting in enhanced corrosion attack. Furthermore, the low-alloy steel exhibits a high corrosion rate as expected, due to the fact that it contains very low amounts of Cr, Ni and Mo. The newly developed DMR-1700 steel contains only 1% chromium, 3% nickel and <1% molybdenum. It is known that an appropriate amount of chromium is essential for the formation of a passivation film on steel [Citation22]. Other elements can influence the effectiveness of chromium in forming or maintaining the film.
As mentioned earlier, the new steel is not able to form a protective oxide layer as it contains a small amount of Cr. Furthermore, the environments under which the steel was tested were highly corrosive. Therefore, it is very easy for the corrosive species of the environment to destroy or damage the thin layer, resulting in considerable corrosion. Alternatively, the corrosive environments do not allow the formation of a protective oxide layer on the surface of steel due to the fact that the corrosive species such as chloride and sulphur ions interfere with its formation, thus causing the steel to degrade at a faster rate.
The cyclic polarization data show that the low-alloy steel is susceptible to pitting and crevice corrosion under marine and industrial environmental conditions. This form of corrosion is highly critical and causes failures without revealing much corrosion on the surface. The observation of a lower corrosion rate in both the marine and industrial environments supports the present investigations. Generally, alloys resistant to pitting are also resistant to crevice corrosion. Increased amounts of chromium, nickel, molybdenum and nitrogen in steels increase their resistance to both pitting and crevice corrosion [Citation23]. Chlorine is the most common ion for the initiation of pitting, and sulphur-containing compounds also cause pitting, as evidenced from the present investigations although its effect is less. Once a pit is formed, it effectively becomes a crevice; the local chemical environment is substantially more corrosive than the bulk environment. Furthermore, the area of a pit is very small; thereby, the pit growth is very fast and the ultimate result is the formation of holes in the steel within a short time upon exposure to marine and industrial environments. The stability of a passivation film with respect to resistance to pitting initiation is determined primarily by the amounts of chromium and molybdenum. Minor alloying elements can also have an important effect by influencing the amount and type of inclusions in the steel, which can act as pitting sites. The present steel contains only small amounts of Cr, Ni and Mo as mentioned above and hence pitting was expected and the present results were confirmed from SEM images. However, in the chemical environment the steel corrodes uniformly. This is attributed to the dissolution of the oxide scale in the chemical environment, which is in agreement with the high corrosion rate in the chemical environment.
Corrosion rates as well as pitting studies clearly reveal the effect of the environment on the corrosion characteristics of the newly developed advanced steel. Pitting is the primary mode of degradation in the marine and industrial environments. The low corrosion rate is attributed to the pitting corrosion. However, pitting corrosion is highly critical and it is natural that the components will be exposed to these environments during service conditions. It is therefore essential to select a material that will not be subjected to pitting and the formation of crevice or to enhance its pitting resistance by suitable techniques. The chemical environment produced different corrosion characteristics of the steel, causing it to degrade uniformly at a high rate. That means that the chemical environment causes the mechanism of degradation to change from pitting to general corrosion. In essence, the environment has a considerable effect on the corrosion characteristics of the advanced steel. Furthermore, the results clearly suggest the necessity of carrying out protective measures to enhance the life of the steel by avoiding failures during service under different environmental conditions.
Surface engineering approaches alleviate pitting and crevice corrosion by tailoring the surface of the steel, which enhances the surface properties while keeping the mechanical properties intact. In fact, surface engineering techniques are effective and economically viable and are widely used. There are a variety of surface engineering techniques and materials available. Once the most effective technique and a suitable coating materials are identified by extensive investigations in the laboratory as well as in the field, it will be ensured that the steel will have a long lifetime and excellent mechanical properties. In fact, the usefulness of surface engineering techniques has been proven in a number of cases [Citation24–29]. A high-performance coating and a surface engineering technique have been successfully identified for DMR-1700 steel, which enhance its lifetime significantly as evidenced from the present results (figure ).
From the above observations, it is clear that the newly developed DMR-1700 ultrahigh-strength low-alloy steel undergoes more pitting and crevice corrosion in marine and industrial environments and greater general corrosion in chemical environments. However, a high-performance coating has been successfully developed that can enhance its life significantly. An alternative three-layer coating for the protection of DMR-1700 steel is under long-term evaluation [Citation30]. Furthermore, the excellent mechanical properties of DMR-1700 steel are an additional advantage over other widely used steels. EN 24 low-alloy steel with a cadmium coating has been used for aerospace applications. However, it can be replaced with the newly developed steel for better efficiency and enhanced lifetime as failures of EN 24 have been reported due to hydrogen cracking. Hydrogen pickup takes place during the electrodeposition of cadmium. Failures occur rapidly during service if dehydrogenation is not performed after electroplating. Therefore, the use of DMR-1700 with a 96% zinc-rich coating which can be coated using a spray gun, thus eliminating hydrogen pickup and absorption is recommended for the fabrication of components for aerospace and other industrial applications. The developed coating is expected to provide good protection to steel components that are to be used in the chemical industry. However, further research is needed to confirm the suitability of this coating under chemical environments. The development of alternative coatings is another ongoing area of research, which will help further enhance the efficiency of systems further by improving their lifetime.
Conclusions
The effect of the environment on the corrosion characteristics of the newly developed ultrahigh-strength low-alloy steel DMR-1700 has been investigated systematically. | |||||
The steel is not able to form a protective oxide scale on its surface. | |||||
DMR-1700 exhibits a low corrosion rate in marine and industrial environments and a high corrosion rate in chemical environments. | |||||
The low-alloy steel is vulnerable to pitting and crevice corrosion under marine and industrial environmental conditions and general corrosion in chemical environments. | |||||
A high-performance coating has been successfully developed for DMR-1700 steel to protect it against corrosion. | |||||
Advanced DMR-1700 steel with a 96% zinc-rich coating is suitable for the fabrication of components for aerospace as well as for other industrial applications. |
Acknowledgments
The Defence Research and Development Organization is gratefully acknowledged for providing financial assistance. We thank Professor P Rama Rao, ARCI and Dr D Banerjee, Chief Controller (R&D) (DRDO) for their support and constant encouragement.
References
- GurrappaI 2001 Surf. Coat. Technol. 139 272 http://dx.doi.org/10.1016/S0257-8972(00)01156-7
- StarosvetskyJStarosvetskyDArmonR 2007 Eng. Failure Anal. 14 1500 http://dx.doi.org/10.1016/j.engfailanal.2007.01.020
- YuZ WXuX L 2006 Eng. Failure Anal. 13 673 http://dx.doi.org/10.1016/j.engfailanal.2004.10.018
- WullschlegerLRuttiT FZgraggenMPiskotyG 2007 Eng. Failure Anal. 14 1512 http://dx.doi.org/10.1016/j.engfailanal.2006.12.006
- EbaraE 2007 Mater. Sci. Eng. A 468–470 109 http://dx.doi.org/10.1016/j.msea.2006.09.128
- JonesD A 1996 Principles and Prevention of Corrosion 2nd edn Upper Saddle River NJ:Prentice Hall p 199
- SeberryD WReytonG RClarkW S 1984 Corrosion 40 250
- WagnerG H 1989 Werkstoffe und Korrosion 40 703 http://dx.doi.org/10.1002/maco.19890401203
- SeverynG M 1988 J Protective Coat. Linings 2 58
- GoyWKaempflerM 1992 Werkstoffe und Korrosion 43 270 http://dx.doi.org/10.1002/maco.19920430604
- MalakondaiahGSrinivasMRaoP R 1997 Prog. Mater. Sci. 42 209 http://dx.doi.org/10.1016/S0079-6425(97)00016-9
- GurrappaIMalakondaiahG 2005 Mater. Sci. Eng. A 391 235 http://dx.doi.org/10.1016/j.msea.2004.08.079
- GurrappaIReddyC V K 2007 J. Mater. Process. Technol. 182 195 http://dx.doi.org/10.1016/j.jmatprotec.2006.07.031
- GurrappaIMalakondaiahG 2006 Met. Mater. Trans. A 37 3039 http://dx.doi.org/10.1007/s11661-006-0185-9
- KamatS VMalakondaiahGRamuSSharmaRSekharanV GRaoA S 2000 DMRL Technical Report 2000289 December
- GurrappaI 2001 Corros. Prev. Control 48 116
- GurrappaI 2002 Mater. Charact. 48 63 http://dx.doi.org/10.1016/S1044-5803(02)00248-6
- GurrappaI 2002 J. Alloys Compound. 339 241 http://dx.doi.org/10.1016/S0925-8388(01)02005-9
- GurrappaI 2005 J. Alloys Compound. 360 190 http://dx.doi.org/10.1016/j.jallcom.2004.05.079
- GurrappaI 2002 Mater. Charact. 49 73 http://dx.doi.org/10.1016/S1044-5803(02)00320-0
- GurrappaI 2002 J. Mater. Chem. Phys. 35 719
- GreenS J 1987 Metals Handbook, Corrosion volume 13 9th edn Metals Park OH:ASM International p 550
- TuckerR CJr 2004 Adv. Mater. Process. 162 25
- GurrappaI 2001 Surf. Coat. Technol. 139 272 http://dx.doi.org/10.1016/S0257-8972(00)01156-7
- GurrappaI 2003 Mater. Sci. Eng. A 356 372 http://dx.doi.org/10.1016/S0921-5093(03)00150-3
- MckeeD WLuthraK L 1993 Surf. Coat. Technol. 56 109 http://dx.doi.org/10.1016/0257-8972(93)90014-F
- GurrappaI 2003 Oxid. Met. 59 321 http://dx.doi.org/10.1023/A:1023044111767
- SugamaTWebsterRReamsWGawlikK 2000 J. Mater. Sci. 35 2145 http://dx.doi.org/10.1023/A:1004754204893
- OhK TParkY S 1998 Surf. Coat. Technol. 110 4 http://dx.doi.org/10.1016/S0257-8972(98)00537-4
- KamatS VMalakondaiahGRamuSSharmaRSekharanV GRaoA S unpublished results