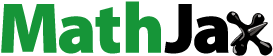
Abstract
Background
The aim was to profile transient accommodative axial length changes from early adulthood to advanced presbyopia and to determine whether any differences exist between the responses of myopic and emmetropic individuals.
Methods
Ocular biometry was measured by the LenStar biometer (Haag‐Streit, Switzerland) in response to zero, 3.00 and 4.50 D accommodative stimuli in 35 emmetropes and 37 myopes, aged 18 to 60 years. All results were corrected to reduce errors arising from the increase in crystalline lens thickness with accommodation. Accommodative responses were measured sequentially by the WAM 5500 Auto Ref/Keratometer (Grand Seiko, Hiroshima, Japan).
Results
Axial length increased significantly with accommodation (p < 0.001), with a mean corrected increase in axial length of 2 ± 18 µm and 8 ± 16 µm observed at 3.00 and 4.50 D, respectively. The magnitude of accommodative change in axial length was not dependent on refractive error classification (p = 0.959); however, a significant reduction in the magnitude and variance of axial length change was evident after 43 to 44 years of age (p < 0.002).
Conclusion
The negative association between transient increase in axial length and age, in combination with reduced variance of data after age 43 to 44 years, is consistent with a significant increase in posterior ocular rigidity, which may be influential in the development of presbyopia.
The amplitude of accommodation decreases with age, from approximately 15 D in early infancy to 1.00 D at 60 years of age,Citation1864. when presbyopia is well established. Significant age‐related structural changes in the accommodative apparatus (for example, increased lenticular stiffness) are thought to be responsible for the attenuation of the amplitude of accommodation and therefore, the development of presbyopia.Citation1995 While age‐related accommodative changes within the anterior segment have been investigated (for example, ciliary muscle morphology and mobility),Citation1997 accommodative changes within the posterior segment with respect to age have yet to be reported.
Indeed, a significant transient elongation of axial length during short periods of accommodation has been observed in young adults.Citation1998 It has been hypothesised that this axial length elongation during accommodation may be produced by the force applied to the equatorial choroid by ciliary muscle contraction, thus necessitating posterior pole elongation to maintain a constant ocular volume.Citation1998 In support of this theory, Croft and colleaguesCitation2013 observed the equatorial choroid and retina moved centripetally during Edinger‐Westphal stimulated accommodation in rhesus monkeys. Due to the persistence of ciliary muscle contractility into advanced presbyopia,Citation2011 it is feasible that transient increase in axial length may also be present in presbyopic individuals; however, an in vivo anterior scleral studyCitation2005 and in vitro posterior choroidalCitation1991 and scleralCitation1988 studies have reported ocular stiffness increases with age. Van AlphenCitation1961 suggested the ciliary muscle and choroid behave as a single elasto‐muscular sheet around the eye, moulding the sclera to accommodate ciliary muscle tonus and therefore, it is conceivable that age‐related increases in ocular stiffness may mute transient axial length elongation during accommodation. Nevertheless, the relocation of the anterior uveal tract after cataract surgery to a more youthful position in humans suggests the choroid may retain its elasticity with age,Citation2010 despite the sclera and choroid becoming thickerCitation2004 and thinnerCitation2010 with age, respectively. Furthermore, evidence reported by Hollins (cited by AtchisonCitation1995) of transient axial length elongation in one advanced presbyopic patient during near vision also suggests that accommodation‐induced transient axial length elongation may persist into advanced presbyopia.
Additionally, it has been hypothesised that high levels of transient axial length elongation during accommodation may precipitate a myopic shift in refraction in susceptible eyes.Citation2006 Therefore transient axial length elongation during accommodation is one potential factor responsible for the connection between high levels of near work and the onset and progression of myopia in both childrenCitation2002 and adults.Citation1994 Mallen, Kashyap and HampsonCitation2006 hypothesised that myopic eyes may be more amenable to transient axial length elongation during accommodation due to reduced ocular rigidity (present due to the stretched, elongated eye characteristic of myopia). Yet, despite reduced posterior choroidalCitation2009 and scleralCitation2006 thickness in myopic eyes compared to emmetropic eyes, it remains unclear whether a correlation exists between in vivo anterior ocular rigidity and myopia.Citation2013 Moreover, previous research in young adults has produced contradictory results; Drexler and colleaguesCitation1998 reported the magnitude of axial length elongation was larger in emmetropic eyes than myopic eyes, whereas Mallen, Kashyap and HampsonCitation2006 reported greater elongation in myopic eyes and Read and colleaguesCitation2010 found no difference in ocular axial elongation between myopes and emmetropes during accommodation.
The aim of this study was to profile accommodative changes in axial length from early adulthood to advanced presbyopia and to determine whether any differences exist between the responses of myopic and emmetropic individuals.
METHODS
The study was approved by the Aston University Research Ethics Committee and was conducted in accordance with the tenets of the Declaration of Helsinki. Written informed consent was obtained from all the participants after an explanation of the nature and possible consequences of the study. Participants were included in the study, if they were aged between 18 and 70 years; could achieve 0.0 logMAR visual acuity, were classified as emmetropic (spherical equivalent between −0.75 and +0.50 D) or myopic (spherical equivalent more myopic than −0.75 D) and had 0.75-DC or less of uncorrected astigmatism. All participants were screened to exclude those with a positive history of ocular or systemic disease or prescribed medications known to affect the accommodative response.
Sample size calculation
The calculated total sample size for repeated measures analysis of variance (ANOVA) testing (within and between interaction), including an effect size (f) of 0.25, an error probability (α) of 0.05 and required power (1 – β) of 0.80 for three measurements between the two groups, was 28 participants.Citation2007
Amplitude of accommodation
The right eye of all the myopic participants was fitted with a soft daily disposable spherical contact lens (Focus Dailies, nelfilcon A, 69 per cent water content; Ciba Vision, Duluth, Georgia, USA). The power of the contact lens was chosen following a subjective refraction. A minimum settling period of 10 minutes was permitted prior to over‐refraction to ensure the contact lens prescription fully corrected the ametropia. The subjective amplitude of accommodation of the right eye was measured using a Royal Air Force Rule (Richmond Products, New Mexico, USA) via the push‐up/pull‐down method.Citation1986 The left eye of each participant was occluded. Using their right eye, participants were asked to attempt maintaining clear focus of the high‐contrast N5 print, as it was slowly pushed toward them until the point where they first reported sustained blur (push‐up amplitude). Subsequently, the first point at which the N5 print became clear when moved away from the eyes (pull‐down amplitude) was measured. If a patient was unable to achieve the minimum amplitude of accommodation measureable (2.00 D), a +3.00 D full‐aperture trial lens was introduced in front of the right eye. The power of the lens was subsequently subtracted from the push‐up/pull‐down amplitude value obtained. Push‐up/pull‐down amplitude was measured three times and averaged to calculate the mean amplitude of accommodation for the right eye.
Accommodative stimulus response
Change in objective spherical equivalent refractive error during accommodation was measured by the binocular open‐field WAM‐5500 autorefractor (Grand Seiko Co. Ltd., Hiroshima, Japan),Citation2010b with an external fixation target (a Maltese cross) viewed through a +5.00 D Badal lens attached to the instrument. The fixation target was placed 20-cm, 8.0-cm and 2.0-cm away from the Badal lens to stimulate zero, 3.00 and 4.50 D of accommodation, respectively. Each participant was asked to focus on the centre of the Maltese cross as accurately as possibleCitation1994 and was exposed to the stimulus for 20 seconds prior to the acquisition of data.Citation1989 A one minute distance viewing break was permitted between accommodative levels. The presentation order of each accommodative level was randomised between participants. Three consecutive measurements of refraction were acquired at each accommodative level.
LenStar biometry
LenStar biometry was measured while stimulating accommodation with an external target (viewed through a pellicle beam splitter and a +10.00 D Badal lens). To provide zero, 3.00 and 4.50 D accommodative stimuli, the Badal lens system letter target was placed 10-cm, 7-cm and 5.5-cm away from the +10.00 Badal lens, respectively. The average of three repeat measures of anterior chamber depth (ACD), crystalline lens thickness (LT) and axial length (AXL) were recorded at the three accommodative levels (zero, 3.00 and 4.50 D). Corneal thickness (CT) was measured at zero dioptres only because it has been well‐established the human corneal thickness does not change during accommodation.Citation1998 Anterior segment length (ASL = CT + ACD + LT) and vitreous chamber depth (VCD = AXL – ASL) were calculated from these values. If the LenStar was unable to acquire lens thickness measurements for a participant, these data were excluded from the analysis.
Biometric error calculations
The LenStar uses an average ocular refractive index to convert an optical path length (OPL) to a geometric axial length.Citation2010 Therefore, to correct for the potential over‐estimation of axial length due to an increase in lens thickness with accommodation and age, the individual error (E) was calculated for each participant using Equations Equation to Equation
as proposed by Atchison and Smith.Citation2004 In line with previous publications,Citation2010 the relative proportions of the crystalline lens taken up by the anterior cortex, nucleus and posterior cortex during accommodation were kept constant because, despite the well‐established thickening of the nucleus during accommodation,Citation2003 the exact nature of the change in refractive index during accommodation is not fully understood.Citation2005 To compensate for age‐related changes in anterior cortex thickness (ACT), nucleus thickness (NT) and posterior cortex thickness (PCT), Equations Equation
, Equation
and Equation
were used to modify the segmentation of the crystalline lens according to age (in years), derived by Dubbelman and colleagues.Citation2003 Optical path length and the average refractive index of the eye (nav) were calculated using the refractive indices specified by Gullstrand's No. 1 (exact) eye with shell lens (Equations Equation
and Equation
).Citation2004 Equation Equation
was used to calculate the error (E), which is subtracted from the geometric axial length reported by the LenStar to provide corrected axial length values.
Statistical analysis
To assess whether biometry changed significantly with accommodation (within‐subject factor) and to determine its dependency on refractive error (between‐subject factor), a repeated measures ANOVA was performed (SPSS, SPSS Inc, Chicago, Illinois, USA). The relationship between biometry and age was determined by linear regression analysis.
To compare the variance of the data between the younger and older participants, a two‐tailed variance test was used, which accounts for differences in group sizes. The ANOVA residual mean square indices produced by individual linear regression analysis of the younger and older groups are divided to produce an F ratio. The significance of the difference in variance is determined by a two‐tailed F look‐up table, according to the degrees of freedom in each group.
RESULTS
Participants
Seventy‐two participants aged 18 to 60 years (median age 33.60-years) were recruited from an Aston University staff and student volunteer call. Participants were classified as emmetropic (spherical equivalent from −0.50 to +0.50 D) or myopic (spherical equivalent −0.75 D or greater) and all subjects had less than 0.75-DC astigmatism. Of the total cohort, 37 were myopic (−3.49 ± 1.87 DS) and 35 were emmetropic (−0.16 ± 0.32 DS). The onset of ametropia (established by the participant's recollection) in the majority of the myopic subjects was before the age of 15 years (68 per cent) and all myopes reported their myopia to be stable (defined as myopic progression less than 0.50 D in the last 12 months). The myopic and emmetropic groups were 65 and 54 per cent female, respectively. The myopic group contained 20 white European and 17 British Indian individuals and the emmetropic group contained 21 white European and 14 British Indian individuals.
The emmetropic and myopic groups were balanced for age (U = 607, p = 0.652, myopes median age 31.49-years, emmetropes median age 34.67-years) and right eye amplitude of accommodation (t = 0.588, p = 0.558, myopes 7.69 ± 3.37 D, emmetropes 7.26 ± 2.83 D). As expected, right eye amplitude of accommodation decreased significantly with age (r = 0.918, p < 0.001; Figure 2). Baseline uncorrected spherical equivalent was not significantly correlated with age in either group (myopes r = 0.063, p = 0.701; emmetropes r = 0.084, p = 0.621).
Ocular biometry
The disaccommodated vitreous chamber depth was not significantly correlated with age in either group (myopes r = 0.093, p = 0.584, emmetropes r = 0.277, p = 0.108); however, the disaccommodated anterior chamber depth was significantly smaller with increasing age (myopes r = 0.516, p = 0.001, emmetropes r = 0.347, p = 0.041) and lens thickness (myopes r = 0.707, p < 0.001, emmetropes r = 0.602, p < 0.001) and anterior segment length (myopes r = 0.417, p = 0.010, emmetropes r = 0.375, p = 0.027) were significantly larger with increasing age. Older emmetropic individuals had significantly longer axial lengths than younger emmetropic individuals (myopes r = 0.045, p = 0.790, emmetropes r = 0.437, p = 0.009).
Repeated measures ANOVA testing showed the decrease in anterior chamber depth (F = 95.994, p < 0.001) and the increase in lens thickness (F = 142.410, p < 0.001), anterior segment length (F = 8.239, p = 0.001), vitreous chamber depth (F = 5.955, p = 0.006) and axial length (F = 11.104, p < 0.001) was statistically significant during accommodation. No differences in the accommodative response emerged based on the grouping of ametropia (anterior chamber depth F = 0.507, p = 0.562; lens thickness F = 3.482, p = 0.053; anterior segment length F = 1.342, p = 0.263; vitreous chamber depth F = 1.330, p = 0.266; axial length F = 0.277, p = 0.730). As expected, anterior chamber depth (F = 7.653, p = 0.007), vitreous chamber depth (F = 68.321, p < 0.001) and axial length (F = 63.741, p < 0.001) values were consistently longer in myopic eyes when compared to emmetropic eyes.Citation1980 No significant differences emerged between myopic and emmetropic lens thickness (F = 0.285, p = 0.595) and anterior segment length (F = 2.340, p = 0.131). Considering the whole cohort, a non‐statistically significant, possible negative association emerged between the change in anterior chamber depth (r = 0.128, p = 0.285), lens thickness (r = 0.067, p = 0.577) and anterior segment length (r = 0.020, p = 0.093) with the change in axial length observed at 4.50 D.
The relationship between the accommodation‐induced axial length changes and age are illustrated in Figure 3. After visual inspection of the graphical data in Figure 3, the cohort was split into under 43 years (59 participants) and 43 years and older (13 participants), owing to the dramatic reduction in data spread after age 43 years. The difference in variance of the corrected axial length data between the groups was significant at the 3.00 D (F = 2.983, p < 0.020) and 4.50 D (F = 8.852, p < 0.002) accommodative levels. To validate the choice of 43 years as the fulcrum point, the spread of data was analysed in one‐year increments either side of age 43 years at 3.00 D (41-years F = 1.848, p > 0.100; 42 years F = 2.204, p < 0.100; 44 years F = 2.983, p < 0.020; 45 years F = 2.454, p < 0.050) and 4.50 D (41-years F = 2.736, p < 0.02; 42 years F = 5.055, p < 0.002; 44 years F = 8.852, p < 0.002; 45 years F = 8.312, p < 0.002). Between the ages of 43 to 44 years was therefore selected as GibsonCitation2008 reported that the posterior movement of the crystalline lens is negligible until the accommodative demand reaches approximately 2.00 D (eliciting an accommodative response of approximately 1.50 D). Therefore, only participants who demonstrated an accommodative response greater than 1.50 D to the 4.50 D accommodative target were included in calculations to determine the changes in ocular biometry per dioptre of accommodation exerted. The top age limit included in the biometric response per dioptre of accommodation measurements was consequently readjusted to 44 years; however, younger participants who failed to accommodate more than 1.50 D (Figure 2) were excluded, resulting in an amended cohort size of 56 participants. Despite a significant attenuation of the accommodative change in anterior chamber depth, lens thickness and axial length with age according to the magnitude of the accommodative stimulus, the changes per dioptre of accommodation exerted were not dependent on age (Table ). The decrease in anterior chamber depth per dioptre of accommodation exerted was 0.081 ± 0.053-mm/D, lens thickness increased by 0.109 ± 0.052-mm/D, anterior segment length increased by 0.028 ± 0.057-mm/D, vitreous chamber depth decreased by 0.025 ± 0.059-mm/D and axial length increased by 0.003 ± 0.006-mm/D.
Table 1. Dependency of accommodative changes in biometry on participant age (56 participants). Vitreous chamber depth (VCD) and axial length (AXL) values have been corrected for the increase in lens thickness (LT) with age and accommodation.
DISCUSSION
The present study is the first to document the effect of age on axial elongation during accommodation. In accordance with previous studies investigating accommodative changes in axial length in young adults,Citation1998 a statistically significant axial elongation with accommodation was observed in the current investigation, which was proportional to the magnitude of the accommodative stimulus. The change in ocular biometry per dioptre of accommodation exerted remained invariant between the ages of 18 to 44 years, as previously reported;Citation1997 however, beyond the age of 43 to 44 years, the change in axial length was negligible and the variance of data reduced significantly (Figure 3).
Due to the persistence of ciliary muscle contractility into advanced presbyopia,Citation2011 it is feasible that the reduced variance and magnitude of change in axial length with accommodation may signify in vivo posterior ocular rigidity increases significantly during the presbyopic years. Indeed, previous research has also relied on changes in axial length to determine changes in posterior ocular rigidity; Ebneter, Wagels and ZinkernagelCitation2009 reported ocular rigidity is higher in patients with glaucoma due to a smaller decrease in axial length following a pharmacologically induced (500-mg oral acetazolamide) fall in intraocular pressure when compared to a healthy control group. However, changes in axial length alone are not able to disentangle the relative contributions of each ocular layer to changes in rigidity and thickness, only to infer their cumulative effect. Currently, in vitro studies give the best indication of how the properties of the individual ocular layers may vary with age.
In support of the data presented here, an age‐related decrease in the elasticity of choroidal and scleral sections has been found by multiple in vitro studies.Citation2006 It is feasible that a reduction in the flexibility of the posterior pole with age may contribute to the development of presbyopia; reducing the ability of the choroid and posterior zonules to restore the accommodative apparatus to an disaccommodated position.Citation2008 Studies of in vitro isolated choroidal and scleral sections do not account for the impact of vascular rigidity, perfusion pressure, intraocular pressure, extra‐ocular muscle action, post‐mortem structural changes and how these factors may vary with age.Citation1988 Indeed, the choroidCitation2010 and its blood vesselsCitation2007 thin and the blood flow reduces with age,Citation2003 whereas the sclera becomes thicker with age.Citation2004 Unfortunately, it is not possible to measure posterior ocular rigidity in vivo directly and hitherto, the literature has not reached a consensus as to whether anterior eye rigidity increases with age.Citation2005
Furthermore, it is feasible the hypothesised increase in ocular shell rigidity with age is accompanied by a reduction in the capacity of the choroid to thin during accommodation, which has been suggested to contribute to the magnitude of axial elongation in young adults.Citation2012 The cumulative effect of these age‐related changes may be responsible for the abrupt loss of axial elongation during accommodation after 43 to 44 years of age. It is possible to extract choroidal thickness measurements from further analysis of the LenStar A‐scan peaks, which allows choroidal thickness to be simultaneously captured with corneal thickness, anterior chamber depth, lens thickness and axial length measurements;Citation2012 however, Woodman, Read and CollinsCitation2012 reported resolution was too poor to calculate choroidal thickness in 37 per cent of their cohort and the LenStar may be unable to acquire choroidal thickness measurements in older subjects due to reduced ocular media transparency.Citation2011 Therefore, future studies should use posterior eye optical coherence tomography to investigate accommodative choroidal changes, with the additional advantage of capturing data across a larger area of the choroid to profile the spatial change in choroidal thickness with accommodation.
Similar to the findings of Read and colleagues,Citation2010 the magnitude of the change in biometry in this study was not dependent on the classification of ametropia; however, Woodman, Read and CollinsCitation2012 found that the axial elongation of young myopes was significantly slower to return to baseline after cessation of a 30 minute 4.00 D near task. This could suggest that the magnitude of axial elongation during accommodation may be less significant in the mechanism of myopic progression than the time taken for the posterior shell to recoil after cessation of near vision. The delayed recoil may be as a result of the greater vitreous chamber volume in myopic eyes providing a greater resistance to immediate reformation. Alternatively, the choroid in myopic eyes is known to stretch and consequently thin with axial growthCitation2009 and therefore, conceivably its elasticity may also be compromised, which may mute the speed of the recoil response. Further research including longer sustained periods of accommodation and longitudinal follow‐up are required to clarify the role of choroidal thickness changes and posterior shell recoil in the development of myopia.
While the mean axial length increased during accommodation, a minority of participants demonstrated a reduction in uncorrected and corrected axial length (Figure 3). Perhaps an asymmetric elongation of the posterior pole, which is not centred on the fovea may be responsible for the apparent reduction of axial length during accommodation (Figure 4) due to the asymmetric ciliary muscle contractile response (greater temporally than nasallyCitation2011) and/or disaccommodated posterior pole asymmetry.Citation2004 Indeed, there is evidence of spatial distortions in vision accompanying marked accommodationCitation1973 consistent with asymmetric retinal stretch during accommodation. Further research to determine how the retinal contour changes during accommodation and where the point of maximum ocular elongation resides is indicated.
The current study is the first to investigate how accommodative changes in axial length attenuate with age. In conclusion, the change in ocular biometry per dioptre of accommodation exerted remained invariant between the ages of 18 to 44 years; however, after 43 to 44 years of age, the axial length change was negligible and the variance of data significantly reduced. The findings are consistent with a significant increase in ocular rigidity and attenuation of accommodative choroidal thinning with age, which may be influential in the development of presbyopia. Longitudinal research is required to investigate whether the mechanism of axial elongation during accommodation is associated with myopigenesis.
ACKNOWLEDGEMENTS
Dr Deborah Laughton received funds from the College of Optometrists, UK. We would like to thank Richard Armstrong for his assistance with statistical analysis.
REFERENCES
- Donders FC. Mechamisms of accommodation. In: Moore WD, ed. On the Anomalies of Accommodation and Refraction of the Eye. London, UK: The New Sydenham Society, 1864. pp 23–26.
- Duane A. Normal values of the accommodation at all ages. J Am Med Ass 1912; 59: 1010–1013.
- Atchison DA. Accommodation and presbyopia. Ophthalmic Physiol Opt 1995; 15: 255–272.
- Gilmartin B. The aetiology of presbyopia: a summary of the role of lenticular and extralenticular structures. Ophthalmic Physiol Opt 1995; 15: 431–437.
- Strenk SA, Strenk LM, Koretz JF. The mechanism of presbyopia. Prog Retin Eye Res 2005; 24: 379–393.
- Charman WN. The eye in focus: accommodation and presbyopia. Clin Exp Optom 2008; 91: 207–225.
- Atchison DA. New thinking about presbyopia. Clin Exp Optom 2008; 91: 205–206.
- Koretz JF, Cook CA, Kaufman PL. Accommodation and presbyopia in the human eye. Changes in the anterior segment and crystalline lens with focus. Invest Ophthalmol Vis Sci 1997; 38: 569–578.
- Sheppard AL, Davies LN. The effect of ageing on in vivo human ciliary muscle morphology and contractility. Invest Ophthalmol Vis Sci 2011; 52: 1809–1816.
- Drexler W, Findl O, Schmetterer L et al. Eye elongation during accommodation in humans: Differences between emmetropes and myopes. Invest Ophthalmol Vis Sci 1998; 39: 2140–2147.
- Mallen EAH, Kashyap P, Hampson KM. Transient axial length change during the accommodation response in young adults. Invest Ophthalmol Vis Sci 2006; 47: 1251–1254.
- Read SA, Collins MJ, Woodman EC et al. Axial length changes during accommodation in myopes and emmetropes. Optom Vis Sci 2010; 87: 656–662.
- Woodman EC, Read SA, Collins MJ et al. Axial elongation following prolonged near work in myopes and emmetropes. Br J Ophthalmol 2010; 95: 652–656.
- Woodman E, Read SA, Collins MJ. Axial length and choroidal thickness changes accompanying prolonged accommodation in myopes and emmetropes. Vision Res 2012; 72: 34–41.
- Croft MA, Nork TM, Mcdonald JP et al. Accommodative movements of the vitreous membrane, choroid and sclera in young and presbyopic human and nonhuman primate eyes. Invest Ophthalmol Vis Sci 2013; 54: 5049–5058.
- Strenk SA, Semmlow JL, Strenk LM et al. Age‐related changes in human ciliary muscle and lens: a magnetic resonance imaging study. Invest Ophthalmol Vis Sci 1999; 40: 1162–1169.
- Llikaris IG, Kymionis GD, Ginis HS et al. Ocular rigidity in living human eyes . Invest Ophthalmol Vis Sci 2005; 45: 409–414.
- van Alphen GW, Graebel WP. Elasticity of tissues involved in accommodation. Vision Res 1991; 31: 1417–1438.
- Ugarte M, Hussain AA, Marshall J. An experimental study of the elastic properties of the human Bruch's membrane‐choroid complex: relevance to ageing. Br J Ophthamol 2006; 90: 621–626.
- Friberg TR, Lace JW. A comparison of the elastic properties of human choroid and sclera. Exp Eye Res 1988; 47: 429–436.
- van Alphen G. On emmetropia and ametropia. Opt Acta (Lond) 1961; 142 (Suppl): 1–92.
- Strenk SA, Strenk LM, Guo S. Magnetic resonance imaging of the anteroposterior position and thickness of the aging, accommodating, phakic and pseudophakic ciliary muscle. J Cataract Refract Surg 2010; 36: 235–241.
- Watson PG, Young RD. Scleral structure, organisation and disease. A review. Exp Eye Res 2004; 78: 609–623.
- Manjunath V, Taha M, Fujimoto JG et al. Choroidal thickness in normal eyes measured using Cirrus HD optical coherence tomography. Am J Ophthalmol 2010; 150: 325–329.
- Mutti DO, Mitchell GL, Moeschberger ML et al. Parental myopia, near work, school achievement and children's refractive error. Invest Ophthalmol Vis Sci 2002; 43: 3633–3640.
- Saw SM, Chua WH, Hong CY et al. Nearwork in early‐onset myopia. Invest Ophthalmol Vis Sci 2002; 43: 332–339.
- Simensen B, Thorud OA. Adult‐onset myopia and occupation. Acta Ophthalmol 1994; 72: 469–471.
- Mcbrien NA, Adams DW. A longitudinal investigation of adult‐onset and adult‐progression of myopia in an occupational group. Invest Ophthalmol Vis Sci 1997; 38: 321–333.
- Maheshwari R, Sukul RR, Gupta Y et al. Accommodation: Its relation to refractive errors, amblyopia and biometric parameters. Nepal J Ophthalmol 2011; 3: 146–150.
- Ikuno Y, Tano Y. Retinal and choroidal biometry in highly myopic eyes with spectral‐domain optical coherence tomography . Invest Ophthalmol Vis Sci 2009; 50: 3876–3880.
- Rada JAS, Shelton S, Norton TT. The sclera and myopia. Exp Eye Res 2006; 82: 185–200.
- Dastiridou AI, Ginis H, Tsilimbaris M et al. Ocular rigidity, ocular pulse amplitude and pulsatile ocular blood flow: the effect of axial length. Invest Ophthalmol Vis Sci 2013; 54: 2087–2092.
- Schmid KL, Li RWH, Edwards MH et al. The expandability of the eye in childhood myopia. Curr Eye Res 2003; 26: 65–71.
- Patel H, Gilmartin B, Cubbidge RP et al. In vivo measurement of regional variation in anterior scleral resistance to Schiotz indentation. Ophthalmic Physiol Opt 2011; 31: 437–443.
- Faul F, Erdfelder E, Lang AG et al. G*Power 3: A flexible statistical power analysis program for the social, behavioral and biomedical sciences. Behav Res Meth 2007; 39: 175–191.
- Mcbrien NA, Millodot M. Amplitude of accommodation and refractive error. Invest Ophthalmol Vis Sci 1986; 27: 1187–1190.
- Wolffsohn JS, Sheppard AL, Vakani S et al. Accommodative amplitude required for sustained near work. Ophthalmic Physiol Opt 2011; 31: 480–486.
- Sheppard AL, Davies LN. Clinical evaluation of the Grand Seiko Auto Ref/Keratometer WAM‐5500. Ophthal Physiol Opt 2010b; 30: 143–151.
- Stark LR, Atchison DA. Subject instructions and methods of target presentation in accommodation research. Invest Ophthalmol Vis Sci 1994; 35: 528–537.
- Heron G, Winn B. Binocular accommodation reaction and response times for normal observers. Ophthalmic Physiol Opt 1989; 9: 176–183.
- Atchison DA, Smith G. Possible errors in determining axial length changes during accommodation with the IOLMaster. Optom Vis Sci 2004; 81: 282–285.
- Zhong J, Tao A, Xu Z et al. Whole eye axial biometry during accommodation using ultra‐long scan depth optical coherence tomography . Am J Ophthalmol 2014; 157: 1064–1069.
- Dubbelman M, van der Heijde GL, Weeber HA et al. Changes in the internal structure of the human crystalline lens with age and accommodation. Vision Res 2003; 43: 2363–2375.
- Dubbelman M, van der Heijde GL, Weeber HA. Change in shape of the aging human crystalline lens with accommodation. Vision Res 2005; 45: 117–132.
- Hermans EA, Dubbelman M, van der Heijde R et al. Equivalent refractive index of the human lens upon accommodative response. Optom Vis Sci 2008; 85: 1179–1184.
- Jones CE, Atchison DA, Pope JM. Changes in lens dimensions and refractive index with age and accommodation. Optom Vis Sci 2007; 84: 990–995.
- Sheppard AL, Davies LN. In vivo analysis of ciliary muscle morphologic changes with accommodation and axial ametropia. Invest Ophthalmol Vis Sci 2010; 51: 6882–6889.
- Fontana ST, Brubaker RF. Volume and depth of the anterior chamber in the normal aging human eye. Arch Ophthalmol 1980; 98: 1803–1808.
- Logan N, Davies L, Mallen EAH et al. Ametropia and ocular biometry in a UK University student population . Optom Vis Sci 2005; 82: 261–266.
- Gibson GA. Theoretical and empirical evaluation of phakic and pseudophakic accommodation. PhD thesis, Aston University, 2008. pp 116–149.
- Ebneter A, Wagels B, Zinkernagel MS. Non‐invasive biometric assessment of ocular rigidity in glaucoma patients and controls. Eye 2009; 23: 606–611.
- Graebel WP, van Alphen GWHM. The elasticity of sclera and choroid of the human eye and its implications on scleral rigidity and accommodation. J Biomech Eng 1977; 99: 203–208.
- Glasser A. Restoration of accommodation: surgical options for correction of presbyopia. Clin Exp Optom 2008: 91: 279–295.
- Jablonski MM, Iannaccone A, Reynolds DH et al. Age‐related decline in VIP‐Positive parasympathetic nerve fibers in the human submacular choroid. Invest Ophthalmol Vis Sci 2007; 48: 479–485.
- Lam AKC, Chan ST, Chan H et al. The effect of age on ocular blood supply determined by pulsatile ocular blood flow and color Doppler ultrasonography. Optom Vis Sci 2003; 80: 305–311.
- Read SA, Collins MJ, Alonso‐caneiro D. Validation of optical low coherence reflectometry retinal and choroidal biometry. Optom Vis Sci 2011; 88: 855–863.
- Logan NS, Gilmartin B, Wildsoet CF et al. Posterior retinal contour in adult human anisomyopia . Invest Ophthalmol Vis Sci 2004; 45: 2152–2162.
- Gilmartin B, Nagra M, Logan NS. Shape of the posterior vitreous chamber in human emmetropia and myopia. Invest Ophthalmol Vis Sci 2013; 54: 7240–7251.
- Blank K, Enoch JM. Monocular spatial distortions induced by marked accommodation. Science 1973; 182: 393–395.
- Blank K, Provine RR, Enoch JM. Shift in the peak of the photopic Stiles‐Crawford function with marked accommodation. Vision Res 1975; 15: 499–507.
- Enoch JM. Marked accommodation, retinal stretch, monocular space perception and retinal receptor orientation. Am J Optom Physiol Opt 1975; 52: 376–392.